Chemistry of Engineering Materials Engineering Chemistry PDF
Document Details
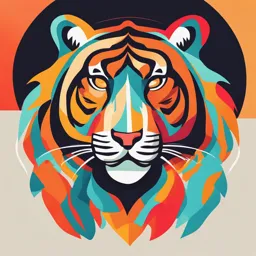
Uploaded by ConscientiousEuphemism
Pandit Deendayal Energy University
Tags
Summary
This document provides an introduction to the topic of engineering materials within the field of chemistry. It covers traditional materials, advanced materials (including nanomaterials), and applications in various engineering disciplines.
Full Transcript
Chemistry of Engineering Materials UNIT 1: Chemistry of Engineering Materials 10 h Traditional Materials: Introduction and classification of materials; metallic materials, polymeric, ceramic materials Advanced Materials: Introduction to nanomaterials: Prope...
Chemistry of Engineering Materials UNIT 1: Chemistry of Engineering Materials 10 h Traditional Materials: Introduction and classification of materials; metallic materials, polymeric, ceramic materials Advanced Materials: Introduction to nanomaterials: Properties and application; Carbonaceous materials (fullerene, carbon nanotube, graphene, etc.); Composite materials; Liquid crystals: Classification and Application Reference book: Wiley Engineering Chemistry Materials Science and Engineering-An Introduction; Callister Lectures Unit Name & Details UNIT 1: Chemistry of Engineering Materials 1 Introduction to Chemistry of Engineering Materials: 2 Classification of materials: 3 Traditional Materials: Traditional ferrous metals: examples, properties and applications; Traditional ceramic materials: examples, properties and applications ; Traditional polymeric materials: (addition/ condensation polymer; Thermoplastic/ thermosetting) 4 Advanced materials: Nano materials; Carbonaceous materials; Composite materials; Liquid crystals Nanomaterials L1: What is nano-materials Surface area to volume ratio and influence in sensing, catalytic, absorption applications 5 Nanomaterials L2: Quantum confinement and influence in bandgap, electrical and optical properties 6 Top down and bottom up approaches for synthesis of materials Sol-Gel, Chemical vapour deposition, Ball milling 7 Carbonaceous materials L1 (fullerene, graphene) Properties & Applications 8 Carbonaceous materials L2 Carbon nanotube Properties & Applications Composite materials L1 9 Composite materials L2 Liquid crystals L1 10 Liquid crystals L2 Chemical Engineering Natural Process Mechanical Engineering Civil Engineering Chemistry Materials Electrical Engineering Computer Engineering Synthetic Process Electronic Engineering Chemistry for Engineering Disciplines: Few Examples Chemical Engineer: Do we need to know Chemistry !! Mechanical Engineer: Do we need to know Chemistry !! Civil Engineer: Is material important for us !! Non renewable energy Fuel Cell Battery Piezoelectric Supercapacitor Advanced PV technologies Electrical Engineer: Should we be specific only to metallic conductor… Do we need to know materials!! Computer Vision Machine Learning Artificial Intelligence Internet of Things Cyber Physical Systems Computer Engineer: Do we need to know chemistry / materials!! The basic function of computers and communications systems is to process and transmit information in the form of signals representing data, speech, sound, documents, and visual images. These signals are created, transmitted, and processed as moving electrons or photons, and so the basic materials groups involved are classified as electronic and photonic. In some cases, materials known as optoelectronic bridge these two classes, combining abilities to interact usefully with both electrons and photons. Among the electronic materials are various crystalline semiconductors; metalized film conductors; dielectric films; solders; ceramics and polymers formed into substrates on which circuits are assembled or printed; and gold or copper wiring and cabling. Photonic materials include a number of compound semiconductors designed for light emission or detection; elemental dopants that serve as photonic performance-control agents; metal- or diamond-film heat sinks; metalized films for contacts, physical barriers, and bonding; and silica glass, ceramics, and rare earths for optical fibres. Materials Science and Engineering Materials science involves investigating the relationships that exist between the structures and properties of materials (i.e., why materials have their properties). In contrast, materials engineering involves, on the basis of these structure– property correlations, designing or engineering the structure of a material to produce a predetermined set of properties. From a functional perspective, the role of a materials scientist is to develop or synthesize new materials. A materials engineer is called upon to create new products or systems using