Chem 111 Ch7 - Chemical Bonding and Molecular Geometry PDF
Document Details
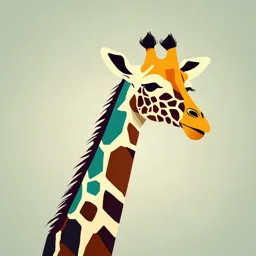
Uploaded by InterestingPerception4835
Indiana University of Pennsylvania
Tags
Summary
This document is a chapter on chemical bonding and molecular geometry, likely from a chemistry textbook or lecture notes. It covers topics such as ionic bonding, covalent bonding, electronegativity, bond types, and molecular structure. The content includes explanations, examples, and diagrams.
Full Transcript
Chapter 7 CHEMICAL BONDING AND MOLECULAR GEOMETRY Chapter Outline 7.1 Ionic Bonding 7.2 Covalent Bonding 7.3 Lewis Symbols and Structures 7.4 Formal Charges and Resonance 7.5 Strengths of Ionic and Covalent Bonds 7.6 Molecular Structure and Polarity Electronegativity Electroneg...
Chapter 7 CHEMICAL BONDING AND MOLECULAR GEOMETRY Chapter Outline 7.1 Ionic Bonding 7.2 Covalent Bonding 7.3 Lewis Symbols and Structures 7.4 Formal Charges and Resonance 7.5 Strengths of Ionic and Covalent Bonds 7.6 Molecular Structure and Polarity Electronegativity Electronegativity is a measure of the tendency of an atom to attract electrons (or electron density) towards itself. It determines how the shared electrons are distributed between the two atoms in a bond. The more strongly an atom attracts the electrons in its bonds, the larger the electronegativity. The greater the difference in electronegativity, the more polarized the electron distribution and the larger the partial charges of the atoms. In general, electronegativity increases from left to right across a period in the periodic table and decreases down a group. Electronegativity and Bond Type The absolute value of the difference in electronegativity (𝚫EN) of two bonded atoms provides a rough measure of the polarity to be expected in the bond and, thus, the bond type. When 𝚫EN is very small or zero, the bond is covalent and nonpolar. When it is large, the bond is polar covalent or ionic. The best guide, however, to a covalent or ionic character of a bond is to consider the types of atoms involved and their relative positions in the periodic table. Bonds between two nonmetals are generally covalent; bonding between a metal and a nonmetal is often ionic. As the electronegativity difference increases between two atoms, the bond becomes more ionic. Electronegativity and Bond Polarity ution polarity of these bonds increases as the absolute value of the electronegativity difference increases. The As the electronegativity difference increases between two atoms, m with the δ– designation is the more electronegative of the two. Table 7.1 shows these bonds in order of the bond becomes more polar. easing polarity. Bond Polarity and Electronegativity Difference Bond ΔEN Polarity C–H 0.4 S–H 0.4 C–N 0.5 N–H 0.9 C–O 1.0 O–H 1.4 General Bond Type High electronegativity difference (metal and non-metal): ionic bond – one or more electron transfers from metal to non-metal, cation and anion held together by ionic attraction Low average electronegativity difference (two non-metals): covalent bond – electrons shared between atoms and bonds establish. A group of atoms called a molecule Low average electronegativity difference (two metals): metallic bond – electrons shared in a completely delocalized manner, all atoms in one unified material Intermediates between these three general types are very common Ionic Compounds and Ionic Bonds s in sodium chloride Compounds (common composed table of ions aresalt) areionic called arranged to (a)(or compound maxim spheres salts).represent sodium are Ionic compounds ions, thetogether held larger ones represent by ionic bonds. chlorid can be seen more clearly. Note that each ion is “bonded” to all of t Ionic bonding results from the electrostatic attraction of oppositely charged ions that are typically produced by the transfer ofbetween electronsNa + between – c attraction and Clmetallic (cations) ions holds themandtightly togeth nonmetallic (anions) atoms. rgy to dissociate one mole of solid NaCl into separate gaseous N es of Cations Strong electrostatic attraction between Na+ and Cl- hold them n, an tightly atom oftogether a mainingroup solid NaCl. It requires element tends 769 kJ of to lose allenergy to of its valenc dissociate one mole of solid NaCl into separate Na+ and Cl- ic structure of the noble gas that precedes it in the periodic tab ions. line earth metals), the group numbers are equal to the number uently, to the charges of the cations formed from atoms of these Covalent Compounds and Covalent Bonds Covalent bonds are formed between two atoms when both have similar tendencies to attract electrons to themselves. Because the attraction between molecules, which are electrically neutral, is weaker than that between electrically charged ions, covalent compounds generally have much lower melting and boiling points than ionic