Chapter 7 Nerve Cells and Electrical Signaling PDF
Document Details
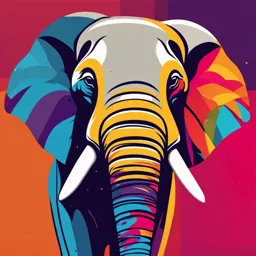
Uploaded by AmazedElectricOrgan
Tags
Summary
This document is a chapter from a PowerPoint lecture presentation about nerve cells and electrical signaling. It covers various aspects of the nervous system, including cells of the nervous system, the resting membrane potential, electrical signaling, and maintaining neural stability.
Full Transcript
PowerPoint® Lecture Presentation CHAPTER 7 Nerve Cells and Electrical Signaling © 2017 Pearson Education, Inc. Chapter Outline 7.1 7.2 7.3 7.4 7.5 Overview of the Nervous System Cells of the Nervous System Establishment of the Resting Membrane Potential Electrical Signaling Through Changes in Membra...
PowerPoint® Lecture Presentation CHAPTER 7 Nerve Cells and Electrical Signaling © 2017 Pearson Education, Inc. Chapter Outline 7.1 7.2 7.3 7.4 7.5 Overview of the Nervous System Cells of the Nervous System Establishment of the Resting Membrane Potential Electrical Signaling Through Changes in Membrane Potential Maintaining Neural Stability © 2017 Pearson Education, Inc. 7.1 Overview of the Nervous System Central nervous system (CNS) Brain Spinal cord Peripheral nervous system (PNS) Afferent information (input) Efferent information (output) © 2017 Pearson Education, Inc. Figure 7.1 Organization of the nervous system. Central Nervous System Peripheral Nervous System Somatic senses INPUT Afferent Special senses Visceral senses Brain and spinal cord Somatic OUTPUT Skeletal muscle Efferent Sympathetic Autonomic Parasympathetic © 2017 Pearson Education, Inc. Cardiac muscle Smooth muscle Glands Enteric nervous system Gastrointestinal tract 7.2 Cells of the Nervous System Neurons Excitable cells Glial cells Support cells © 2017 Pearson Education, Inc. Components of a Neuron Soma Contains nucleus and most organelles Dendrites Reception of incoming information Axon Transmits electrical impulses called action potentials Axon hillock Where axon originates and action potentials are initiated Axon terminal Releases neurotransmitter © 2017 Pearson Education, Inc. Figure 7.2 Structure of a typical neuron. Dendrites Cell body (soma) Axon terminals Nucleus Direction of signal Axon hillock Presynaptic neuron Axon Synapse Dendrites Axon terminal Postsynaptic neuron Neurotransmitter (after release) Axon terminals Axon Collateral axon © 2017 Pearson Education, Inc. Figure 7.3 Variations in the extent of dendritic branching. © 2017 Pearson Education, Inc. Transport in Neurons Anterograde transport From soma to axon terminal Retrograde transport From axon to soma Microtubules and neurofilaments Slow: 0.5–40 mm/day Fast: 100–400 mm/day Vesicles Kinesins © 2017 Pearson Education, Inc. Figure 7.4 Fast axonal transport of vesicles. Synaptic vesicle Kinesin protein Microtubule Smooth endoplasmic reticulum Nucleus Axon terminals Golgi apparatus Synaptic vesicle (empty) Rough endoplasmic reticulum Transport vesicles Microtubule Synaptic vesicle (with neurotransmitters) © 2017 Pearson Education, Inc. Synapse Site of communication between two neurons or between a neuron and an effector organ © 2017 Pearson Education, Inc. Ion Channels: Neurons Leak channels Always open Throughout the neuron Resting membrane potential Ligand-gated channels Open or close in response to ligand binding Dendrites and cell body Synaptic potentials © 2017 Pearson Education, Inc. Ion Channels: Neurons Voltage-gated channels Open or close in response to changes in membrane potential Sodium and potassium channels Throughout, but more in the axon (especially in the axon hillock) Action potentials Calcium channels Axon terminal Release of neurotransmitter © 2017 Pearson Education, Inc. Structural Classes of Neurons Bipolar Pseudo-unipolar Multipolar © 2017 Pearson Education, Inc. Figure 7.5 Structural classes of neurons. Dendrites Dendrite Peripheral axon Cell body Cell body Axon Bipolar © 2017 Pearson Education, Inc. Central axon Pseudo-unipolar Axon Multipolar Functional Classes of Neurons Afferent neurons Efferent neurons Interneurons © 2017 Pearson Education, Inc. Figure 7.6 Functional classes of neurons. Peripheral Nervous System Central Nervous System Central axon Cell body Peripheral axon Interneurons Input Afferent neuron Output Cell body © 2017 Pearson Education, Inc. Sensory/ visceral receptors Effector organs Efferent neuron Structural Organization of Neurons Nuclei Pathways, tracts, commissures Ganglia Nerves © 2017 Pearson Education, Inc. Glial Cells 90% of all cells in the nervous system Glia: Latin, meaning "glue" Four types: Astrocytes Microglia