existing materials and/or to develop techniques for processing materials. Classification of Materials World of human being !! World of Materials !! Composition wise Metal Ceramic Polymer Carbonaceous Composite Materials Science and Engineering, William D. Callister Structural features and property wise According to structural features, materials can also be classified Crystalline Porous Amorphous Non-porous According to property, materials can be classified as follows Electrical property Thermal property Mechanical property Conductor Conductor Brittle Semiconductor Insulator Ductile Insulator Opical property Transparent Translucent Opaque Length scale wise Nano materials and Bulk materials Nanomaterials are invisible to the naked eye. But the bulk materials, we can see their particles. The difference between nanomaterials and bulk materials is that nanomaterials have their size in 1-100 nm range at least in one dimension whereas bulk materials have their size above 100 nm in all dimensions. Application / use wise Traditional and Advanced Materials Traditional Ferrous metals Ceramic materials Polymeric materials Advanced Nano materials Carbonaceous materials Composite materials Liquid crystals Metallic Materials In periodic table out of 118 elements, 90 are metals only. 10/12/2022 Dr. Anu Manhas 17 Metallic Materials Metallic materials are normally combination of one or more metallic elements and may also contain some non-metallic elements like C, N, O, etc. Metals have a crystalline structure in which the atoms are arranged in an orderly manner. They are quite strong, yet deformable, which accounts for their extensive use in structural applications. Metals are commonly divided into two classes namely, i) Ferrous Metals and ii) Non- Ferrous Metals. Metals account for about two thirds of all the elements and about 24% of the mass of the planet. Metals have useful properties including strength, ductility, high melting points, thermal and electrical conductivity, and toughness. 10/12/2022 Dr. Anu Manhas 18 Metallic Materials Ferrous Metals: Ferrous metals are rich in iron. Iron such as cast iron, wrought iron, steel is the main constituents in ferrous metals. Ferrous metals are magnetic and capable of little resistance to the corrosion too. Examples for ferrous metals are cast iron, carbon steels, alloy steels, stainless steels, tool steels and die steels. Non-Ferrous Metals: These metals have lightweight, high conductivity, corrosion resistance and non-magnetic properties are the specialties of non-ferrous metals. These metals don’t have the iron as the composition. Some amount of iron will be added in some of the Non-ferrous metals but it is not a considerable amount. Example: aluminium, copper, lead, nickel, tin, titanium and zinc. Some of the Non-ferrous alloys such as brass, gold, silver and platinum. cobalt, mercury, lithium, tungsten, indium, beryllium, cadmium, niobium, tellurium, gallium, germanium, selenium, tantalum, vanadium, and zirconium are some of the rare non-ferrous metals. 10/12/2022 Dr. Anu Manhas 19 Metallic Materials Common Metallic Materials Iron/Steel - Steel alloys are used for strength critical applications. Aluminum - Aluminum and its alloys are used because they are easy to form, readily available, inexpensive, and recyclable. Copper - Copper and copper alloys have a number of properties that make them useful, including high electrical and thermal conductivity, high ductility, and good corrosion resistance. Titanium - Titanium alloys are used for strength in higher temperature (~1000° F) application, when component weight is a concern, or when good corrosion resistance is required. Nickel - Nickel alloys are used for still higher temperatures (~1500-2000° F) applications or when good corrosion resistance is required. Refractory materials are used for the highest temperature (> 2000° F) applications. 10/12/2022 Dr. Anu Manhas 20 Metallic Materials The key feature that distinguishes metals from non-metals is their bonding. Metallic materials have free electrons that are free to move easily from one atom to the next. The existence of these free electrons has a number of profound consequences for the properties of metallic materials. For example, metallic materials tend to be good electrical conductors because the free electrons can move around within the metal so freely. 