Chapter 7: Lymphocyte Receptor Signaling PDF
Document Details
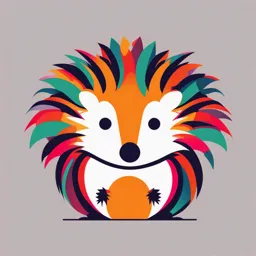
Uploaded by VigilantJasper
Tags
Summary
This chapter details lymphocyte receptor signaling, outlining the development of B and T lymphocytes and general principles of signal transduction. It also covers antigen receptor signaling, co-stimulatory signaling, and inhibitory receptors.
Full Transcript
PART III The development of mature lymphocyte receptor repertoires 7 8 Lymphocyte Receptor Signaling The Development of B and T Lymphocytes Lymphocyte Receptor Signaling T and B lymphocytes are cells of the adaptive immune system, each of which expresses a unique antigen receptor. These cells circ...
PART III The development of mature lymphocyte receptor repertoires 7 8 Lymphocyte Receptor Signaling The Development of B and T Lymphocytes Lymphocyte Receptor Signaling T and B lymphocytes are cells of the adaptive immune system, each of which expresses a unique antigen receptor. These cells circulate between the blood, the lymph, and, most importantly, the secondary lymphoid organs, where they survey antigen-presenting cells for their specific antigen. Once that antigen is encountered, signals from the antigen receptor activate several downstream pathways that convert quiescent naive lymphocytes into metabolically active cells that are reorganizing their actin cytoskeleton, activating transcription factors, and synthesizing a wide range of new proteins. As a result of these events, naive T and B cells undergo rapid cell division and differentiate into armed effector cells, thus expanding lymphocyte populations during an immune response and equipping these cells with the machinery to combat infections. We begin by discussing some general principles of intracellular signaling, and then outline the pathways activated when a naive lymphocyte encounters its specific antigen. Next, we briefly discuss the co-stimulatory signaling that is necessary to activate naive T cells and, in most cases, naive B cells. In the last part of the chapter we focus on inhibitory receptors, and their roles in downregulating signaling pathways in T and B cells. General principles of signal transduction and propagation. In this part of the chapter we review briefly some general principles of receptor action and signal transduction that are common to many of the pathways discussed here. All cell-surface receptors that have a signaling function either are transmembrane proteins themselves or form parts of protein complexes that link the exterior and interior of the cell. Different classes of receptors transduce extracellular signals in a variety of ways. A common theme among the receptors covered in this chapter is that ligand binding results in the activation of an intracellular enzymatic activity. ERRNVPHGLFRVRUJ 7 IN THIS CHAPTER General principles of signal transduction and propagation. Antigen receptor signaling and lymphocyte activation. Co-stimulatory and inhibitory receptors modulate antigen receptor signaling in T and B lymphocytes. 258 Chapter 7: Lymphocyte Receptor Signaling 7-1 Transmembrane receptors convert extracellular signals into intracellular biochemical events. The enzymes most commonly associated with receptor activation are the protein kinases. This large group of enzymes catalyzes the covalent attachment of a phosphate group to a protein, a reversible process called phosphorylation. For receptors that use protein kinases, the binding of ligand to the extracellular part of the receptor allows the receptor-associated protein kinase to become ‘active’—that is, to phosphorylate its intracellular substrate—and thus to propagate the signal. As we shall see, receptor-associated kinases can become activated in various ways, such as by undergoing modifications to the kinase itself that alter its intrinsic catalytic efficiency or by changes in subcellular localization that increase access to its biochemical substrates. In animals, protein kinases phosphorylate proteins on three amino acids— tyrosine, serine, or threonine. Most of the enzyme-linked receptors we discuss in detail in this chapter activate tyrosine protein kinases. Tyrosine kinases are specific for tyrosine residues, whereas serine/threonine kinases phosphorylate serine and threonine residues; less common are dual-specificity kinases that phosphorylate both tyrosine and serine/threonine residues in their substrates. Protein tyrosine phosphorylation is much less common than serine/threonine phosphorylation in general, and is employed mainly in signaling pathways. One large group of receptors—the so-called receptor tyrosine kinases— carry a kinase activity within the cytoplasmic region of the receptor itself (Fig. 7.1, top panel). This group contains receptors for many growth factors; lymphocyte receptors of this type include Kit and FLT3, which are expressed on developing lymphocytes in addition to other hematopoietic progenitor cells and are discussed in Chapter 8. The receptor for transforming growth factor-β (TGF‑β), an important regulatory cytokine produced by many cells, is a receptor serine/threonine kinase. Even more important to the function of mature lymphocytes are receptors that have no intrinsic enzymatic activity themselves but associate with intracellular tyrosine kinases. The antigen receptors on B lymphocytes and T lymphocytes are of this type, as are the receptors for some types of cytokines. Ligand binding to the extracellular domain of such receptors causes particular amino acid residues in their cytoplasmic domains to become phosphorylated by specific cytoplasmic tyrosine kinases (see Fig. 7.1, bottom panel). These nonreceptor kinases either can be constitutively associated with the cytoplasmic domains of the receptors, as with many cytokine receptors, or may become associated with the receptors when they bind their ligands, as is the case for the antigen receptors. For many cytokine receptors, ligand binding causes dimerization or clustering of individual receptor molecules, bringing the associated kinases together and enabling them to phosphorylate the cytoplasmic tail of adjacent receptors— thus initiating an intracellular signal. In the case of the lymphocyte antigen receptors, association with cytoplasmic tyrosine kinases occurs after ligand binding but is unlikely to be due to a simple clustering mechanism. Instead, the actions of co-receptors are required: these bring cytoplasmic tyrosine kinases into proximity with the cytoplasmic regions of the antigen receptor, a complex process that we will describe later. Signaling is usually not a simple ‘on or off’ switch. Depending on the affinity of the receptor for the ligand, the abundance of the ligand, the concentrations of intracellular signaling components, and a complex network of positiveand negative-feedback pathways, receptor activation and downstream signaling occur when a minimum threshold determined by all of these factors is exceeded. These features are often merged into the simple term ‘signal ERRNVPHGLFRVRUJ General principles of signal transduction and propagation. In one class of receptors, the kinase domain is an intrinsic part of the receptor Ligand binding dimerizes the receptor, activating the kinases, which phosphorylate each other The activated kinases phosphorylate downstream substrates Ligand binding dimerizes the receptor, activating the associated kinases, which phosphorylate each other The activated kinases phosphorylate downstream substrates kinase domain In another class of receptors, a kinase is noncovalently associated with the receptor kinase Immunobiology | chapter 7 | 07_001 Murphy et al | Ninth edition © Garland Science design by blink studio limited strength.’ It is important to keep in mind that variations in signal strength will determine the magnitude of cellular responses—some will be all-or-nothing, whereas others will increase as the strength of signaling increases. The role of protein kinases in cell signaling is not confined to receptor activation, as they act at many different stages in intracellular signaling pathways. Protein kinases figure largely in cell signaling because phosphorylation and dephosphorylation—the removal of a phosphate group—are the means of regulating the activity of many enzymes, transcription factors, and other proteins. Equally important to the workings of signaling pathways is the fact that phosphorylation generates sites on proteins to which other signaling proteins can bind. Phosphate groups are removed from proteins by a large class of enzymes called protein phosphatases. Different classes of protein phosphatases remove phosphate groups from phosphotyrosine or from phosphoserine/ phosphothreonine, or both (as in dual-specificity phosphatases). Specific dephosphorylation by phosphatases is one important means of regulating signaling pathways by resetting a protein to its original state and thus switching signaling off. Dephosphorylation does not always inhibit a protein’s activity. In many instances the removal of a particular phosphate group by a specific phosphatase is needed to activate an enzyme. In other cases, the extent of phosphorylation of an enzyme determines its activity, and represents a balance between the activity of kinases and phosphatases. ERRNVPHGLFRVRUJ Fig. 7.1 Enzyme-associated receptors of the immune system can use intrinsic or associated protein kinases to signal. These receptors activate a protein kinase on the cytoplasmic side of the membrane to convey the information that a ligand has bound to their extracellular portion. Receptor tyrosine kinases (top panels) contain the kinase activity as part of the receptor itself. Ligand binding results in clustering of the receptor, activation of catalytic activity, and the consequent phosphorylation (denoted by red dots) of the receptor tails and other substrates, transmitting the signal onward. Receptors that lack intrinsic kinase activity associate with nonreceptor kinases (bottom panels). Receptor dimerization or clustering after ligand binding activates the associated enzyme. In all receptors of these types encountered in this chapter, the enzyme is a tyrosine kinase. 259 260 Chapter 7: Lymphocyte Receptor Signaling 7-2 Intracellular signal propagation is mediated by large multiprotein signaling complexes. As we learned in Chapter 3, binding of a ligand to its receptor can initiate a cascade of events involving intracellular proteins that sequentially convey signaling information onward. The unique enzymes and other components assembled into a particular multiprotein receptor complex will determine the character of the signal it generates. These components may be shared by several receptor pathways, or they may be exclusive to one receptor pathway, thus allowing distinct signaling pathways to be built up from a relatively limited number of components. The assembly of multisubunit signaling complexes involves specific interactions of a number of distinct types of proteininteraction domains, or protein-interaction modules, carried by the signaling proteins. Figure 7.2 gives a few examples of such domains. Signaling proteins in general contain at least one such protein-interaction domain, but many contain multiple domains. These protein modules cooperate with each other, for example, to organize signaling proteins into the correct subcellular localizations, to enable specific binding between protein partners, and to modify enzymatic activity. For the pathways considered in this chapter, the most important mechanism underlying the formation of signaling complexes is the phosphorylation of protein tyrosine residues. Phosphotyrosines are binding sites for a number of protein-interaction domains, including the SH2 (Src homology 2) domain (see Fig. 7.2). SH2 domains, built from approximately 100 amino acids, are present in many intracellular signaling proteins, where they are frequently linked to other types of enzymatic or other functional domains. SH2 domains recognize the phosphorylated tyrosine (pY) and, typically, the amino acid three positions away (pYXXZ, where X is any amino acid and Z is a specific amino acid); they bind in a sequence-specific fashion, with different SH2 domains preferring different combinations of amino acids. In this way, the unique SH2 domain of a signaling molecule can act as a ‘key’ that allows inducible and specific association with a protein containing the appropriate pY-containing amino acid sequence. Tyrosine kinase-associated receptors can assemble multiprotein signaling complexes by using proteins called scaffolds and adaptors. Scaffolds and adaptors lack enzymatic activity, and they function by recruiting other proteins into a signaling complex so that interactions among these proteins can take place. Fig. 7.2 Signaling proteins interact with each other and with lipid signaling molecules via modular protein domains. A few of the most common protein domains used by immune-system signaling proteins are listed, together with some proteins that contain them and the general class of ligand bound by the interaction domain. The right-hand column lists specific examples of a protein motif (in single-letter amino acid code) or, for the phosphoinositide-binding domains, the particular phosphoinositide that they bind. All these domains are used in many other nonimmune signaling pathways as well. Protein domain Found in Ligand class Example of ligand SH2 Lck, ZAP-70, Fyn, Src, Grb2, PLC-γ, STAT, Cbl, Btk, Itk, SHIP, Vav, SAP, PI3K phosphotyrosine pYXXZ SH3 Lck, Fyn, Src, Grb2, Btk, Itk, Tec, Fyb, Nck, Gads proline PXXP PH Tec, PLC-γ, Akt, Btk, Itk, Sos phosphoinositides PIP3 phosphoinositides PI(3)P PX phox P40 , P47phox, PLD PDZ CARMA1 C termini of proteins IESDV, VETDV C1 RasGRP, PKC-θ membrane lipid diacylglycerol (DAG) phorbol ester NZF TAB2 polyubiquitin (K63-linked) polyubiquitinated RIP, TRAF-6, or NEMO Immunobiology | chapter 7 | 07_002 Murphy et al | Ninth edition © Garland Science design by blink studio limited ERRNVPHGLFRVRUJ General principles of signal transduction and propagation. Scaffolds are relatively large proteins that can, for example, become tyrosine phosphorylated on multiple sites in order to recruit many different proteins (Fig. 7.3, top panel). By specifying which proteins are recruited, scaffolds can define the character of a particular signaling response. This is accomplished by several mechanisms. For example, scaffolds can regulate the specificity of a recruited enzyme by recruiting one of the enzyme’s substrates. Binding to a scaffold can also change the conformation of a recruited protein, thereby revealing sites for protein modifications, such as phosphorylation or ubiquitination, or for protein–protein interactions. Finally, scaffolds can function to promote membrane localization of the signaling complex. Adaptors are membrane-anchored or cytoplasmic proteins containing several signaling modules that serve to link two or more proteins together. The adaptor proteins