Chemistry in Biology #2 Review Outline- Alisa PDF
Document Details
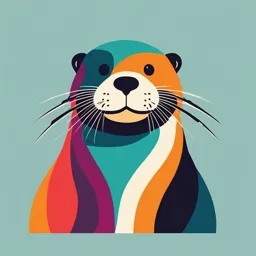
Uploaded by ProsperousBaltimore6630
Alisa Liashkova
Tags
Summary
This review outline covers organic chemistry concepts, focusing on carbohydrates and lipids. It details their structure, function, and roles in biological systems. The document emphasizes the importance of carbon and its versatility in forming the backbone of many biological molecules.
Full Transcript
Chemistry in Biology #2 Review Outline Made by Alisa Liashkova Organic Chemistry - Organic molecules - what are they? Organic molecules are compounds primarily composed of carbon (C) and hydrogen (H) atoms,...
Chemistry in Biology #2 Review Outline Made by Alisa Liashkova Organic Chemistry - Organic molecules - what are they? Organic molecules are compounds primarily composed of carbon (C) and hydrogen (H) atoms, often incorporating other elements such as oxygen (O), nitrogen (N), sulfur (S), and phosphorus (P). A fundamental characteristic of organic molecules is the presence of carbonhydrogen (C-H) bonds, where carbon atoms are covalently bonded to hydrogen atoms. These molecules form the backbone of life, serving as the building blocks for more complex structures in living organisms. Organic molecules can exist as small molecules like methane or as large polymers like DNA and proteins. Also, it’s… 1. A molecule that is normally found in or produced by living systems. 2. A molecule that typically consists of carbon atoms in rings or long chains, where other atoms (e.g. hydrogen, oxygen, and nitrogen) are attached. Importance of carbon: Tetravalency: Carbon atoms can form four covalent bonds, allowing for a vast diversity of stable compounds. Versatility in Structures: The ability to form single, double, and triple bonds enables carbon to create chains, branched structures, and rings, essential for building complex molecules. Stability: Carbon-based compounds are generally stable under a wide range of conditions, making them suitable for intricate biological functions. Examples of Organic Molecules: Glucose (C₆H₁₂O₆): A simple sugar used for energy in cellular respiration. Methane (CH₄): The simplest organic molecule, constituting a primary component of natural gas. DNA and RNA: Nucleic acids that store and transmit genetic information. Proteins: Polymers of amino acids that perform various structural and functional roles in organisms. Macromolecules Macromolecules are formed by joining smaller organic molecules together. Macromolecules are large, complex molecules essential to life. They are built from smaller units called monomers and include four major classes: carbohydrates, lipids, proteins, and nucleic acids. Each class plays distinct and essential roles in living organisms. - Carbohydrates Function: Carbohydrates serve as a primary energy source and provide structural support in organisms.Monomers: Monosaccharides (simple sugars) are the building blocks of carbohydrates (and are nucleotides). - Monosaccharides, Disaccharides, Polysaccharides Monosaccharides: Single sugar molecules. Examples: Glucose: Main energy source for cells. Fructose: Found in fruits; the sweetest natural sugar. Galactose: Part of lactose in milk. Disaccharides: Two monosaccharides joined together. Examples: Sucrose: Glucose + fructose (table sugar). Lactose: Glucose + galactose (milk sugar). Maltose: Glucose + glucose (product of starch digestion). Polysaccharides: Long chains of monosaccharides. Examples: Starch: Energy storage in plants. Glycogen: Energy storage in animals. Cellulose: Structural component of plant cell walls. Chitin: Structural component in fungal cell walls and insect exoskeletons. Functions Energy Storage: Polysaccharides like starch and glycogen store energy for future use. Structural Support: Cellulose provides structural integrity to plant cell walls; chitin serves a similar role in exoskeletons. Recognition and Signaling: Carbohydrates on cell surfaces play roles in cell recognition, signaling, and interactions. For example, glycoproteins are involved in immune responses. Examples and Details Glucose Metabolism: Glucose undergoes glycolysis to produce ATP, the energy currency of the cell. Dietary Fiber: Indigestible