10/12/2022 Dr. Anu Manhas 21 Know the polymers surrounding you !! What are polymers !! What is degree of polymerization !! Polymers are (Greek poly-many, mers-units or parts) macromolecules built up by the linking together of a large number of small molecules, called monomers. Large molecules are build up by repetition of simple chemical unit. The repeat units are linear or branched. Degree of polymerization (DP) is the number of repeating units in chain formed in polymer. The molecular weight of the polymer is the product of the molecular weight of the repeat unit and the DP. Monomer Polymer CH3 H3C Polyethylene n Ethylene Repeat unit CH3 Ph n Polystyrene Ph Ph Ph Ph Ph Ph Ph Styrene CH3 CH3 n Polypropylene CH3 CH3 CH3 CH3 CH3 CH3 CH3 Propylene Nomenclature Homopolymers and copolymers : A polymer may consist of identical monomers or different chemical structure and accordingly they are called homopolymers and copolymers. …..-M-M-M-M-M-M-M-…… ……-M1-M2-M1-M2-M1-M2-M1-…… Homopolymer Copolymer Copolymers are of different types two or more monomers polymerized together random random – A and B randomly vary in chain alternating – A and B alternate in alternating polymer chain block – large blocks of A alternate block with large blocks of B graft – chains of B grafted on to A backbone graft A– B– Tacticity: The orientation of monomeric units in a polymer molecule can take place in an orderly or disorderly fashion with respect to the main chain. The difference in configuration (tacticity) do affect their physical properties. (i) In this configuration the functional groups are all on the same side of the chain, is called isotactic polymer. Example polypropylene made by Ziegler-Natta catalyst H H H H H H H H C C C C C C C C H R H R H R H R (ii) If the arrangement of functional groups are at random around the main chain, it is called atactic polymer, for example polyvinylchloride H H H H H R H H C C C C C C C C H R H R H H H R (iii) If the arrangement of side groups is in alternating fashion, it is called syndiotactic polymer, for example gutta percha H H H R H H H R C C C C C C C C H R H H H R H H Functionality of monomer The number of bonding sites in a monomer is referred to as it’s functionality Mono-functional e.g. CH3OH, C2H5OH, C6H5NH2 Bi-functional e.g. CH2=CH2, CCl2=CCl2, HOCH2CH2OH, C6H5CH=CH2 Multifunctional: Si(OH)4 Importance of functionality: Bifunctional monomer alone can form linear homopolymer Trifunctional polymer can yield branched or cross linked polymer Mixture of bifunctional and trifunctional monomers produces branched or cross-linked Mixture of two bifunctional monomers yield branched or graft polymer. Types of polymerization There are mainly three types of polymerization reaction, (a) Addition or chain polymerization (b) Condensation or step-polymerization (c) Copolymerization (a) Addition or chain polymerization is a reaction that yield a product, which is exact multiple of the original monomeric molecule. Chain-growth polymers are prepared by chain reactions. Monomers are added to the growing end of a polymer chain. The conversion of vinyl chloride to poly(vinyl chloride) is an example. The addition polymerization reaction is initiated by application of heat, light, pressure or a catalyst for breaking down the double covalent bonds of monomers. Mechanism of Addition polymerization Initiation peroxide Rad Rad + CH2=CH Rad-CH 2-CH G G Chain propagation CH2=CHG Rad-CH 2-CH + CH2=CH Rad-CH 2-CH-CH 2-CH Rad-CH 2-CH-CH 2-CH-CH 2-CH G G G G G G G Chain termination. 2 Rad(CH 2-CH) n-CH 2-CH G G Rad(CH 2CH) nCH2CHCHCH 2(CH-CH 2)nRad G G G G Types of polymerization (b) Condensation or step polymerization may be defined as a reaction when monomers containing two functional groups come together and lose a small molecule such as H2O or HCl. In this method, any two reactive molecules can combine, so that monomer is not necessarily added to the end of a growing chain. Step-growth polymerization is used to prepare polyamides and polyesters. Amide linkage Diamine Adipoyl chloride Polyamide Differences between Addition and Condensation polymerization Addition polymerization Condensation polymerization 1. Only growth reaction adds Any two molecular species repeating units one at a time present can react to the chain 2. Number of units increases Monomers disappear early in steadily throughout the the reaction reaction 3. Longer reaction times have To obtain high molecular a little effect on molecular weight, longer reaction time weight, but gives higher yields is essential 4. Classification on the basis of Thermal response: Based on the behaviour of the polymer when heated to processing temperature, polymeric material may be classified as (i) Thermoplastic and (ii) Thermosetting polymers (i) Thermoplastic polymers are linear, long chain polymers, which can be softened on heating and hardened on cooling reversibly, their hardness is temporary property, subject to change with rise and fall of temperature. Examples: polythene, polypropylene, polystyrene etc. (ii) Thermosetting polymers or thermosets are those polymers, which during moulding get hardened and