Grb2 and Gads, for example, each contain an SH2 domain and two copies of another module called the SH3 domain (see Fig. 7.2). This arrangement of modules can be used to link tyrosine phosphorylation of a receptor to molecules acting in the next stage of signaling. For example, the SH2 domain of Grb2 binds to a phosphotyrosine residue on a receptor or a scaffold protein, while its two SH3 domains bind to proline-rich motifs on other signaling proteins (see Fig. 7.3, bottom panel), such as Sos, which we discuss in the next section. Activation of a protein kinase results in phosphorylation of a scaffold The phosphorylated scaffold recruits signaling proteins that bind to it Membrane localization Bring together enzymes and substrates Phosphorylationdependent recruitment (inducible and reversible) Promote conformational changes protein kinase The adapter Grb2 binds to the signaling protein Sos via its SH3 domains An activated erythropoietin (Epo) receptor becomes tyrosine phosphorylated Grb2 binds to the phosphotyrosine via its SH2 domain, bringing Sos to the receptor Epo Epo receptor kinase Grb2 SH3 SH2 SH3 Sos Immunobiology | chapter 7 | 07_100 Murphy et al | Ninth edition © Garland Science design by blink studio limited ERRNVPHGLFRVRUJ Fig. 7.3 Assembly of signaling complexes is mediated by scaffold and adaptor proteins. Assembly of signaling complexes is an important aspect of signal transduction. This is often achieved through scaffold and adaptor proteins. In general, scaffolds have numerous sites of phosphorylation that function to bring many different signaling proteins together (top panel). Scaffolds may also function to promote membrane localization, to bring enzymes into close proximity with their substrates, and to induce conformational changes in proteins that regulate their functions. An adaptor protein functions to bring two different proteins together (bottom panel). When erythropoietin (Epo) binds to its receptor, associated tyrosine kinases phosphorylate (red dots) sites on the Epo receptor cytoplasmic domain, generating binding sites for the SH2 domain of an adaptor protein. The adaptor protein (green) shown here contains two SH3 domains in addition to an SH2 domain. With the SH3 domains it can, for example, bind proline-rich sites on an intracellular signaling molecule (yellow). 261 262 Chapter 7: Lymphocyte Receptor Signaling In the resting state, small G proteins are bound to GDP and are inactive GTP GEF GDP:Ras Signaling activates guanine-nucleotide exchange factors (GEFs) such as Sos, which increase the rate of exchange of GDP for GTP The GTP-bound small G protein is the active effector molecule active Ras GTP:Ras Over time, the small G protein hydrolyzes the GTP to GDP and becomes inactive, a process that is accelerated by GAPs GDP:Ras Immunobiology | chapter 7 | 07_004are switched Fig. 7.4 Small G proteins Murphy et al | Ninth edition from inactive to active states by © Garland Science design by blink studio limited guanine-nucleotide exchange factors (GEFs) and the binding of GTP. Ras is a small GTP-binding protein with intrinsic GTPase activity. In its resting state, Ras is bound to GDP. Receptor signaling activates guanine-nucleotide exchange factors (GEFs), such as Sos, which can bind to small G proteins such as Ras and increase the rate of exchange of GDP for GTP (center panels). The GTP-bound form of Ras can then bind to a large number of effectors, recruiting them to the membrane. Over time, the intrinsic GTPase activity of Ras will result in the hydrolysis of GTP to GDP. GTPase-activating proteins (GAPs) can accelerate the hydrolysis of GTP to GDP, thus shutting off the signal more rapidly. 7-3 Small G proteins act as molecular switches in many different signaling pathways. Monomeric GTP-binding proteins known as small G proteins or small GTPases are important in the signaling pathways leading from many tyrosine kinase-associated receptors. The small GTPases are distinct from the larger heterotrimeric G proteins associated with G-protein-coupled receptors such as the chemokine receptors discussed in Chapter 3. The superfamily of small GTPases comprises more than 100 different proteins, and many are important in lymphocyte signaling. One of these, Ras, is involved in many pathways leading to cell proliferation. Other small GTPases include Rac, Rho, and Cdc42, which control changes in the actin cytoskeleton caused by signals received through the T-cell receptor or B-cell receptor. We will describe their actions in more detail in Section 7-19. Small GTPases exist in two states, depending on whether they are bound to GTP or to GDP. The GDP-bound form is inactive but is converted into the active form by exchange of the GDP for GTP. This reaction is mediated by proteins known as guanine-nucleotide exchange factors, or GEFs, which cause the GTPase to release GDP and to bind the more abundant GTP (Fig. 7.4). Sos, which is recruited to signaling pathways by the adaptor Grb2 (see Section 7-2), is one of the GEFs for Ras. The binding of GTP induces a conformational change in the small GTPase that enables it to bind to and induce effector activity in a variety of target proteins. Thus, GTP binding functions as an on/off switch for small GTPases. This GTP-bound form does not remain permanently active but is eventually converted into the inactive GDP-bound form by the intrinsic GTPase activity in the G protein, which removes a phosphate group from the bound GTP. Regulatory cofactors known as GTPase-activating proteins (GAPs) accelerate the conversion of GTP to GDP, thus rapidly downregulating the activity of the small GTPase. Because of GAP activity, small GTPases are usually present in the inactive GDP-bound state and are activated only transiently in response to a signal from an activated receptor. RAS is frequently mutated in cancer cells, and the mutated Ras protein is thought to be a significant contributor to the cancerous state. The importance of GAPs in signaling regulation is indicated by the fact that some mutations in Ras found in cancer act by preventing GAP from enhancing the intrinsic GTPase activity of Ras, thus prolonging the duration for which Ras exists in the active GTP-bound state. GEFs are the key to G-protein activation and are recruited to the site of receptor activation at the cell membrane by binding to adaptor proteins or to lipid metabolites produced by receptor activation. Once recruited, they are able to activate Ras or other small G proteins, which are localized to the inner surface of the plasma membrane via fatty acids that are attached to the G protein post-translationally. Thus, G proteins act as molecular switches, becoming switched on when a cell-surface receptor is activated and then being switched off. Each G protein has its own specific GEFs and GAPs, which help to confer specificity on the pathway. 