Chapter 9 - Electricity PDF
Document Details
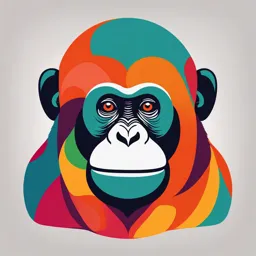
Uploaded by DenxTV
Stonyhurst Southville International School
Tags
Summary
This document is an educational chapter about electricity, covering topics such as electric current, electric potential difference, and resistance. It details how electric current is a flow of charge and how differences in electric potential drive the movement of charges in circuits.
Full Transcript
Electricity BIG IDEA: Energy flows in a circuit to produce electricity. ESSENTIAL QUESTION: How do different parts of the electrical system work together to enable devices to work? How can varying components affect the output of the system? 9. 1 Electricity ELECTRIC CURRENT If the two requirements o...
Electricity BIG IDEA: Energy flows in a circuit to produce electricity. ESSENTIAL QUESTION: How do different parts of the electrical system work together to enable devices to work? How can varying components affect the output of the system? 9. 1 Electricity ELECTRIC CURRENT If the two requirements of an electric circuit are met, then charge will flow through the external circuit. It is said that there is a current - a flow of charge. Using the word current in this context is to simply use it to say that something is happening in the wires - charge is moving. Yet current is a physical quantity that can be measured and expressed numerically. As a physical quantity, current is the rate at which charge flows past a point on a circuit. As depicted in the diagram below, the current in a circuit can be determined if the quantity of charge Q passing through a cross section of a wire in a time t can be measured. The current is simply the ratio of the quantity of charge and time. Current is a rate quantity. There are several rate quantities in physics. For instance, velocity is a rate quantity - the rate at which an object changes its position. Mathematically, velocity is the position change per time ratio. Acceleration is a rate quantity - the rate at which an object changes its velocity. Mathematically, acceleration is the velocity change per time ratio. And power is a rate quantity - the rate at which work is done on an object. Mathematically, power is the work per time ratio. In every case of a rate quantity, the mathematical equation involves some quantity over time. Thus, current as a rate quantity would be expressed mathematically as Note that the equation above uses the symbol I to represent the quantity current. As is the usual case, when a quantity is introduced in The Physics Classroom, the standard metric unit used to express that quantity is introduced as well. The standard metric unit for current is the ampere. Ampere is often shortened to Amp and is abbreviated by the unit symbol A. A current of 1 ampere means that there is 1 coulomb of charge passing through a cross section of a wire every 1 second. 1 ampere = 1 coulomb / 1 second Conventional Current Direction The particles that carry charge through wires in a circuit are mobile electrons. The electric field direction within a circuit is by definition the direction that positive test charges are pushed. Thus, these negatively charged electrons move in the direction opposite the electric field. But while electrons are the charge carriers in metal wires, the charge carriers in other circuits can be positive charges, negative charges or both. In fact, the charge carriers in semiconductors, street lamps and fluorescent lamps are simultaneously both positive and negative charges traveling in opposite directions. Ben Franklin, who conducted extensive scientific studies in both static and current electricity, envisioned positive charges as the carriers of charge. As such, an early convention for the direction of an electric current was established to be in the direction that positive charges would move. The convention has stuck and is still used today. The direction of an electric current is by convention the direction in which a positive charge would move. Thus, the current in the external circuit is directed away from the positive terminal and toward the negative terminal of the battery. Electrons would actually move through the wires in the opposite direction. Knowing that the actual charge carriers in wires are negatively charged electrons may make this convention seem a bit odd and outdated. Nonetheless, it is the convention that is used worldwide and one that a student of physics can easily become accustomed to. ELECTRIC POTENTIAL DIFFERENCE Electric potential is a location-dependent quantity that expresses the amount of potential energy per unit of charge at a specified location. When a Coulomb of charge (or any given amount of charge) possesses a relatively large quantity of potential energy at a given location, then that location is said to be a location of high electric potential. And similarly, if a Coulomb of charge (or any given amount of charge) possesses a relatively small quantity of potential energy at a given location, then that location is said to be a location of low electric potential. As we begin to apply our concepts of potential energy and electric potential to circuits, we will begin to refer to the difference in electric potential between two points. This part will be devoted to an understanding of electric potential difference and its application to the movement of charge in electric circuits. Consider the task of moving a positive test charge within a uniform electric field from location A to location B as shown in the diagram at the right. In moving the charge against the electric field from location A to location B, work