once they have solidified, they can not be softened. They are permanent setting polymers. Such polymers during heating acquire three- dimensional cross-linked structure, which predominately strong covalent bonds. Examples: Polyester, bakelite, epoxy-resin etc. Differences between Thermoplastic and Thermosetting polymers Thermoplastic polymers Thermosetting polymers 1. They soften on heating readily They do not soften on heating. On prolong heating however, they burn. 2. They consist of long-chain Their set molecules have three- linear macromolecules dimensional net work structure, joined by strong covalent bonds 3. They are mostly formed by They are formed by addition polymerization process condensation polymerization process 4. By reheating to a suitable They retain their shape and temperature, they can be structure, even on heating. softened, reshaped and thus Hence they can not be reshaped reused and reused 5. They are usually soft, weak They are usually hard, strong 6. They can be reclaimed from They can not be reclaimed from wastes wastes 7. They are usually soluble in Due to strong bond and cross- some organic solvent linking, they are insoluble in almost all organic solvents Molecular weight and degree of polymerisation Number of repeating unit in a polymer called as degree of polymerisation (DP). DP provides the indirect method of expressing the molecular weight and the relation is as follows; M = DP x m Where, M is the molecular weight of polymer, DP is the degree of polymerisation and m is the molecular weight of the monomer ni (DP)i ni (DP)i 2 (DP)n = and( DP ) w = n i n (DP) i i Each of these averages can be related to the corresponding molecular weight average by the following two equations; Mn = (DP)n.m Mw = (DP)w.m Number average molecular weight and weight average molecular weight The number average molecular weight is the total weight of the sample divided by the number of molecules in the sample. Number-average molecular weight Mn= niMi = wi ni wi/Mi Weight-average molecular weight w= Mw== niMi 2 Mw== wiMi niMi 2 niMi wiMi 2 = wiMi niMi niMi wi niMi wi wi For synthetic polymers Mw is greater than the Mn. If they are equal than they will consider as perfectly homogeneous. (Each molecule has same molecular weight). The dispersity index, or formerly polydispersity index (PDI), or heterogeneity index, or simply dispersity (Đ), is a measure of the distribution of molecular mass in a given polymer sample. Đ (PDI) of a polymer is calculated: PDI=Mw/Mn Determine the mole fraction of vinyl chloride and vinylacetate in a copolymer having a molecular weight of 10520 g/mol and a degree of polymerization of 160. Ans: 0.86: 0.14 If a vinyl chloride-vinyl acetate copolymers has a ratio of 10:1 vinyl chloride to vinyl acetate and a molecular weight of 16000 g/ mol. What is the degree of polymerization !! Ans: 248 Vinyl chloride: CH2=CHCl; Vinyl acetate: CH2=CHCOCH3 In a polymer given that M1=1000 g/mol, N1=100; M2=2000g/mol, N2=200; M3=5000 g/mol, N3=500. Calculate the no average (Mn) and weight average (Mw) molecular weight. From the given polymer-data calculate the following: (i) number average molecular weight; (ii) weight-average molecular weight. Mol. weight No. Weight range (g/mol) fraction fraction 8000-12000 0.10 0.05 12000-16000 0.30 0.25 16000-20000 0.40 0.35 20000-24000 0.20 0.35 Glass-ceramic Ceramics are compounds between metallic and nonmetallic elements; they are most frequently oxides, nitrides, and carbides. For example, common ceramic materials include aluminum oxide (or alumina, Al2O3), silicon dioxide (or silica, SiO2), silicon carbide (SiC), silicon nitride (Si3N4), and, in addition, what some refer to as the traditional ceramics—those composed of clay minerals (e.g., porcelain), as well as cement and glass. Ceramic materials are often describe that they are inorganic and nonmetallic materials. Most ceramics are compounds between metallic and nonmetallic elements for which the interatomic bonds are either totally ionic, or predominantly ionic but having some covalent character. The term ceramic comes from the Greek word keramikos, which means “burnt stuff,” indicating that desirable properties of these materials are normally achieved through a high-temperature heat treatment process called firing. With regard to mechanical behavior, ceramic materials are relatively stiff and strong—stiffnesses and strengths are comparable to those of the metals. In addition, they are typically very hard. Historically, ceramics have exhibited extreme brittleness (lack of ductility) and are highly susceptible to fracture. However, newer ceramics are being engineered to have improved resistance to fracture; these materials are used for cookware, cutlery, and even