compounds. Nonmetal atoms frequently form covalent bonds with other nonmetal atoms. For example, the hydrogen molecule, H2, contains a covalent bond between its two hydrogen atoms. Pure vs. Polar Covalent Bonds Covalent bonds could be pure (or nonpolar) or polar. If the atoms that form a covalent bond are identical, as in H2, Cl2, and other diatomic molecules, then the electron in the bond must be shared equally and therefore, form a pure covalent bond. Electrons shared in pure covalent bonds have an equal probability of being near each nucleus. When the atoms linked by a covalent bond are different, the bonding electrons are more attracted to the atom with higher electronegativity and therefore, creating an unequal distribution of electrons known as polar covalent bond, characterized by the partial charges on the atoms. (a) The distribution of electron density in the HCl molecule is uneven. The electron density is greater around the chlorine nucleus. The small, black dots indicate the location of the hydrogen and chlorine nuclei in the molecule. (b) Symbols δ+ and δ– indicate the polarity of the H–Cl bond. Lewis Symbols Lewis symbols are used to describe valence electron configurations of atoms and nonmetallic ions. A Lewis symbol consists of an elemental symbol surrounded by one dot for each of its valance electrons. Lewis Symbols Lewis symbols can be used to illustrate the formation of cations from atoms: Likewise, they can be used to illustrate the formation of anions from atoms: Cations are formed when atoms lose electrons, represented by fewer Lewis dots, whereas anions are formed by atoms gaining electrons. The total number of electrons does not change. The formation of ionic compounds using Lewis symbols. Lewis Structures Lewis symbols can be used to indicate the formation of covalent bonds, which are shown in Lewis structures. For example, when two chloride atoms form a chlorine molecule, they share one pair of electrons: The Lewis structure indicates that each Cl atom has three pairs of electrons that are not used in bonding (called lone pairs) and one shared pair of electrons (written between the atoms). A dash (or line) is sometimes used to indicate a shared pair of electrons: A single shared pair of electrons is called a single bond. Each Cl atom interacts with eight valence electrons: the six in the lone pairs and the two in the single bond. The Octet Rule The octet rule refers to the tendency of main group atoms to bond in such a way that each atom has eight electrons in its valence shell, giving it the same electronic configuration as a noble gas. The rule is illustrated below for carbon in CCl4 (carbon tetrachloride) and silicon in SiH4 (silane). Carbon has four valence electrons and therefore, bonded with four chlorine atoms to have eight valence electrons. Similarly, each chlorine atom has seven valence electrons and only made one single bond to have eight valence electrons each. Hydrogen only needs two electrons to fill its valence shell, so it is an exception to the octet rule. The Octet Rule Nitrogen have five valence electrons: one lone pair and three unpaired electrons. To obtain an octet, these atoms three covalent bonds, as in NH3 (ammonia). Similarly, oxygen form two covalent bonds in H2O (water) and fluorine form one covalent bond in HF (hydrogen fluoride) to obtain octet. The Octet Rule for Double and Triple Bonds A pair of atoms may need to share more than one pair of electrons in order to achieve the octet. A double bond forms when two pairs of electrons are shared between a pair of atoms, as between the carbon and oxygen atoms in CH2O (formaldehyde) and between the two carbon atoms in C2H4 (ethylene). A triple bond forms when three electron pairs are shared by a pair of atoms, as in carbon monoxide (CO) and the cyanide ion (CN-). Writing Lewis Structures with the Octet Rule 1. Determine the total number of valence (outer shell) electrons. For cations, subtract one electron for each positive charge. For anions, add one electron for each negative charge. 2. Draw a skeleton structure of the molecule or ion, arranging the atoms around a central atom (generally, the least electronegative element should be placed in the center). Connect each atom to the central atom with a single bond (one electron pair). 3. Distribute the remaining electrons as lone pairs on the terminal atoms (except hydrogen), completing an octet around each atom. 4. Place all remaining electrons on the central atom. 5. Rearrange the electrons of the outer atoms to make multiple bonds with the central atom in order to obtain octets wherever possible. 