7-4 Signaling proteins are recruited to the membrane by a variety of mechanisms. We have seen how receptors can recruit intracellular signaling proteins to the plasma membrane through tyrosine phosphorylation of the receptor itself or of an associated scaffold, followed by recruitment of SH2-domain-containing signaling proteins or adaptors, such as Grb2 (Fig. 7.5). A second mechanism for membrane recruitment of signaling proteins is via binding to small GTPases, such as Ras, following their activation. As described in Section 7-3, small GTPases are constitutively bound to the cytoplasmic surface of the plasma ERRNVPHGLFRVRUJ General principles of signal transduction and propagation. Binding to phosphorylated sites on a membrane-associated protein Recognition of activated small G proteins PI 3-kinase phosphorylates PIP2 to generate PIP3 Binding to membrane lipids LAT PH domain PLC-γ Ras (inactive) Gads Ras (active) PI 3kinase Grb2 Immunobiology | chapter 7 | 07_005 Murphy et al | Ninth edition membrane due to their fatty acid modifications. Once activated by exchange of GDP for GTP, the activated GTPases bind to signaling proteins such as Sos, relocalizing the bound proteins to the plasma membrane (see Fig. 7.5). © Garland Science design by blink studio limited Another way in which receptors can recruit signaling molecules to the plasma membrane is by the local production of modified membrane lipids. These lipids are produced by phosphorylation of the membrane phospholipid phosphatidylinositol by enzymes known as phosphatidylinositol kinases, which are activated as a result of receptor signaling. The inositol headgroup of phosphatidylinositol is a carbohydrate ring that can be phosphorylated at one or more positions to generate a wide variety of derivatives. The ones that we will focus on in this chapter are phosphatidylinositol 4,5-bisphosphate (PIP2) and phosphatidylinositol 3,4,5-trisphosphate (PIP3), the latter of which is generated from PIP2 by the enzyme phosphatidylinositol 3-kinase (PI 3-kinase) (see Fig. 7.5). PI 3-kinase is often recruited by binding of the SH2 domain of its regulatory subunit to phosphotyrosines in a receptor tail, bringing its catalytic subunit into proximity with inositol phospholipid substrates in the membrane. In this way, membrane phosphoinositides such as PIP3 are rapidly produced after receptor activation. This, combined with their short life span, makes them ideal signaling molecules. PIP3 is recognized specifically by proteins containing a pleckstrin homology (PH) domain or, less commonly, a PX domain (see Fig. 7.2), and one of its functions is to recruit such proteins to the membrane and in some cases contribute to enzyme activation. 7-5 Post-translational modifications of proteins can both activate and inhibit signaling responses. Protein phosphorylation is a common mechanism for transducing signals from cellular receptors to downstream pathways. These signals are terminated by the action of protein phosphatases, which dephosphorylate signaling intermediates (Fig. 7.6). The importance of protein phosphatases in terminating signaling is underscored by the existence of diseases, such as autoimmunity and cancer, which may result from absent or deficient protein phosphatase activity. However, protein dephosphorylation can also function as a mechanism of activation. Dephosphorylation can regulate protein–protein interactions, protein subcellular localization, or nucleic acid binding, thereby promoting downstream signaling events. Another general mechanism of protein regulation by post-translational modification is the covalent attachment of one or more molecules of the small protein ubiquitin. Ubiquitination is a potent means of signal termination, as it often leads to protein degradation. Ubiquitin is attached by its carboxyterminal glycine to lysine residues of target proteins in a multi-step process. ERRNVPHGLFRVRUJ Akt Itk Fig. 7.5 Signaling proteins can be recruited to the membrane in a variety of ways. Recruitment of signaling proteins to the plasma membrane is important in signal propagation because this is where receptors are usually located. Left panel: tyrosine phosphorylation of membraneassociated proteins, such as the scaffold LAT, recruits phosphotyrosine-binding proteins. This can also protect the scaffold from dephosphorylation by tyrosine phosphatases, which inhibit signaling. Second panel: small G proteins such as Ras can associate with the membrane by having lipid modifications (shown in red). When activated, they can bind a variety of signaling proteins. Right two panels: modifications to the membrane itself that result from receptor activation can recruit signaling proteins. In this example the membrane lipid PIP3 has been produced in the inner leaflet of the plasma membrane by the phosphorylation of PIP2 by PI 3-kinase. PIP3 is recognized by the PH domains of signaling proteins such as the protein kinases Akt and Itk. 263 264 Chapter 7: Lymphocyte Receptor Signaling Fig. 7.6 Signaling must be turned off as well as turned on. The inability to terminate a signaling pathway can result in serious diseases such as autoimmunity or cancer. As a significant proportion of signaling events depend on protein phosphorylation, protein phosphatases, such as SHP, have an important part in shutting down signaling pathways (left panel). Another common mechanism for terminating signaling is regulated protein degradation (center and right panels). Phosphorylated proteins recruit ubiquitin ligases, such as Cbl, that add the small protein ubiquitin to proteins, thus targeting them for degradation. Cytoplasmic proteins are targeted for destruction in the proteasome by the addition of polyubiquitin chains, linked through lysine 48 (K48) of ubiquitin (center panel). Membrane receptors that become ubiquitinated by individual ubiquitin molecules or di-ubiquitin are internalized and transported to the lysosome for destruction (right panel). Dephosphorylation of phosphorylated substrates Ubiquitin-mediated degradation by proteasome Ubiquitin-mediated degradation in the lysosome Cbl Cbl K48 polyubiquitin lysosome SHP proteasome Immunobiology | chapter 7 | 07_006 Murphy et al | Ninth edition First, an E1 ubiquitin-activating enzyme promotes attachment of ubiquitin to an E2 ubiquitin-conjugating enzyme. The ubiquitin is then transferred to the protein substrate by an enzyme known as an E3 ubiquitin ligase. Ubiquitin ligases can continue to add ubiquitin molecules to form polyubiquitin. Importantly, different ubiquitin ligases add the carboxy terminus of one ubiquitin molecule to different lysine residues of the conjugated ubiquitin, typically either lysine 48 (K48) or lysine 63 (K63). These different forms of polyubiquitin produce divergent outcomes for signaling pathways. © Garland Science design by blink studio limited When polyubiquitin chains are formed using K48 linkages, the outcome is to target the protein for degradation by the proteasome (see Fig 7.6). An important ubiquitin ligase of this kind in lymphocytes is Cbl, which selects its targets via its SH2 domain. Cbl can thus bind to specific tyrosine-phosphorylated targets, causing them to become ubiquitinated via K48 linkages. Proteins that recognize this form of polyubiquitin then target the ubiquitinated proteins to degradative pathways via the proteasome. Membrane proteins such as receptors can be tagged by single ubiquitin molecules or by di-ubiquitin. These are not recognized by the proteasome, but instead, are recognized by specific ubiquitin-binding proteins that target proteins for degradation in lysosomes (see Fig. 7.6). Thus, ubiquitination of proteins can inhibit signaling. Unlike phosphatases, where the mechanism of inhibition is reversible, inhibition by ubiquitin-mediated protein degradation is a more permanent means of terminating signaling. Ubiquitination can also be used to activate signaling pathways. We have already discussed this aspect in Section 3-7 in connection with the NFκB signaling pathway from TLRs. There, the ubiquitin ligase TRAF-6 produces K63-linked polyubiquitin chains on TRAF-6 and NEMO. In lymphocytes, K63‑linked polyubiquitination is a key step in signaling through tumor necrosis factor (TNF) receptor family members, as will be discussed in Section 7-23 (and Fig. 7.31). This form of polyubiquitin is recognized by specific domains in signaling proteins that recruit additional signaling molecules to the pathway (see Fig. 3.15). 