automobile engine parts. Furthermore, ceramic materials are typically insulative to the Ceramic Structures Because ceramics are composed of at least two elements, and often more, their crystal structures are generally more complex than those for metals. The atomic bonding in these materials ranges from purely ionic to totally covalent; many ceramics exhibit a combination of these two bonding types, the degree of ionic character being dependent on the electronegativities of the atoms. Table 1 presents the percent ionic character for several common ceramic materials; CERAMICS BONDING Bonding: Table 1 Percent Ionic Mostly ionic, some covalent. Character of the % ionic character increases with difference in Interatomic Bonds for electronegativity. Several Ceramic Materials SILICATE CERAMICS Silicates are materials composed primarily of silicon and oxygen, the two most abundant elements in Earth’s crust; consequently, the bulk of soils, rocks, clays, and sand come under the silicate classification. Rather than characterizing the crystal structures of these materials in terms of unit cells, it is more convenient to use various arrangements of an SiO44- tetrahedron (Figure 1). Each atom of silicon is bonded to four oxygen atoms, which are situated at the corners of the tetrahedron; the silicon atom is positioned at the center. Because this is the basic unit of the silicates, it is often treated as a negatively charged entity. Figure 1 A silicon–oxygen 42 (SiO44- )tetrahedron. Often the silicates are not considered to be ionic because there is a significant covalent character to the interatomic Si–O bonds, which are directional and relatively strong. Regardless of the character of the Si–O bond, there is a formal charge of −4 associated with every SiO4−4 tetrahedron because each of the four oxygen atoms requires an extra electron to achieve a stable electronic structure. Various silicate structures arise from the different ways in which the SiO4−4 units can be combined into one-, two-, and three-dimensional arrangements. Dr. Syed Shahabuddin 43 The Silicates For the various silicate minerals, one, two, or three of the corner oxygen atoms of the 𝑆𝑖𝑂 tetrahedra are shared by other tetrahedra to form some rather complex structures. Some of these, represented in Figure 2, have formulas 𝑆𝑖𝑂 , 𝑆𝑖2𝑂 , 𝑆𝑖3𝑂 and so on; single- chain structures are also possible. Positively charged cations such as Ca2+, Mg2+, and Al3+ serve two roles: First, they compensate the negative charges from the 𝑆𝑖𝑂 units so that charge neutrality is achieved; second, these cations ionically bond the 𝑆𝑖𝑂 tetrahedra together. Figure 2 Five silicate ion structures formed from 𝑆𝑖𝑂 tetrahedra. Dr. Syed Shahabuddin 44 Simple Silicates Of these silicates, the most structurally simple ones involve isolated tetrahedral (Figure 2a). For example, forsterite (Mg2SiO4) has the equivalent of two Mg2+ ions associated with each tetrahedron in such a way that every Mg2+ ion has six oxygen nearest neighbours. The 𝑆𝑖2𝑂 ion is formed when two tetrahedra share a common oxygen atom (Figure 2b). Akermanite (Ca2MgSi2O7) is a mineral having the equivalent of two Ca2+ ions and one Mg2+ ion bonded to each 𝑆𝑖2𝑂 unit. Dr. Syed Shahabuddin 45 Layered Silicates A two-dimensional sheet or layered structure can also be produced by the sharing of three oxygen ions in each of the tetrahedra (Figure 3); for this structure, the repeating unit formula may be represented by (Si2O5)2−. The net negative charge is associated with the unbonded oxygen atoms projecting out of the plane of the page. Electroneutrality is ordinarily established by a second planar sheet structure having an excess of cations, which bond to these unbonded oxygen atoms from the Si2O5 sheet. Such materials are called the sheet or layered silicates, and their basic structure is characteristic of the clays and other minerals. One of the most common clay minerals, kaolinite, has a relatively simple two-layer silicate sheet structure. Kaolinite clay has the formula Al2(Si2O5)(OH)4 in which the silica tetrahedral layer, represented by Figure 3 Schematic representation of (Si2O5)2−, is made electrically neutral by an adjacent the two-dimensional silicate sheet Al2(OH)2+4 layer. structure having a repeat unit formula of (Si2O5)2-. Dr. Syed Shahabuddin 46 GLASS Dr. Syed Shahabuddin 47 Dr. Syed Shahabuddin 48 Dr. Syed Shahabuddin 49 Dr. Syed Shahabuddin 50 Dr. Syed Shahabuddin 51 Dr. Syed Shahabuddin 52 Dr. Syed Shahabuddin 53 Dr. Syed Shahabuddin 54 Dr. Syed Shahabuddin 55 Advanced Materials: One can expect ‘Improvement’ Advanced Nano materials Carbonaceous materials Composite materials Liquid crystals History of ‘Nano’ Roman made Lycurgus cup in the 4th Century AD Reflected light Transmitted