5. Rearrange the electrons of the outer atoms to make multiple bonds with the central atom in order to obtain octets wherever possible. Determine the Lewis Structure of NO Let us determine the Lewis structures of SiH , SiH, and 4 OF + as examples in following this procedure: 4 2 1. Determine the total number of valence (outer shell) electrons in the molecule or ion. Step ◦ 1: ForTotal number a molecule, ofthe we add valence numberelectrons of valence electrons on each atom in the molecule: ◦ For a negative ion, such as we add the number of valence electrons on the atoms to the number of negative charges on the ion (one electron is gained for each single negative charge): Step 2: Draw skeleton structure When several arrangements of atoms are possible, as for choose the correct one. In general, the less electronegative elemen the less electronegative carbon atom occupies the centra ◦ For a positive ion, such as NO+, we add the number of valence electrons on the atoms in the ion and then atoms surrounding it. Other examples include P in POCl , S in SO2 subtract the number of positive charges on the ion (one electron is lost for each single positive charge) 3 hydrogen from the total number of valence electrons:is almost never a central atom. As the most electronegat central atom. 3. Distribute the remaining electrons as lone pairs on the terminal at valence shells with an octet of electrons. choose the correct one. In general, the less electronegative elements are more likely to the less electronegative carbon atom occupies the central position with the o Determine the Lewis Structure of CH O atoms surrounding ◦ For a negative ion, such as it. Other 2 - we add the numberinclude examples of valencePelectrons in POCl3on , Sthe inatoms SO2, and to theCl in number of A negativehydrogen is almost charges on the ion (onenever a central electron is gained atom. for eachAs the negative single most electronegative charge): element, nuorin Step 1: Total number of valence electrons central atom. 3. Distribute the remaining electrons as lone pairs on the terminal atoms (except hydrog valence shells with an octet of electrons. When several arrangements of atoms are poss ◦ There are no remaining electrons onchoose SiH4, so the correct it is one. In general, the less ele unchanged: the less electronegative carbon atom 7.3 Lewis Symbols and atoms surrounding it. Other examples include hydrogen is almost never a central atom. As th central atom. Step 2: Draw skeleton ◦ For a structure + 3. of positive ion, such as NO , we add the number Distribute the remaining valence electrons on the atomselectrons asthen in the ion and lone pai subtract the number of positive charges on the ion (one electron is lost for each single positive charge) 4. Place all remaining electrons on thevalence central atom. shells with an octet of electrons. from the total number of valence electrons: + ◦ For SiH4, and NO , there ◦ are no remaining There electrons; electrons are no remaining we alreadyon placed SiH4,all soofi determined in Step 1. When several ◦ arrangements For OF2, we had of16 atoms are possible, as for electrons Step 3, andwe wemust use experimental evid Step 3: Distribute the remaining electrons as loneremaining pairs oninthe terminal placed 12, leaving 4 to be pl choose the correct atom: one. In general, the less electronegative elements are more likely to be central atoms (except H) to thecomplete an octet around each atom less electronegative carbon atom occupies the central position with the oxygen and h atoms surrounding it. Other examples include P in POCl3, S in SO2, and Cl in An exception hydrogen is almost never a central atom. As the most electronegative element, nuorine also canno Step 4: Place all remaining electrons 5. Rearrange on the central the electrons 4.atom. of the outer atomsall Place to remaining make multiple bonds with electrons thecentral on the central at at central atom. octets wherever possible. ◦ For SiH4, and NO+, there are no rema 3. Distribute the remaining electrons as lone pairs on the terminal atoms (except hydrogen) to comp ◦ SiH4: Si already has an octet, so nothing needs to in determined beStep done.1. Step5: Rearrange valencethe electrons shells of the with an octet outer atoms to make of electrons. multiple bonds with the central We ◦ Since◦OF2 is a neutral have distributed molecule, we simply add the valence the number ◦is For electrons of valence as lone electrons: OF2, we had 16 pairs onremaining electrons the oxygeninatoms Ste atom in order to obtain ◦ There octets are no remaining wherever electrons atom lacks an octet: possible. on SiH 4 , so it unchanged: atom: 5. Rearrange the electrons of the outer atoms to m choose ◦ For OF2, we had the correct 16total electrons one. In general, number of remaining in Step the lesswe 3, and electronegative placed 12, leaving el subtract the number of positive charges on the ion (one electron is lost for each single positive Determine atom:the Lewisoctets wherever from the Structure the lessof NOpossible. valence electrons: + electronegative carbon atom occupies the c SiH ◦ atoms Step 1: Total number of : Si alreadyit. 