polysaccharides like cellulose contribute to digestive health by promoting regular bowel movements. - Identify carbohydrate structure Monosaccharides: Typically form ring structures in water solutions. Disaccharides: Two monosaccharide rings connected by a glycosidic bond. Polysaccharides: Long, branching chains of monosaccharides. - Lipids - Function: Lipids are hydrophobic molecules that serve multiple functions, including energy storage, insulation, protective coatings, and components of cell membranes. - Characteristics: Hydrophobic: Water-repelling, insoluble in water but soluble in nonpolar solvents. High Energy Density: Provide more energy per gram than carbohydrates or proteins due to numerous C-H bonds. - - Energy Content: Lipids provide more energy per gram than carbohydrates or proteins due to their high C-H bond content. - Biological Roles: Essential for building cell membranes, signaling pathways, and storing energy - Fats, oils, waxes Triglycerides (Fats and Oils): Structure: One glycerol molecule linked to three fatty acids. Function: Long-term energy storage; insulation; cushioning of organs. Saturated and Unsaturated Fats Types: Saturated Fats: No double bonds between carbon atoms; solid at room temperature (e.g., butter, lard). Unsaturated Fats: One or more double bonds in the fatty acid chains; liquid at room temperature (e.g., olive oil, canola oil). saturated: solid at room temperature; single C bonds; hard for body to break down unsaturated: liquid at room temperature; double C bonds - Phospholipids Structure: Glycerol backbone, two fatty acid tails, and a phosphate group. Function: Main component of cell membranes (phospholipid bilayer). Characteristics: Hydrophilic (water-attracting) head and hydrophobic (water-repelling) tails. - Sterols (Cholesterol, Estrogen and Testosterone) - Structure: Four fused carbon rings. - Function: Hormones and structural components of cell membranes. - Examples: Cholesterol: Precursor for steroid hormones; maintains membrane fluidity. Hormones: Estrogen and testosterone regulate various physiological processes - Waxes: Waxes: Function: Protective coatings; prevent water loss. Examples: Earwax, cuticle on plant leaves. - Identify lipid structure (hydrocarbon chains) Uses the molecular model kits to build the fat molecule called a triglyceride. *Notice that it contains one glycerol and three fatty acids! - Proteins Function: Proteins are involved in nearly every cellular function, including catalysis, structure, signaling, transport, and movement. Amino acids Monomers: Amino Acids are the building blocks of proteins. - each has an amino group, a carboxyl group, and a variable side group (composed of C, H, O, N, and sometimes sulfur) - 20 different amino acids: - 12 made in the body, - other 8 must consume from nuts, beans, or meat - Draw a generalized amino acid - 3D Protein structure - Four levels of Protein Structure Primary Structure: Linear sequence of amino acids. Determined by genetic information. Secondary Structure: Folding into α-helices and β-pleated sheets. Stabilized by hydrogen bonds between the backbone atoms. Tertiary Structure: Three-dimensional shape of a single protein molecule. Formed by interactions among side chains, including hydrogen bonds, ionic bonds, disulfide bridges, and hydrophobic interactions. Quaternary Structure: Assembly of multiple protein subunits into a functional complex. Examples: Hemoglobin (four subunits), DNA polymerase. - Protein function Enzymatic Activity: Enzymes like amylase catalyze biochemical reactions, increasing reaction rates without being consumed. Structural Support: Proteins like collagen provide tensile strength to connective tissues; keratin strengthens hair and nails. Transport: Hemoglobin transports oxygen in the blood; membrane proteins facilitate the transport of ions and molecules across cell membranes. Signaling: Hormones like insulin regulate physiological processes; receptor proteins transmit signals from the environment to the cell's interior. Movement: Actin and myosin are involved in muscle contraction and cellular movement. Defense: Antibodies protect against disease by identifying and neutralizing pathogens. - Three examples Different types: structural, transport, hormone, enzymes, contractile - Identify molecular protein structure How a protein is shaped controls what it can do Change the shape = stop working Denaturation: Denaturation involves the loss of a protein’s native structure due to external stressors such as heat, pH changes, or chemical agents. This results in the loss of the protein’s function. Control growth - Nucleic acids FunctionNucleic acids store and transmit genetic information; they are also involved in protein synthesis. Monomers: Nucleotides are the building blocks of nucleic acids.Nucleotide - DNA Structure DNA often is compared to a twisted ladder. Double helix formed by two antiparallel strands. Sugar-phosphate backbone on the outside. Nitrogenous bases paired on the inside. Base Pairing: Adenine pairs with Thymine (A-T) via two hydrogen bonds. Cytosine pairs with Guanine (C-G) via three hydrogen bonds. Stores genetic information for the development and functioning of living organisms. - Draw a generalized nucleotide (circle, pentagon, rectangle) with labels Drawing: Three parts in nucleotide Sugar Phosphate Nitrogenous base - Structure of the Double Helix Double helix, as related to genomics, is a term used to describe the physical structure of DNA. A DNA molecule is made up of two linked strands that wind around each other to resemble a twisted ladder in a helix-like shape. Each strand has a backbone made of alternating sugar (deoxyribose) and phosphate groups. Attached to each sugar is one of four bases: adenine (A), cytosine (C), guanine (G) or thymine (T). The two strands are connected by chemical bonds between the bases: adenine bonds with thymine, and cytosine bonds with guanine. - Bases (A,T, G,C) The hydrogen bonds between DNA strands occur between specific pairs of nitrogenous bases: T only pairs with A, and C only pairs with G. This specificity comes from the shapes of the bases and their chemical properties. Because the DNA strands are matched according to these base pairing rules, the strands are said to be complementary. - DNA Vs. RNA structures (3 structural differences) DNA consists of two strands that form a double helix structure, while RNA exists as a single strand. DNA contains deoxyribose sugar while RNA contains ribose sugar. The bases that form the lines of DNA and RNA are different by one letter, for example, DNA has (TAGC) adenine (A), thymine (T), guanine (G), and cytosine (C); while RNA has (AUGC) adenine (A), uracil (U), guanine (G), and cytosine (C). The key difference is that RNA uses (U)uracil instead of (T) thymine - DNA History - Griffith & Avery Griffith had performed the first major experiment that led to the discovery of DNA as the genetic material Observation: Harmless bacteria transformed into virulent strains when mixed with heatkilled virulent bacteria. Conclusion: Introduced the concept of a "transforming principle" responsible for heredity. Avery had: Discovery: Identified DNA as the "transforming principle" by isolating it from bacteria. Significance: Provided strong evidence that DNA is the genetic material. ▪ Identified the molecule that transformed the R strain of bacteria into the S strain ▪ Concluded that when the S cells were killed, DNA was released - R bacteria incorporated this DNA into their cells and changed into S cells. - Performed the first major experiment that led to the discovery of DNA as the genetic material (in this case it was the mice). He did this while trying to find a cure for pneumonia. The result of this experiment proved the "principle of transformation". - Hershey & Chase - Used radioactive labeling to trace the DNA and protein - Concluded that the viral DNA was injected into the cell and provided the genetic information needed to produce new viruses - Method: Used bacteriophages labeled with radioactive isotopes to trace DNA and proteins. Conclusion: Confirmed that DNA, not protein, is the genetic material transmitted to bacteria during viral infection. - Chargaff Chargaff’s rule: C = G and T = A Observation: The amount of adenine equals thymine (A = T), and cytosine equals guanine (C = G) in DNA. Implication: Suggested base pairing, crucial for DNA structure - Watson & Crick Achievement: Developed the double-helix model of DNA. Model Features: Two antiparallel