will have to be done on the charge by an external force. The work done on the charge changes its potential energy to a higher value; and the amount of work that is done is equal to the change in the potential energy. As a result of this change in potential energy, there is also a difference in electric potential between locations A and B. The standard metric unit on electric potential difference is the volt, abbreviated V and named in honor of Alessandro Volta. One Volt is equivalent to one Joule per Coulomb. If the electric potential difference between two locations is 1 volt, then one Coulomb of charge will gain 1 joule of potential energy when moved between those two locations. If the electric potential difference between two locations is 3 volts, then one coulomb of charge will gain 3 joules of potential energy when moved between those two locations. And finally, if the electric potential difference between two locations is 12 volts, then one coulomb of charge will gain 12 joules of potential energy when moved between those two locations. Because electric potential difference is expressed in units of volts, it is sometimes referred to as the voltage. ELECTRIC POTENTIAL DIFFERENCE AND SIMPLE CIRCUITS Electric circuits, as we shall see, are all about the movement of charge between varying locations and the corresponding loss and gain of energy that accompanies this movement. In the previous part of Lesson 1, the concept of electric potential was applied to a simple battery-powered electric circuit. In that discussion, it was explained that work must be done on a positive test charge to move it through the cells from the negative terminal to the positive terminal. This work would increase the potential energy of the charge and thus increase its electric potential. As the positive test charge moves through the external circuit from the positive terminal to the negative terminal, it decreases its electric potential energy and thus is at low potential by the time it returns to the negative terminal. If a 12 volt battery is used in the circuit, then every coulomb of charge is gaining 12 joules of potential energy as it moves through the battery. And similarly, every coulomb of charge loses 12 joules of electric potential energy as it passes through the external circuit. The loss of this electric potential energy in the external circuit results in a gain in light energy, thermal energy and other forms of non-electrical energy. With a clear understanding of electric potential difference, the role of an electrochemical cell or collection of cells (i.e., a battery) in a simple circuit can be correctly understood. The cells simply supply the energy to do work upon the charge to move it from the negative terminal to the positive terminal. By providing energy to the charge, the cell is capable of maintaining an electric potential difference across the two ends of the external circuit. Once the charge has reached the high potential terminal, it will naturally flow through the wires to the low potential terminal. The movement of charge through an electric circuit is analogous to the movement of water at a water park or the movement of roller coaster cars at an amusement park. In each analogy, work must be done on the water or the roller coaster cars to move it from a location of low gravitational potential to a location of high gravitational potential. Once the water or the roller coaster cars reach high gravitational potential, they naturally move downward back to the low potential location. For a water ride or a roller coaster ride, the task of lifting the water or coaster cars to high potential requires energy. The energy is supplied by a motor-driven water pump or a motor-driven chain. In a battery-powered electric circuit, the cells serve the role of the charge pump to supply energy to the charge to lift it from the low potential position through the cell to the high potential position. It is often convenient to speak of an electric circuit such as the simple circuit discussed here as having two parts - an internal circuit and an external circuit. The internal circuit is the part of the circuit where energy is being supplied to the charge. For the simple battery-powered circuit that we have been referring to, the portion of the circuit containing the electrochemical cells is the internal circuit. The external circuit is the part of the circuit where charge is moving outside the cells through the wires on its path from the high potential terminal to the low potential terminal. The movement of charge through the internal circuit requires energy since it is an uphill movement in a direction that is against the electric field. The movement of charge through the external circuit is natural since it is a movement in the direction of the electric field. When at the positive terminal of an electrochemical cell, a positive test charge is at a high electric pressure in the same manner that water at a water park is at a high water pressure after being pumped to the top of a water slide. Being under high electric pressure, a positive test charge spontaneously and naturally moves through the external circuit to the low pressure, low potential location. As a positive test charge moves through the external circuit, it encounters a variety of types of circuit elements. Each circuit element serves as an energy-transforming device. Light bulbs, motors, and heating elements (such as in toasters and hair dryers) are examples of energy-transforming devices. In each of these devices, the electrical potential energy of the charge is transformed into other useful (and