4surrounding valence electrons hasOtheran examples octet, so includenothingP needs in POCl to be 3, S in 7.3 Lewis Symbols ◦ hydrogen is almost We have never distributed a central atom. the As valence the most electrons electron central 5. Rearrange atom atom. lacks an the electrons octet: of the outer atoms to make multiple bonds with the 3. wherever octets Distribute the remaining electrons as lone pairs on the termi possible. ◦ SiH4: Sivalence already shells has an withoctet,an so octet nothing of needs electrons. to be done. Step 2: Draw◦ skeleton ◦ ThereWe have are no structure distributed remaining the electrons valence electrons on SiHas, lone so it pairs on the oxy is unchanged ◦ Since OF2 is a neutral molecule, we simply add the number of4valence electrons: atom lacks an octet: Step 3: Distribute the remaining electrons as lone pairs on the terminal arrangements atoms (except of H) atoms are+:possible, to◦complete NO an octet For as around this for ion,each addedweeight weatom mustvalence use experimental electronse rect one. In general, the less electronegative elements are more likely to be cent Step 4: Place all remaining more Drawelectrons 2.electrons on structure a skeleton since the central we orhave atom. of the molecule already ion, arranging the atomsused the atom around a central tota an ss electronegative ◦ NO+:carbon Forform atom thisconnecting ion, weoccupies each atom to thethe added eight central central atom with aposition valence electrons, but with single (one electron the pair) neither oxygen bond. has an (Note that we atom and witha multiple brackets around the bond: structure, indicating the charge outside the brackets:) ding it. Other Step5: examples Rearrange 4. more Place include the electrons electrons allofsincethePwe remaining in have outerPOCl 3, Stoin electrons atoms already SO makeon used ,the and the 2multiple Clbonds in central total that atom. with we theAn found inexcep central Step 1 most never atom a central in order to ◦ aatom. obtain form Foroctets SiH multiple As , the most wherever 4bond: possible. and NO+, there are electronegative element, no remainingnuorine also ca electrons determined in Step 1. Access for free at openstax.org remaining electrons ◦ For asThis OF2,pairs lone we had on16 the electrons terminal remaining atoms in Step (except 3, and wetopla still does not produce an octet, sohydrogen) we must mo co with an octet ofThis atom: electrons. still does not produce an octet, so we must move another pair, forming emaining electrons on SiH4, so it is unchanged: central atom. When several arrangements of atoms are possible, as for we must use exp Determine the Lewis Structure of OF 3. Distribute the remaining electro choose◦ the correct Since OF2 is one. In general, a neutral molecule, the less electronegative 2 we simply add thevalence number of elements valence are more like electrons: shells with an octet of ele the lessofelectronegative Step 1: Total number valence electrons carbon atom occupies the central position with th ◦ There are no remaining electron atoms surrounding it. Other examples include P in POCl3, S in SO2, and Cl in hydrogen is almost never a central atom. As 7.3 the most Lewis electronegative Symbols and Structures element,327 nuo central atom. 3. Distribute the remaining electrons as lone pairs on the terminal atoms (except hyd valence 2. shells Draw a with an structure skeleton octet of ofelectrons. the molecule or ion, arranging the atoms around a central ato StepThere ◦ areconnecting 2: Draw each atom no remaining skeleton structure to the central electrons on SiHatom, so 4 withitaissingle (one electron pair) bond. (Note that unchanged: 4. Place all remaining electrons on with brackets around the structure, indicating the charge outside the brackets:) ◦ For SiH4, and NO+, ther Step 3: Distribute the remaining electrons as lone pairs ondetermined the terminal in Step 1. ents of atoms atoms are H) (except possible, as for an octet around to complete we must useatom each experimental evidence to In general, the less electronegative elements are more likely ◦ toFor beOF 2, weatoms. central had 16Inelectrons rem onegative carbon atom Access occupies for free atom: and hydrogen the central position with the oxygen at openstax.org therStep 4: Placeinclude examples all remaining electrons P in POCl on2,the 3, S in SO andcentral Cl in atom. An exception is that 4. Place all remaining electrons on the central atom. er a central atom. As the most electronegative element, nuorine also cannot be a ◦ For SiH4, and NO+, there are no remaining electrons; we already placed a determined in Step 5. Rearrange the electrons of the ou 1. terminal atoms (except hydrogen) g electrons as lone pairs on the to complete their octets For OF2, we had 16 electrons remaining in Step 3, and we ctet◦of