7-6 The activation of some receptors generates small-molecule second messengers. In many cases, intracellular signaling involves the activation of enzymes that produce small-molecule biochemical mediators known as second messengers (Fig. 7.7). These mediators can diffuse throughout the cell, enabling the ERRNVPHGLFRVRUJ Antigen receptor signaling and lymphocyte activation. Signaling results in the release of the second messenger calcium Amplification by kinase cascades Raf calmodulin receptor Mek IP3 Erk Calcium rapidly diffuses throughout the cell and induces conformational changes in calmodulin effector protein Ca2+ ER Immunobiology | chapter 7 | 07_007 Murphy et al | Ninth edition signal to activate a studio variety design by blink limited of target proteins. The enzymatic production of sec© Garland Science ond messengers also serves the dual purpose of achieving concentrations of them sufficient to activate the next stage of the pathway and of amplifying the signaling cascade. The second messengers generated by receptors that signal via tyrosine kinases include calcium ions (Ca2+) and a variety of membrane lipids and their soluble derivatives. Although some of these lipid messengers are confined to membranes, they can move within them. A second messenger binding to its target protein typically induces a conformational change that allows the protein to be activated. Summary. Cell-surface receptors serve as the front line of a cell’s interaction with its environment; they sense extracellular events and convert them into biochemical signals for the cell. As most receptors sit in the plasma membrane, a critical step in the transduction of extracellular signals to the interior of the cell is recruitment of intracellular proteins to the membrane and changes in the composition of the membrane surrounding the receptor. Many immune receptors operate by activating tyrosine kinases to transmit their signals onward, often using scaffolds and adaptors to form large multiprotein signaling complexes. Both the qualitative and quantitative changes that take place in the composition of these signaling complexes determine the character of the response and biological outcomes. Formation of signaling complexes is mediated by a wide variety of protein-interaction domains, or modules, including the SH2, SH3, and PH domains found in proteins. In many cases, the increase in enzymatically produced small-molecule signaling intermediates called second messengers regulates and amplifies the signaling cascade. Termination of signaling involves protein dephosphorylation as well as ubiquitin-mediated protein degradation. Antigen receptor signaling and lymphocyte activation. The ability of T cells and B cells to recognize and respond to their specific antigen is central to adaptive immunity. As described in Chapters 4 and 5, the B-cell antigen receptor (BCR) and the T-cell antigen receptor (TCR) are made up of antigen-binding chains—the heavy and light immunoglobulin chains ERRNVPHGLFRVRUJ Fig. 7.7 Signaling pathways amplify the initial signal. Amplification of the initial signal is an important element of most signal transduction pathways. One means of amplification is a kinase cascade (left panel), in which protein kinases successively phosphorylate and activate each other. In this example, taken from a commonly used kinase cascade (see Fig. 7.19), activation of the kinase Raf results in the phosphorylation and activation of a second kinase, Mek, that phosphorylates yet another kinase, Erk. As each kinase can phosphorylate many different substrate molecules, the signal is amplified at each step, resulting in a huge amplification of the initial signal. Another method of signal amplification is the generation of second messengers (center and right panels). In this example, signaling results in the release of the second messenger calcium (Ca2+) from intracellular stores into the cytosol or its influx from the extracellular environment. Ca2+ release from the endoplasmic reticulum (ER) is shown here. The sharp increase in free Ca2+ in the cytoplasm can potentially activate many downstream signaling molecules, such as the calcium-binding protein calmodulin. Calcium binding induces a conformational change in calmodulin, which allows it to bind to and regulate a variety of effector proteins. 265 266 Chapter 7: Lymphocyte Receptor Signaling T-cell receptor complex TCR recognition CD3 CD3 α ε β δ γ ε in the B-cell receptor, and the TCRα and TCRβ chains in the T-cell receptor. These variable chains have exquisite specificity for antigen, allowing each lymphocyte to detect the presence of one type of pathogen. However, binding of antigen to the antigen receptor is not sufficient for a lymphocyte to respond— the information that antigen receptor engagement has occurred also needs to be transduced into the intracellular compartment of the lymphocyte. Thus, the fully functional antigen receptor complex must include proteins that can transduce a signal across the plasma membrane. For the B-cell antigen receptor and the T-cell antigen receptor, this function is mediated by invariant accessory proteins that initiate signaling when the receptors bind antigen. Assembly with these accessory proteins is also essential for transport of the receptor to the cell surface. In this part of the chapter we describe the structure of the antigen receptor complexes on T cells and B cells, and the signaling pathways that lead from them. 7-7 ITAMs ζ ζ signaling Immunobiology | chapter 7 | 07_008 Fig. 7.8 Murphy et alThe | NinthT-cell edition receptor complex Science by blink studio limited © isGarland made up design of variable antigen- recognition proteins and invariant signaling proteins. Upper panel: the functional T-cell receptor (TCR) complex is composed of the antigen-binding TCRα:β heterodimer associated with six signaling chains: two ε, one δ, and one γ collectively called CD3, and a homodimer of ζ. Cellsurface expression of the antigen-binding chains requires assembly of TCRα:β with the signaling subunits. Each CD3 chain has one immunoreceptor tyrosine-based activation motif (ITAM), shown as a yellow segment, whereas each ζ chain has three. The transmembrane regions of each chain have unusual acidic or basic residues as shown. Lower panel: the transmembrane regions of the various TCR subunits are represented in cross-section. It is thought that one of the positive charges, from a lysine (K) of the α chain, interacts with the two negative charges of aspartic acid (D) of the CD3δ:ε dimer, while the other positive charge, of arginine (R), interacts with aspartic acids of the ζ homodimer. The positive arginine (K) charge of the β chain interacts with the negative charges of aspartic acid and glutamic acid (E) in the CD3γ:ε dimer. Antigen receptors consist of variable antigen-binding chains associated with invariant chains that carry out the signaling function of the receptor. In T cells, the highly variable TCRα:β heterodimer (see Chapter 5) is not sufficient on its own to make up a complete cell-surface antigen receptor. When cells were transfected with cDNAs encoding the TCRα and TCRβ chains, the heterodimers