non-useful) forms. For instance, in a light bulb, the electric potential energy of the charge is transformed into light energy (a useful form) and thermal energy (a non-useful form). The moving charge is doing work upon the light bulb to produce two different forms of energy. By doing so, the moving charge is losing its electric potential energy. Upon leaving the circuit element, the charge is less energized. The location just prior to entering the light bulb (or any circuit element) is a high electric potential location; and the location just after leaving the light bulb (or any circuit element) is a low electric potential location. Referring to the diagram above, locations A and B are high potential locations and locations C and D are low potential locations. The loss in electric potential while passing through a circuit element is often referred to as a voltage drop. By the time that the positive test charge has returned to the negative terminal, it is at 0 volts and is ready to be re-energized and pumped back up to the high voltage, positive terminal. RESISTANCE An electron traveling through the wires and loads of the external circuit encounters resistance. Resistance is the hindrance to the flow of charge. For an electron, the journey from terminal to terminal is not a direct route. Rather, it is a zigzag path that results from countless collisions with fixed atoms within the conducting material. The electrons encounter resistance - a hindrance to their movement. While the electric potential difference established between the two terminals encourages the movement of charge, it is resistance that discourages it. The rate at which charge flows from terminal to terminal is the result of the combined effect of these two quantities. Electrical Symbols Electricity in the Workplace Places of work generally have power nominally supplied at 230 volt (single phase) and 400 volt (3 phase) although some larger workplaces will receive electricity at a higher supply voltage. The information below relates to workplaces using 230 and 400 volt supplies. The main hazards with electricity are: contact with live parts causing shock and burns faults which could cause fires; fire or explosion where electricity could be the source of ignition in a potentially flammable or explosive atmosphere, e.g. in a spray paint booth The risk of injury from electricity is strongly linked to where and how it is used and there is greater risk in wet and/or damp conditions. Basics of Contact with Electricity It is the level of voltage the body is exposed to and the resistance to flow of electrical current offered by the body that determines the impact of exposure to electricity. The following factors determine the severity of the effect electric shock has on your body: The level of voltage The amount of body resistance you have to the current flow The path the current takes through your body The length of time the current flows through your body If a worker has come into contact with electricity the worker may not be able to remove themselves from the electrical source. The human body is a good conductor of electricity. If you touch a person while they are in contact with the electrical source, the electricity will flow through your body causing electrical shock. Firstly attempt to turn off the source of the electricity (disconnect). If the electrical source cannot readily and safely be turned off, use a non-conducting object, such as a fibreglass object or a wooden pole, to remove the person from the electrical source. As an Employer it is YOUR responsibility to ensure: Extension cables and other flexible leads which are particularly prone to damage to plugs and sockets and to their connections are visually checked, maintained and where necessary replaced before using portable equipment. The ends of flexible cables should always have the outer sheath of the cable firmly clamped to stop the wires (particularly the earth) pulling out of the terminals Use the correct cable connectors or couplers to join lengths of cables together and do not allow taped joints. Electrical installations are installed and maintained by a competent person and checked regularly Socket Outlets are not overloaded by the use of adaptors Electrically powered equipment provided is suitable for use Fixed electrical equipment should have a clearly identified switch to cut off power in an emergency that portable equipment labelled as being double insulated has had the live and neutral connected properly to the plug by a competent person unless the plug is of a moulded type Controlling the Risk Reduce the Voltage Often portable equipment is available that is powered from a 110 volt supply through a simple transformer and these are often centre tapped to earth so that the maximum voltage between a live conductor and earth (the most common cause of electric shocks from equipment) is limited to 55V. Battery operated tools such as drills, screwdrivers etc can replace mains powered equipment Temporary and hand held lighting can be provided at 12, 25, 50 or 110 volts. Ensure Fuses are correctly fitted The fuse protects the device from over current. It is designed to ‘blow’ and cut off the electricity when the current exceeds its rated capacity. It is important to ensure the correct fuse is used for the appliance. As a general guide 3 amp fuses are used in equipment up to 700 watts (W). For equipment with a rating greater than 700 watts (W) a 13-amp fuse will be required. Some equipment requires a 5 amp fuse e.g. some televisions and, other equipment like some printers require 10 amp fuses. Always read the manufacturer’s instructions.