electrons. wherever possible. placed 12, leaving 4 to b atom: electrons on SiH4, so it is unchanged: ◦ SiH4: Si already has an octet, so n ◦ We have distributed th atom lacks an octet: Practice: Determine the Lewis Structure of H3CCH3, HCCH, NH3, CO, CO2 Exception to the Octet Rule Many covalent molecules have central atoms that do not have eight electrons in their Lewis structures. These molecules fall into three categories: 1. Odd-electron molecules have an odd number of valence electrons, and therefore have an unpaired electrons. These molecules are known as free radicals. Nitric oxide (NO): 2. Electron-deficient molecules have a central atom that has fewer electrons than needed for a noble gas configuration. Beryllium Boron dihydride (BeH2): Trifluoride (BF3): 3. Hypervalent molecules have a central atom that has more electrons than needed for a noble gas configuration. Phosphorus Sulfur pentachloride (PCl5): Hexafluoride (SF6): Resonance Structures If two or more Lewis structures with the same arrangements of atoms can be written for a molecule or ion, the actual distribution of electrons is an average of that shown by the various Lewis structures. The individual Lewis structures is known as resonance form, and the actual electronic structure of the molecule (the average of the resonance forms) is called a resonance hybrid of the individual resonance forms. For example, the Lewis structure for nitrite (NO2-) ion can be written in two different ways: A double-bonded arrow between those Lewis structures indicates that they are resonance forms: Resonance Structures The carbonate (CO32-) ion provides another example of resonance: Bond Strength: Covalent Bond A bond’s strength describes how strongly each atom is joined to another atom, and therefore how much energy, known as bond energy, is required to break the bond between the two atoms. The bond energy for a molecule is defined as the standard enthalpy change for the reaction. For example, the sum of the four C-H bond energies in methane (CH4), 1660 kJ, is equal to the standard enthalpy change for the reaction: Therefore, the average C-H bond energy is 1660/4 = 415 kJ/mol. The strength of a bond between two atoms increases as the number of electron pairs in the bond increases. Generally, as the bond strength increases, the bond length decreases. Therefore, double bonds are stronger and shorter than single bonds and triple bonds are stronger and shorter than double bonds. C–P 265 F–S 285 Average bond lengths and bond energies for some TABLE 7.2 common bonds. Average Bond Lengths and Bond Energies for Some Common Bonds Bond Bond Length (Å) Bond Energy (kJ/mol) C–C 1.54 345 1.34 611 1.20 837 C–N 1.43 290 1.38 615 1.16 891 C–O 1.43 350 1.23 741 1.13 1080 reaction: ymbol means “the sum of” H, for aƩchemical reaction isand D representsequal approximately the bond energy to the sum veThenumber. enthalpy The bond change, ΔH, energy for is obtained a chemical reaction isfrom a table (like Table approximately eactants (energy “in”, positive sign) plus the energy equal to released bond is aofsingle, the sum double, the energy orto required triple breakbond. Thus, all bonds in thein calculating reactants enth minus the “out,” negative sign). This can be expressed energy released when all bonds are formed in the products. mathematically nsider the bonding in all reactants and products. Because D valu bond in many different Ʃ molecules, thisƩcalculation provides a r alpy Cl, of mole one reaction. of H–H bonds and one mole of Cl–Cl bonds must be symbol bonds is means Ʃ the sum“the sum of the of”energy bond and Dofrepresents the H–H bondthe bond ene (436 kJ/m eaction: Consider the following reaction: ve number.two e reaction, The bondofenergy moles is obtained H–Cl bonds from(bond are formed a table (like = energy Ta4 bond is cause thea bonds single,indouble, or triple the products arebond. Thus, stronger thaninthose calculating e in the rea nsider the bonding in all reactants and products. Because D v han it consumes: bond in many different molecules, this calculation provides halpy of reaction. Ʃ Ʃ Cl, one mole of H–H bonds and one mole of Cl–Cl bonds must be reaction: bonds is the sum of the bond energy of the H–H bond (436 kJ/m ayPractice be an excellent : alternative fuel. The high-temperature reaction of f the gases carbon monoxide, CO, and hydrogen, H2, from which met bond energies Using the bond in Tablein7.3, energies Tablecalculate the approximate 7.3 in textbook, enthalpy chang calculate the approximate enthalpy change, ΔH, for the following reaction: e the Lewis structures of the reactants and the products: t ΔH for this reaction involves the energy required to break a C–O tri well as the energy produced by the formation of three C–H single bo ngle bond. We can express this as follows: the electrostatic attraction between its positive and negative