formed were degraded and did not appear on the cell surface. This implied that other molecules are required for the T-cell receptor to be expressed on the cell surface. In the T-cell receptor, the other required mole cules are the CD3γ, CD3δ, and CD3ε chains, which together form the CD3 complex; and the ζ chain, which is present as a disulfide-linked homodimer (Fig. 7.8). The CD3 proteins have an extracellular immunoglobulin-like domain, whereas the ζ chain contains only a short extracellular domain. Throughout the remainder of the chapter, we will use the term T-cell receptor to refer to the entire T-cell receptor complex, including these associated signaling subunits. Although the exact stoichiometry of the complete T-cell receptor is not definitively established, it is thought that the receptor α chain interacts with one CD3δ:CD3ε dimer and one ζ dimer, while the receptor β chain interacts with one CD3γ:CD3ε dimer (see Fig. 7.8). These interactions are mediated by reciprocal charge interactions between basic and acidic intramembrane amino acids of the receptor subunits. There are two positive charges in the TCRα transmembrane region and one in the TCRβ transmembrane domain. Negative charges in the CD3 and ζ transmembrane domains interact with the positive charges in α and β. Assembly of CD3 and a ζ dimer with the α:β heterodimer stabilizes the α and β dimer during its production in the endoplasmic reticulum and allows the complex to be transported to the plasma membrane. These associations ensure that all T-cell receptors present on the plasma membrane are properly assembled. Signaling from the T-cell receptor is initiated by tyrosine phosphorylation within cytoplasmic regions called immunoreceptor tyrosine-based activation motifs (ITAMs) in the CD3ε, γ, δ, and ζ chains. CD3γ, δ, and ε each contain one ITAM, and each ζ chain contains three, giving the T-cell receptor a total of 10 ITAMs. This motif is also present in the signaling chains of the B-cell receptor and in the NK-cell receptors described in Chapter 3, as well as in the receptors for the immunoglobulin constant region (Fc receptors) that are present on mast cells, macrophages, monocytes, neutrophils, and NK cells (see Section 7-11 below). Each ITAM contains two tyrosine residues that become phosphorylated by specific protein tyrosine kinases when the receptor binds its ligand, providing ERRNVPHGLFRVRUJ Antigen receptor signaling and lymphocyte activation. Autoinhibited conformation of ZAP-70 extracellular Phosphorylation of the ITAM recruits ZAP-70, which is then activated by phosphorylation Receptor SH2 domain kinase domain SH2 domain ITAM ZAP-70 9–12 Y Y cytoplasm Immunobiology | chapter 7 | 07_009 Murphy et al | Ninth edition sites thedesign recruitment of the SH2 domains of signaling proteins as described Science by blink studio limited © Garlandfor earlier in the chapter. Two YXXL/I motifs are separated by about six to nine amino acids within each ITAM, so the canonical ITAM sequence is …YXX[L/I] X6–9YXX[L/I]…, where Y is tyrosine, L is leucine, I is isoleucine, and X represents any amino acid. The two tyrosines of the ITAM make it particularly efficient in recruiting signaling proteins that contain two tandem SH2 domains (Fig. 7.9). When both tyrosines of the ITAM are phosphorylated, tandem SH2 domain-containing proteins such as Syk or ZAP-70 are recruited. This leads to the phosphorylation of Syk or ZAP-70, an essential step in the activation of these kinases, as will be discussed further below (see Section 7-10). The antigen-binding immunoglobulin on the B-cell surface is also associated with invariant protein chains that mediate signaling. These two polypeptides, called Igα and Igβ, are required for transport of the receptor to the surface and for B-cell receptor signaling (Fig. 7.10). Igα and Igβ are single-chain proteins composed of an extracellular immunoglobulin-like domain connected by a transmembrane domain to a cytoplasmic tail. They form a disulfide-linked heterodimer that becomes associated with immunoglobulin heavy chains and enables their transport to the cell surface. The Igα:Igβ dimer associates with the B-cell receptor through hydrophilic rather than charge interactions between their transmembrane regions. The complete B-cell receptor is thought to be a complex of six chains—two identical light chains, two identical heavy chains, and one associated heterodimer of Igα and Igβ. Like CD3 and the ζ chains of the T-cell receptor, Igα and Igβ have ITAMs, but just one each, and these are essential for the ability of the B-cell receptor to signal. 7-8 Fig. 7.9 ITAMs recruit signaling proteins that have tandem SH2 domains. The ITAMs of the T-cell receptor (TCR) and B-cell receptor (BCR) contain tyrosine residues within the motif`…YXX[L/I] X6–9YXX[L/I]…. The spacing between the tyrosines is important in binding to tandem SH2-containing proteins such as Syk and ZAP-70. Left panel: prior to TCR or BCR stimulation, these kinases are in an inactive conformation, known as the autoinhibited conformation. The autoinhibited conformation is stabilized by interactions between the tandem SH2 domain-kinase domain linker region and the kinase domain that hold the enzyme in a catalytically inactive state. Right panel: after phosphorylation of both tyrosines within one ITAM (here depicted as two tyrosines separated by 9-12 amino acids), the tandem SH2 domains of Syk or ZAP-70 can dock cooperatively to both phosphotyrosines, as shown here for ZAP-70. By being recruited into the active signaling complex, ZAP-70 can itself be phosphorylated, so that it becomes an active kinase that can then phosphorylate its substrates. This final step of ZAP-70 activation requires phosphorylation on two tyrosines in the linker region between the tandem SH2 domains and the kinase domain, together with phosphorylation of a tyrosine in the catalytic site of the kinase domain. B-cell receptor complex Antigen recognition by the T-cell receptor and its co-receptors transduces a signal across the plasma membrane to initiate signaling. To make an effective immune response, T cells and B cells must be able to respond to their specific antigen even when it is present at extremely low levels. This is especially important for T cells, as an antigen-presenting cell will display on its surface many different peptides from both self and foreign proteins, and the number of peptide:MHC complexes specific for a particular T-cell receptor is likely to be very low. Some estimates suggest that a naive CD4 T Fig. 7.10 The B-cell receptor complex is made up of cell-surface immunoglobulin with one each of the invariant signaling proteins Igα and Igβ. The immunoglobulin recognizes and binds antigen but cannot itself generate a signal. It is associated with antigen-nonspecific signaling molecules—Igα and Igβ. These each have a single ITAM (yellow segment) in their cytosolic tails that enables them to signal when the B-cell receptor is ligated with antigen. Igα and Igβ form a disulfide-linked heterodimer that is non-covalently associated with the heavy chains. recognition light chain heavy chain Igβ Igα ITAM signaling Immunobiology | chapter 7 | 07_010 Murphy et al | Ninth edition ERRNVPHGLFRVRUJ © Garland Science design by blink studio limited 267 268 Chapter 7: Lymphocyte Receptor Signaling Lck phosphorylates the ITAMs in the TCR upon co-receptor engagement with antigen:MHC antigen-presenting cell CD4 MHC class II TCR Lck T cell ZAP-70 is recruited by tandem SH2 