light Faraday’s gold (1856) ‘Divided state of gold’ Nanomaterials: Introduction and Examples Imagine ‘nano’ size http://www.essentialchemicalindustry.org/materials-and-applications/nanomaterials.html Nanotechnology: The study of the controlling of matter on an atomic and molecular scale. Generally nanotechnology deals with structures sized between 1 to 100 nanometer in at least one dimension, and involves developing or modifying materials or devices within that size. What do you mean by Nano Particles ? Nano Particles are the particles of size between 1 nm to 100 nm 1 nm is only three to five atoms wide. ~40,000 times smaller than the width of an average human hair Nanometer - One billionth (10-9) of a meter The size of Hydrogen atom 0.04 nm The size of Proteins ~ 1-20 nm Feature size of computer chips 180 nm Diameter of human hair ~ 10 µm At the nanoscale, the physical, chemical, and biological properties of materials differ in fundamental and valuable ways from the properties of individual atoms and molecules or bulk matter Can small things make a big difference! Your idea! What are the decisive factors! How nanomaterials are advanced materials 1. Surface to Volume ratio (chemical reactivity, surface area increases) 2. Quantum confinement (with the decrease in size bandgap increases) A cube having volume of 125000cm3 is divided equally in 8 cubes. Compare the surface to volume ratios between the initial cube and the total nos of fragmented cubes. Quantum confinement Quantum confinement In a small nanocrystals, the electronic energy level are not continuous as in the bulk but are discrete (finite density of states), because of the confinement of the electronic wave function to the physical dimensions of the particles. This phenomenon is called quantum confinement Therefore nanocrystals are also referred to as quantum dots (QDs) In any material substantial variation of fundamental electrical and optical properties with reduced size will be observed when the energy spacing between the electronic levels exceeds the thermal energy (kT) Optical properties metal nanoparticles are enlarged, CdSe semiconductor nanoparticles, their optical properties change a simple change in size alters the only slightly as observed for the optical properties of the different samples of gold Semiconductor nanospheres Metal Fluorescence emission of (CdSe) ZnS absorption spectra of various sizes and quantum dots of various sizes shapes of gold nanoparticles Preparation of Nanomaterials Top down (breaking/ dissociation) Bottom up (growth/ construction) Physical or Chemical !! What is the aim !! Nanoparticles, Nano-structured materials, thin films, 2D materials Sol-gel process The reactions involved in sol-gel chemistry based on the hydrolysis and condensation of metal alkoxides MOR: MOR + H2O MOH +ROH (Hydrolysis) MOH + ROM M-O-M + ROH (Condensation) The process involves the evolution of inorganic networks through the formation of a colloidal suspension (Sol) and gelation of the sol to form a network in a continuous liquid phase (gel). The starting material is processed to form a dispersible oxide and forms a sol in contact with water or dilute acid. Removal of the liquid from the sol yields the gel, and the sol/gel transition controls the particle size and the shape. Calcination of the gel produces the oxide. Schematic representation of sol-gel process of synthesis of nanomaterials Steps of sol-gel process Formation of different stable solutions of the alkoxide or solvated metal precursor Formation of oxide or alcohol-bridged network (the gel) by a polyconensation or polyesterification reaction that causes tremendous increase in the viscosity of the solution. Aging of the gel during which the polycondnsation reactions continue until the gel transform into a solid mass. This is accompanied by contraction of the gel network and expansion of solvent from gel pores. Drying the gel, when water and other volatile liquid are removed from the gel network. This process involves fundamental change in the structure of the gel. Dehydration, during which surface bound M-OH groups are removed, thereby stabilising the gel againest rehydration. This is achieved by heating at temperature up to 800 ºC. Densification and decomposition of the gels at high temperature. The pores of the gel network are collapsed, the remaining organic species are volatilized. Advantages and disadvantages of sol-gel process The advantage is possibility of synthesizing nanomatallic inorganic materials like glasses, glass ceramics or ceramic materials at very low temperatures compared to high temperature process required by melting glass or firing ceramics. One of the main advantages is to synthesize monosized nano particles by any bottom