ions. The ureBond Strength: of the strength Ionic of this Bond The lattice energy (ΔHlattice) of an attraction. y required to separate one mole of the solid into its component gaseous Anisionic nergy compound the enthalpy is stable change of thebecause process:of the electrostatic attraction between its positive and negative ions. The lattice energy of a compound is a measure of the strength of its attraction. The where thelattice energy ionic solid (𝚫Hlattice)into is separated of an ionic ions, so compound is defined our lattice energies willas the textsenergy use therequired equivalentto separate oneconvention, but opposite mole of thedemning solid into its component lattice arategaseous ions. to form a lattice and giving negative (exothermic) ions combine eFor example, energies for thereference, in another ionic solidbeMX, the to certain lattice checkenergy which is the enthalpy demnition is change nitude for of the process: lattice energy indicates a more stable ionic compound. For s, it requires MX769(s) kJ ----> + Mn+ (g)one to separate + Xmole n- (g) of solid 𝚫HNaCl lattice into gaseous Na seous Na+ and Cl– ions form solid NaCl, 769 kJ of heat is released. 𝚫Hlattice can be expressed by the following equation (derived from ystal can be expressed by the following equation (derived from Coulomb’s law, governing the forces between electric charges): ween electric charges): C is a constant that depends on the type of crustal structure; Z+ and Z- are the charges on the ions; and Ro is the interionic distance. n the type of crystal structure; Z+ and Z– are the charges on the ions; Molecular Structure and Polarity A bond angle is the angle between any two bonds that include a common atom, usually measured in degrees. A bond distance (or bond length) is the distance between the nuclei of two bonded atoms along the straight line joining the nuclei. Bond distances are measured in Angstroms (1 Å = 10-10 m) or picometers (1 pm = 10-12 m). Bond distances (lengths) and angles are shown for the formaldehyde molecule, H2CO. VSEPR Theory Valence shell electron-pair repulsion theory (VSEPR theory) enables us to predict the molecular structure, including approximate bond angles around central atom, of a molecule from an examination of the number of bonds and lone electron pairs in its Lewis structure. 1. The VSEPR model assumes that electron pairs in the valence shell of a central atom will adopt an arrangement that minimizes repulsions between these electron pairs by maximizing the distance between them. 2. The electrons in the valence shell of a central atom form either bonding pairs of electrons, located primarily between bonded atoms, or lone pairs. 3. The electrostatic repulsion of these electrons is reduced when the various regions of high electron density assume positions as far from each other as possible. The BeF2 molecule adopts a linear structure in which the two bonds are as far apart as possible, on opposite sides of the Be atom. The bond angle is 1800. The basic electron-pair geometries predicted by VSEPR theory maximize the space around any region of electron density (bonds or lone pairs). # Geometry Regions 2 Linear Trigonal 3 planar 4 Tetrahedral Trigonal 5 bipyramidal 6 Octahedral Electron-pair Geometry vs Molecular Structure Electron-pair geometry around a central atom (VSEPR model) is not the same thing as its molecular structure. Electron-pair geometry describes all regions where electrons are located, bonds as well as lone pairs. Molecular structure describes the location of atoms, not the electrons. The electron-pair geometries will be the same as the molecular structures when there are no lone electron pairs around the central atom, but they will be different when there are lone pairs present on the central atom. Electron-pair Geometry vs Molecular Structure Methane (CH4) has four bonding pairs of electrons around the central carbon atom and therefore, the electron-pair geometry is tetrahedral and so is the molecular structure. VSEPR structures like this one are often drawn using the wedge and dash notation, in which solid lines represent bonds in the plane of the page, solid wedges represent bonds coming up out of the plane, and dashed lines represent bonds going down into the plane. Ammonia (NH3) also has four electron pairs associated with the nitrogen atom, and thus has a tetrahedral electron-pair geometry. One of these regions, however, is a lone pair, which is not included in the molecular structure, and this lone pair influences the shape of the molecule. (a) The electron-pair geometry for the ammonia molecule is tetrahedral with one lone pair and three single bonds. (b) The trigonal pyramidal molecular structure is determined from the electron-pair geometry. (c) The actual bond angles deviate slightly from the idealized angles because the lone pair takes up a larger region