domains to the ITAMs and is phosphorylated by Lck ZAP-70 ZAP-70 Immunobiology | chapter 7 | 07_011 Fig. 7.11 Engagement of co-receptors Murphy et al | Ninth edition with the T-cell receptor enhances phosphorylation of the ITAMs. Upper panel: for simplicity, we show the CD4 co-receptor engaging the same MHC molecule as the T-cell receptor (TCR), although signaling within receptor microclusters may differ from this arrangement. When T-cell receptors and co-receptors are brought together by binding to peptide:MHC complexes on the surface of an antigen-presenting cell, recruitment of the co-receptor-associated kinase Lck leads to phosphorylation of ITAMs in CD3γ, δ, and ε, and in the ζ chains. Lower panel: the tyrosine kinase ZAP-70 binds to phosphorylated ITAMs through its SH2 domains, enabling ZAP-70 to be phosphorylated and activated by Lck. ZAP‑70 then phosphorylates other intracellular signaling molecules. © Garland Science design by blink studio limited cell can become activated by fewer than 50 antigenic peptide:MHC complexes displayed by an antigen-presenting cell, and effector CD8 cytotoxic T cells may be even more sensitive. B cells become activated when about 20 B-cell receptors are engaged. These estimates based on in vitro studies may not be precise for cells in vivo, but it is clear that the antigen receptors on T cells and B cells confer remarkable sensitivity to antigen. For a peptide:MHC complex to activate T cells, it must bind directly to the T-cell receptor (Fig. 7.11, upper panel, and see Fig. 4.22). However, it remains unclear precisely how this extracellular recognition event is transmitted across the T-cell membrane to initiate signaling. Questions remain as to the stoichiometry and physical arrangement of T-cell receptors and peptide:MHC complexes required to initiate the signaling cascade. We will discuss this area of active research only briefly before moving on to explain well-understood intracellular events that occur after antigen recognition. One suggestion is that signaling is initiated by T-cell receptor dimerization through formation of ‘pseudo-dimeric’ peptide:MHC complexes containing one antigen peptide:MHC molecule and one self peptide:MHC molecule on the surface of the antigen-presenting cell. This model relies on a weak interaction occurring between the T-cell receptor and self peptide:MHC complexes, stabilized by the CD4 or CD8 co-receptor interactions with the self peptide:MHC complexes, but it would explain signaling induced by low densities of antigenic peptides. An additional possibility is that the antigenic peptide:MHC complex induces a conformational change in the T-cell receptor, or its associated CD3 and ζ chains, that promotes receptor phosphorylation; however, direct structural evidence supporting this model is still lacking. It has also been suggested that signaling might involve receptor oligomerization, or clustering, as antibodies that bind to and cross-link T-cell receptors can activate T cells. Since antigenic peptides are vastly outnumbered by other peptides displayed on the surface of the antigen-presenting cell, it is unlikely that physiologic amounts of antigen induce conventional oligomerization as observed with antibodies. However, assemblies of small numbers of T-cell receptors called microclusters have been observed in the zone of contact between the T cell and the antigen-presenting cell. These microclusters form rapidly following TCR stimulation, and quickly merge with microclusters containing downstream signaling components, such as scaffolds and adaptors. Current evidence indicates that signaling is initiated in these microclusters. One popular model proposes that signal initiation takes place when inhibitory signaling proteins are excluded from these complexes. A key component of this model is that prior to TCR signaling, activating and inhibitory enzymes are in a balanced equilibrium; the initiation of signaling occurs when this equilibrium is perturbed in favor of the activating modifications. 7-9 Antigen recognition by the T-cell receptor and its co-receptors leads to phosphorylation of ITAMs by Src-family kinases, generating the first intracellular signal in a signaling cascade. The first intracellular signal generated after the T cell has detected its specific antigen is the phosphorylation of both tyrosines in the ITAMs of the T-cell receptor. This signal is initiated with the help of the CD4 and CD8 coreceptors, which bind to MHC class II molecules and class I molecules, respectively, via their extracellular domains (see Section 4-18), and associate with nonreceptor kinases via their intracellular domains. The Src-family kinase Lck is constitutively associated with the cytoplasmic domains of CD4 and CD8 and is thought to be the kinase primarily responsible for phosphorylation of the ITAMs of the T-cell receptor (see Fig. 7.11). Evidence suggests that binding of the co-receptor to the peptide:MHC complex that binds the T-cell receptor ERRNVPHGLFRVRUJ Antigen receptor signaling and lymphocyte activation. enhances the recruitment of Lck to the engaged T-cell receptor, leading to more efficient phosphorylation of the T-cell receptor ITAMs. The importance of this event is demonstrated by the profound reduction in T-cell development in Lck-deficient mice. This indicates the essential role of Lck in T-cell receptor signaling during the selection of developing T cells in the thymus (discussed in Chapter 8). Lck is important for T-cell receptor signaling in naive T cells and effector T cells, but is less important for the activation or maintenance of memory CD8 T cells by their specific antigen. A related tyrosine kinase, Fyn, is weakly associated with the ITAMs of the T-cell receptor and may have some role in signaling. Whereas mice lacking Fyn develop normal CD4 and CD8 T cells that respond in essentially normal fashion to antigen, mice lacking both Lck and Fyn show a more complete loss of T-cell development than mice lacking Lck alone. Another role of the co-receptors in T-cell receptor signaling may be to stabilize interactions between the receptor and the peptide:MHC complex. Affinities of individual receptors for their specific peptide:MHC complexes are in the micromolar range, which means that the T-cell receptor:peptide:MHC complexes have half-lives of less than 1 second and dissociate rapidly. The additional binding of a co-receptor to the MHC molecule is thought to stabilize the interaction by increasing its duration, thereby providing time for an intracellular signal to be generated. The Lck bound to the cytoplasmic tails of CD4 or CD8 is brought near its substrate ITAMs in the T-cell receptor when the co-receptor binds the receptor:peptide:MHC complex (see Fig. 7.11). Lck’s activity is also regulated allosterically by phosphorylation of a tyrosine in its carboxy terminus by the C-terminal Src kinase (Csk). The resulting phosphotyrosine interacts with Lck’s SH2 domain and helps maintain Lck in a closed conformation, resulting in a catalytically inactive state (Fig. 7.12). The absence of Csk during T-cell development causes T cells to mature autonomously in the thymus without needing to bind peptide:MHC, presumably as a result of abnormal activation of TCR signaling by hyperactive Lck in Csk-deficient thymocytes. This suggests that Csk normally acts to reduce Lck activity and to attenuate TCR signaling. Dephosphorylation of the tyrosine or engagement of the SH2 or SH3 domains by their ligands releases Lck from