strands. Complementary base pairing. Explained DNA replication mechanism - Built a model of the double helix that conformed to the others’ research 1. two outside strands consist of alternating deoxyribose and phosphate 2. cytosine and guanine bases pair to each other by three hydrogen bonds 3. thymine and adenine bases pair to each other by two hydrogen bonds - Franklin & Wilkins Franklin: First to discover DNA's double-helix shape in 1952 through her famous "Photo 51" X-ray diffraction image4 Determined that DNA could exist in two forms: A-DNA (dry form) at high humidity B-DNA (crystalline form) in wet conditions Concluded as early as November 1951 that DNA forms "a big helix in several chains, phosphates on the outside" Wilkins: - Produced the first clear X-ray images of DNA during 1948-19508 - Generated high-quality "B form" X-shaped images from squid sperm8 - Discovered that concentrated DNA solutions could form ordered threads suitable for X-ray diffraction - By November 1951, provided evidence that both cellular and purified DNA had helical structure Contribution: Used X-ray crystallography to photograph DNA fibers. Photo 51: Franklin's X-ray diffraction image revealing DNA's helical structure. - DNA Replication - Semi-Conservative Replication Semi-Conservative ReplicationSemi-conservative replication is the mechanism by which DNA is copied, ensuring that each new DNA molecule consists of one original parent strand and one newly synthesized strand. This method preserves the genetic information and maintains the integrity of the genome across generations. - Helicase, RNA Primase, DNA Polymerase, Ligase DNA helicase, an enzyme, is responsible for unwinding and unzipping the double helix. How does replication actually get going at the forks? Helicase is the first replication enzyme to load on at the origin of replication. Helicase's job is to move the replication forks forward by "unwinding" the DNA (breaking the hydrogen bonds between the nitrogenous base pairs). Proteins called single-strand binding proteins coat the separated strands of DNA near the replication fork, keeping them from coming back together into a double helix.RNA primase adds a short segment of RNA, called an RNA primer, on each DNA strand. DNA polymerase continues adding appropriate nucleotides to the chain by adding to the 3′ end of the new DNA strand.DNA polymerases are responsible for synthesizing DNA: they add nucleotides one by one to the growing DNA chain, incorporating only those that are complementary to the template. They always need a template They can only add nucleotides to the 3' end of a DNA strand They can't start making a DNA chain from scratch, but require a pre-existing chain or short stretch of nucleotides called a primer They proofread, or check their work, removing the vast majority of "wrong" nucleotides that are acidentally added to the chain DNA polymerase removes the RNA primer and fills in the place with DNA nucleotides. DNA ligase links the two sections. 1. Origins of Replication: Specific DNA sequences where replication begins. In prokaryotes like E. coli, there is a single origin called OriC. Eukaryotes have multiple origins to facilitate faster replication. Helicase: An enzyme that unwinds the double helix by breaking hydrogen bonds between complementary bases. 2. Unwinding and Stabilization Single-Strand Binding Proteins (SSBs): Bind to the separated DNA strands to prevent them from reannealing. Topoisomerase: Relieves the tension and prevents supercoiling ahead of the replication fork by creating temporary breaks in the DNA backbone. 3. Primer Synthesis RNA Primase: Synthesizes short RNA primers complementary to the DNA template strands, providing a starting point for DNA synthesis. 4. Elongation DNA Polymerase III: Extends the RNA primers by adding complementary DNA nucleotides to the 3' end, synthesizing the new DNA strand. Leading and Lagging Strands: Leading Strand: Synthesized continuously in the direction of the replication fork. Lagging Strand: Synthesized discontinuously away from the replication fork as short fragments called Okazaki fragments. 5. Primer Replacement and Ligation DNA Polymerase I: Removes RNA primers and replaces them with DNA nucleotides. Example molecules to identify