of space than do the single bonds, causing the HNH angle to be slightly smaller than 109.5°. Molecular Structure VSEPR theory predicts the distortions caused by various regions of electron density by establishing an order of repulsions and an order of the amount of space occupied by different kinds of electron pairs. The order of electron-pair repulsions from greatest to least repulsion is: lone pair-lone pair > lone-pair-bonding pair > bonding pair-bonding pair This order of repulsions determines the amount of space occupied by different regions of electrons. The order of sizes from largest to smallest is: lone pair > triple bond > double bond > single bond This molecule of formaldehyde (H2CO) has regions of electron density that consist of two single bonds and one double bond. The basic geometry should be the trigonal planar with 1200, but because of the higher occupied space by double bond electrons, the angle between the single bonds is slightly smaller (1180). The molecular structures are identical to the electron-pair geometries when there are no lone pairs present (first column). For a particular number of electron pairs (row), the molecular structures for one or more lone pairs are determined based on modifications of the corresponding electron-pair geometry. Molecular Structure for Trigonal Bipyramid For trigonal bipyramidal electron-pair geometries, there are two distinct positions: an axial position and an equatorial position. The axial position is surrounded by bond angles of 90o, whereas the equatorial position has more space available because of the 120o bond angles. Therefore, in a trigonal bipyramidal electron-pair geometry, lone pairs always occupy equatorial positions over axial positions. (b–d) The two lone pairs (red lines) in ClF3 have several possible arrangements, but the T- shaped molecular structure (b) is the one actually observed, consistent with the larger lone pairs both occupying equatorial positions. Practice : Predict the electron-pair geometry and molecular structures of the following molecules: CO2, BCl3, NH4+, CO32-, PF5, H3O+, SF4, XeF4 Molecular Polarity and Dipole Moment Polar covalent bonds connect two atoms with different electronegativities, leaving one atom with a partial positive charge (𝛿+) and the other atom with a partial negative charge (𝛿-), as the electrons are pulled toward the more electronegative atom. This separation of charge gives rise to a bond dipole moment. The magnitude of a bond dipole moment is represented by mu (µ = Qr), where Q is the magnitude of the partial charges (determined by the electronegativity difference) and r is the distance between the charges. The bond dipole moment can be represented as a vector, a quantity having both direction and magnitude. Dipole vectors are shown as arrows pointing along the bond from the less electronegative atom toward the more electronegative atom. A small plus sign is drawn on the less electronegative end to indicate the partially positive end of the bond. The length of the arrow is proportional to the magnitude of the electronegativity difference between the two atoms. (a) There is a small difference in electronegativity between C and H, represented as a short vector. (b) The electronegativity difference between B and F is much larger, so the vector representing the bond moment is much longer. CO2 is nonpolar but H2O is polar The overall dipole moment of a molecule depends on the individual bond dipole moments and how they are arranged. (a) Each CO bond has a bond dipole moment, but they point in opposite directions so that the net CO2 molecule is nonpolar. (b) In contrast, water is polar because the OH bond moments do not cancel out. Overall Dipole Moment The OCS molecule has a structure similar to CO2, but a sulfur atom has replaced one of the oxygen atoms. The C-O bond is considerably polar. Although C and S have very similar electronegativity values, S is slightly more electronegative than C, and so C-S bond is slightly polar. Because oxygen is more electronegative than sulfur, the oxygen end of the molecule is the negative end for the overall dipole moment of OCS. Chloromethane (CH3Cl) is a tetrahedral molecule with three slightly polar C-H bonds and a more polar C-Cl bond. The relative electronegativity values of the bonded atoms is H < C < Cl. Therefore, the bond moments all point toward the Cl end of the molecule and sum to yield a considerable dipole moment. What makes a molecule polar? 1. Contain at least one polar covalent bond. 2. Have a molecular structure such that the sum of the vectors of each bond dipole moment does not cancel. Properties of Polar Molecules (a) Molecules are always randomly distributed in the liquid state in the absence of an electric field. (b) When an electric field is applied, polar molecules like HF will align to the dipoles with the field direction.