up approach. The major difficulty is controlling the growth of the particles and then stopping the newly formed particles from agglomerating. Other technical issues are ensuring the reactions are complete so that no unwanted reactant is left on the product and completely removing any growth aids that may have been used in the process. Production rate of nano powders are very low. Mechanical Grinding (Physical Method) Mechanical milling is achieved using high energy shaker, planetary ball, or tumbler mills Energy transferred to the powder from refractory depends on the Rotational speed, size and number of the balls Ratio of the ball to powder mass The time of milling Milling atmosphere Nanoparticle are produced by the shear action during grinding. Chemical Vapour Deposition What is CVD Buckminsterfullerene/ Buckyballs from sciencedaily.com from unusualife.com Molecule consisting of 60 C atoms sp2 hybridized bonds Buckminster Fuller Has 20 hexagons, 12 pentagons (American architect) Less than 1 nm in diameter Designed geodesic domes Useful as drug carrier Compressed – becomes stronger than diamond Related structures have 70 or 84 C atoms 1996 Nobel Prize in Chemistry from Nobelprize.org Graphene (single sp2 bonded carbon sheet) from cnx.org from Nobelprize.org In proportion to its thickness, it is stronger than the steel. It conducts heat and electricity very efficiently and is nearly transparent Nobel Prize in Physics 2010 Carbon nanotubes Rolled up sheet of sp2 bonded carbon atoms Rolled up sheet of graphene from informaworld.com Discovered by Japanese Scientist Sumio Iijima in 1991 Single Wall Carbon nanotube (SWNT) or Multi Wall Carbon nanotube (MWNT) Interlayer separation ~ 3.4 Angstrom Multiwalled Carbon Nanotube Parchment Russian Doll Classification of CNT: Chirality CNT Chiral Achiral No mirror plane Mirror plane Types of Carbon Nanotubes Single-walled nano tubes (SWNT): Multi-walled nano tubes (MWNT): (n, m) notation of CNT What does it mean (4, 2) CNT; (4, 0) CNT; (5, 9) CNT Chiral vector of the tube C(n, m) Chiral Angle θ (n, n) gives an armchair tube (n, 0) gives a zigzag tube (n, m) gives a chiral tube If (n-m) is divisible by 3, tube is metallic otherwise it is semiconducting How are carbon nanotubes made? Three main methods are currently available for the production of CNTs: arc discharge, laser ablation of graphite, and chemical vapor deposition (CVD). In the first two processes, graphite is combusted electrically or by means of a laser, and the CNTs developing in the gaseous phase are separated. All three methods require the use of metals (e.g. iron, cobalt, nickel) as catalysts. CVD process: The CVD process currently holds the greatest promise, since it allows the production of larger quantities of CNTs under more easily controllable conditions and at lower cost. In the CVD process, manufacturers can combine a metal catalyst (such as iron) with carbon-containing reaction gases (such as hydrogen or carbon monoxide) to form carbon nanotubes on the catalyst inside a high-temperature furnace. 10/12/2022 Dr. Megha Balha 97 Carbon Nanotubes The CVD process can be purely catalytic or plasma-supported. The latter requires slightly lower temperatures (200-500°C) than the catalytic process (up to 750°C) and aims at producing 'lawn-like' CNT growth. Purification: Even though synthetic techniques have been improved to obtain high-purity carbon nanotubes, the formation of byproducts containing impurities such as metal encapsulated nanoparticles, metal particles in the tip of a carbon nanotube, and amorphous carbon has been an unavoidable phenomenon, because the metal nanoparticles are essential for the nanotube growth. These foreign nanoparticles, as well as structural defects that occurred during synthesis, have the unfortunate implication that they modify the physico-chemical properties of the produced carbon nanotubes. That's why carbon nanotubes need to be purified with the help of various methods such as acid treatment or ultrasound at the end of the production process. 10/12/2022 Dr. Megha Balha 98 Carbon nanotube uses and applications CNTs are well-suited for virtually any application requiring high strength, durability, electrical conductivity, thermal conductivity and lightweight properties compared to conventional materials. Currently, CNTs are mainly used as additives to synthetics. CNTs are commercially available as a powder, i.e. in a highly tangled-up and agglomerated form. For CNTs to unfold their particular properties they need to be untangled and spread evenly in the substrate. Another requirement is that CNTs need to be chemically bonded with the substrate, e.g. a plastic material. For that purpose, CNTs are functionalized, i.e. their surface is chemically adapted for optimal incorporation into different materials and for the specific application in question. Carbon nanotubes can also be spun into fibers, which not only promise interesting possibilities for specialty textiles but may also help realize a particularly utopian project – the space elevator. 