its inactive conformation, resulting in a primed, but not fully active, Lck kinase (see Fig. 7.12). Full activation of catalytic activity also requires Lck autophosphorylation of a tyrosine in its kinase domain. In nonstimulated lymphocytes, phosphorylation of Lck is counteracted by the tyrosine phosphatase CD45, which can dephosphorylate both of the Lck tyrosine phosphorylation sites. Prior to TCR stimulation, multiple phosphorylated species of Lck are found in T cells, but antigen receptor stimulation is required to stabilize the activated form of Lck and lead to ITAM phosphorylation. Fig. 7.12 Lck activity is regulated by tyrosine phosphorylation and dephosphorylation. Src kinases such as Lck contain SH3 (blue) and SH2 (orange) domains preceding the kinase domain (green). Lck also contains a unique amino-terminal motif (yellow) with two cysteine residues that bind a Zn ion that is also bound to a similar motif in the cytoplasmic domain of CD4 or CD8. Upper panel: in inactive Lck, the two lobes of the kinase domain are constrained by interactions with both the SH2 and SH3 domains. The SH2 domain interacts with a phosphorylated tyrosine (red) at the carboxy-terminal end of the kinase domain. The SH3 domain interacts with a proline-rich sequence in the linker between the SH2 domain and the kinase domain. Middle panel: dephosphorylation of the carboxyterminal tyrosine by the phosphatase CD45 (not shown) releases the SH2 domain. Binding of other ligands to the SH3 region can facilitate release of the linker region (not shown). In this state, Lck is considered primed, but not fully activated. Lower panel: full activation of Lck catalytic activity requires autophosphorylation on the activation loop in the kinase domain. Active Lck can then phosphorylate ITAMs in the signaling chains of the nearby T-cell receptor. Rephosphorylation of the carboxy-terminal tyrosine by the C-terminal Src kinase (Csk) or loss of the SH3 ligand returns Lck to the inactive state. Lck is inactive when its terminal tyrosine is phosphorylated and binds the SH2 domain and the linker region binds the SH3 domain CD4/CD8 Cys Zn Cys Cys Cys SH3 kinase domain SH2 inactive Lck CD45 Csk Lck is primed when its terminal tyrosine is dephosphorylated and its SH3 domain releases the linker region Cys Zn Cys Cys Cys Y primed Lck Lck is fully activated when its activation loop tyrosine in the kinase domain is autophosphorylated Cys Cys Zn Cys Cys Y active Lck Immunobiology | chapter 7 | 07_012 Murphy et al | Ninth edition ERRNVPHGLFRVRUJ © Garland Science design by blink studio limited ζ ζ 269 270 Chapter 7: Lymphocyte Receptor Signaling 7-10 Phosphorylated ITAMs recruit and activate the tyrosine kinase ZAP-70. Structure of autoinhibited ZAP-70 Y315 Y319 SH2 SH2 kinase Immunobiology | chapter 7of | 07_110 Fig. 7.13 Structure the autoinhibited Murphy et al | Ninth edition ZAP-70 kinase. The structure of the © Garland Science design by blink studio limited inactive autoinhibited conformation of ZAP-70 is shown with the protein domains color-coded according to the domain map shown at the bottom; the dashed red line indicates a region of the protein that was not detected in the structural analysis. Prior to T-cell receptor stimulation, the ZAP-70 kinase is in this inactive conformation, based on interactions between the tandem SH2 domain-kinase domain linker region (thick red line) and the kinase domain. This interaction stabilizes ZAP-70 in a catalytically inactive state, referred to as the autoinhibited form of ZAP-70, by locking the kinase domain in an inactive conformation. Following T-cell receptor stimulation, Lck phosphorylates two tyrosine residues in this linker region, Y315 and Y319, shown in yellow. Lck also phosphorylates a tyrosine residue in the catalytic (kinase) domain. When Y315 and Y319 are phosphorylated, the linker region no longer binds to the kinase domain, allowing the phosphorylated kinase domain to adopt an active conformation. Courtesy of Arthur Weiss. Receptors other than antigen receptors also associate with ITAM-containing chains that deliver activating signals NK cells Macrophages Neutrophils NK cells FcγRIII (CD16) FcγRIV NKG2C, D, E (CD94) Mast cells Basophils FcεRI α γ or ζ DAP12 γ β The precise spacing of the two YXXL/I motifs in an ITAM suggests that the ITAM is a binding site for a signaling protein with two SH2 domains. In the case of the T-cell receptor, this protein is the tyrosine kinase ZAP-70 (ζ-chainassociated protein), which carries the activation signal onward. ZAP-70 has two tandem SH2 domains that can be simultaneously engaged by the two phosphorylated tyrosines in an ITAM (see Fig. 7.9). The affinity of the phosphorylated YXXL sequence for a single SH2 domain is low; binding of both SH2 domains to the ITAM is significantly stronger and confers specificity on ZAP-70 binding. Thus, when Lck has sufficiently phosphorylated an ITAM in the T-cell receptor, ZAP-70 binds to it. Once bound, ZAP-70 is phosphorylated by Lck at three tyrosine residues, two in the linker region between the tandem SH2 domains and the kinase domain, and a third residue in the catalytic domain. Together these phosphorylations activate ZAP-70 by disrupting the autoinhibited form of inactive ZAP-70, allowing the ZAP-70 kinase domain to adopt the active conformation (Fig. 7.13). ZAP-70 can also be activated by autophosphorylation. 7-11 ITAMs are also found in other receptors on leukocytes that signal for cell activation. The signaling subunits of the T-cell receptor and the B-cell receptor each contain ITAMs, which are essential for T-cell receptor and B-cell receptor signaling. Phosphorylation of both tyrosines in the ITAM functions to recruit a tyrosine kinase with tandem SH2 domains—ZAP-70 in the case of T cells, and a closely related kinase, Syk, in B cells. Other immune-system receptors also use ITAM-containing accessory chains to transduce activating signals (Fig. 7.14). One example is FcγRIII (CD16); this is a receptor for IgG that triggers antibody-dependent cell-mediated cytotoxicity (ADCC) by NK cells, which we consider in Chapter 11; CD16 is also found on macrophages and neutrophils, where it facilitates the uptake and destruction of antibody-bound pathogens. To signal, FcγRIII must associate either with the ζ chain found also in the T-cell receptor or with another member of the same protein family known as the Fcγ chain. The Fcγ chain is also the signaling component of another Fc receptor—the Fcε receptor I (FcεRI) on mast cells. As we discuss in Chapter 14, this receptor binds IgE antibodies, and on cross-linking by allergens, it triggers the degranulation of mast cells. Last, many activating receptors on NK cells are associated with DAP12, another ITAM-containing protein (see Section 3-26). Each of these additional ITAM-containing signaling chains becomes tyrosine phosphorylated following stimulation of its associated receptor, leading to the recruitment of a tyrosine kinase, either Syk or ZAP-70. With the exception of Fig. 7.14 Other receptors that pair with ITAM-containing chains can deliver activating signals. Cells other than B and T cells have receptors that pair with accessory chains containing ITAMs, which are phosphorylated when the receptor is cross-linked. These receptors deliver activating signals. The Fcγ receptor III (FcγRIII, or CD16) is found on NK cells, macrophages, and neutrophils. Binding of IgG to this receptor activates the killing function of the NK cell, leading to the process known