10/12/2022 Dr. Megha Balha 99 Carbon nanotube uses and applications Materials Carbon nanotube enabled nanocomposites have received much attention as a highly attractive alternative to conventional composite materials due to their mechanical, electrical, thermal, barrier and chemical properties such as electrical conductivity, increased tensile strength, improved heat deflection temperature, or flame retardancy. These materials promise to offer increased wear resistance and breaking strength, antistatic properties as well as weight reduction. For instance, it has been estimated that advanced CNT composites could reduce the weight of aircraft and spacecraft by up to 30%. Catalysis What makes carbon nanotubes so attractive for catalysis is their exceptionally high surface area combined with the ability to attach essentially any chemical species to their sidewalls. Already, CNTs have been used as catalysts in many relevant chemical processes, however, controlling their catalytic activity is not easy. 10/12/2022 100 Dr. Megha Balha Carbon nanotube uses and applications Transistors Despite the rise of graphene and other two-dimensional (2D) materials, semiconducting single-walled carbon nanotubes are still regarded as strong candidates for the next generation of high-performance, ultra-scaled and thin-film transistors as well as for opto-electronic devices to replace silicon electronics. Electrodes Carbon nanotubes have been widely used as electrodes for chemical and biological sensing applications and many other electrochemical studies. With their unique one-dimensional molecular geometry of a large surface area coupled with their excellent electrical properties, CNTs have become important materials for the molecular engineering of electrode surfaces where the development of electrochemical devices with region-specific electron-transfer capabilities is of paramount importance 10/12/2022 101 Dr. Megha Balha Carbon nanotube uses and applications Optoelectronic and photonic applications While individual nanotubes generate discrete fine peaks in optical absorption and emission, macroscopic structures consisting of many CNTs gathered together also demonstrate interesting optical behavior. For example, a millimeter-long bundle of aligned MWCNTs emits polarized incandescent light by electrical current heating and SWCNT bundles are giving higher brightness emission at lower voltage compared with conventional tungsten filaments. Displays Given their high electrical conductivity, and the incredible sharpness of their tip (the smaller the tips' radius of curvature, the more concentrated the electric field, the higher field emission), carbon nanotubes are considered the most promising material for field emitters and a practical example are CNTs as electron emitters for field emission displays (FED). 10/12/2022 102 Dr. Megha Balha Carbon nanotube uses and applications Displays Field emission display (FED) technology makes possible a new class of large area, high resolution, low cost flat panel displays. However, FED manufacturing requires CNT to be grown in precise sizes and densities. Height, diameter and tip sharpness affect voltage, while density affects current. Nanomedicine and biotechnology Carbon nanomaterials such as nanotubes or graphene not only are widely researched for their potential uses in industrial applications, they also are of great interest to biomedical engineers working on nanotechnology applications. Nitrogen-doped carbon nanotubes for instance have been developed for drug delivery applications 10/12/2022 103 Dr. Megha Balha Carbon nanotube uses and applications Sensors The group of Cees Dekker paved the way for the development of CNT-based electrochemical nanosensors by demonstrating the possibilities of SWCNTs as quantum wires and their effectiveness in the development of field-effect transistors. Many studies have shown that although CNTs are robust and inert structures, their electrical properties are extremely sensitive to the effects of charge transfer and chemical doping by various molecules. Most sensors based on CNTs are field effect transistors (FET) – although CNT are robust and inert structures, their electrical properties are extremely sensitive to the effects of charge transfer and chemical doping by various molecules. CNTs-FETs have been widely used to detect gases such as greenhouse gases in environmental applications 10/12/2022 104 Dr. Megha Balha