Oligodendrocytes Schwann cells © 2017 Pearson Education, Inc. Myelin-Forming Cells Oligodendrocytes Central nervous system One oligodendrocyte: Forms several myelin sheaths Myelinates sections of several axons © 2017 Pearson Education, Inc. Myelin-Forming Cells Schwann cell Peripheral nervous system One Schwann cell: Forms one myelin sheath Myelinates one section of an axon © 2017 Pearson Education, Inc. Figure 7.7a Formation and origins of myelin sheaths. Axon Nucleus Cytoplasm © 2017 Pearson Education, Inc. Schwann cell Myelin sheath Cytoplasm Figure 7.7b-c Formation and origins of myelin sheaths. Schwann cell Nucleus Node of Ranvier Node of Ranvier Oligodendrocyte Myelin sheath Axon Myelin sheath Axon Nucleus © 2017 Pearson Education, Inc. Figure 7.7d Formation and origins of myelin sheaths. Myelin sheath © 2017 Pearson Education, Inc. Axon 7.3 Resting Membrane Potential Establishment Determining equilibrium potentials For potassium ions For sodium ions Exists because there are more negative charges inside the cell and more positive charges outside the cell All cells have a resting membrane potential –5 mV to –100 mV Resting membrane potential of neurons Approximately –70 mV © 2017 Pearson Education, Inc. Equilibrium Potentials Determining the equilibrium potentials for potassium and sodium ions Two factors are critical in determining resting membrane potential: Ion concentration gradients Membrane permeability to these ions Ion channels © 2017 Pearson Education, Inc. © 2017 Pearson Education, Inc. Na+/K+ Pump 20% of the resting membrane potential is directly due to Na+/K+-ATPase Electrogenic: 3 Na+ out, 2 K+ in Net: +1 out 80% of resting membrane potential is indirectly due to Na+/K+-ATPase Produces concentration gradients Na+: high outside, low inside K+: low outside, high inside © 2017 Pearson Education, Inc. Potassium Equilibrium Potential K+ chemical driving force: out of the cell K+ diffuses out of the cell Cell permeable to potassium only High [Na+] High [Cl–] High [K+] High [A–] No membrane potential 0 mV Chemical force © 2017 Pearson Education, Inc. K+ ions Potassium Equilibrium Potential As K+ diffuses out of the cell, the inside of the cell becomes more negative Electrical driving force acts to "pull" K+ back into the cell Cell permeable to potassium only + – + – – Inside of cell becomes (–) + – Chemical force © 2017 Pearson Education, Inc. + – + Electrical force Potassium Equilibrium Potential The cell eventually reaches equilibrium Chemical and electrical driving forces: Opposite in direction Equal in magnitude Cell permeable to potassium only + + – – + + – + – – + – + – + Equilibrium – potential – EK = –94 mV + – – – + + + Electrical force exactly opposes chemical force © 2017 Pearson Education, Inc. Equilibrium An ion is at equilibrium when there is no net force for it to move across the membrane Chemical force = negative electrical force or Electrochemical force = 0 © 2017 Pearson Education, Inc. K+ Equilibrium Potential Nernst equation (Chapter 4) EK = 61 mV × log 4 mM = –94 mV 140 mM When membrane potential = –94 mV, potassium is at equilibrium. © 2017 Pearson Education, Inc. Establishing the Sodium Equilibrium Potential Hypothetical cell Permeable only to sodium Ion distribution Outside cell: sodium and chloride Inside cell: potassium and organic anions © 2017 Pearson Education, Inc. Forces Acting on Na+ Two forces acting on Na+: Chemical: to move in Electrical: to move out Net force = electrochemical force Initially in, due to stronger chemical force But electrical force continues to increase © 2017 Pearson Education, Inc. Sodium Equilibrium Potential Na+ chemical driving force: into the cell Na+ diffuses into the cell Cell permeable to sodium only High [Na+] High [Cl–] High [K+] High [A–] No membrane potential 0 mV Chemical force © 2017 Pearson Education, Inc. Na+ ions Sodium Equilibrium Potential As Na+ diffuses into the cell, the inside of the cell becomes less negative (positive) Electrical driving force acts to "push" Na+ out of the cell Cell permeable to sodium only – + – + + Inside of cell becomes (+) – + Chemical force © 2017 Pearson Education, Inc. – + – Electrical force Sodium Equilibrium Potential The cell eventually reaches equilibrium Chemical and electrical driving forces: Opposite in direction Equal in magnitude Cell permeable to sodium only – + – – + + – – + – + + – + Equilibrium potential + + ENa = +60 mV – + + + – – – – Electrical force exactly opposes chemical force © 2017 Pearson Education, Inc. Na+ Equilibrium Potential ENa = 61 mV × log 145 = +60 mV 15 When membrane potential = +60 mV, sodium is at equilibrium. © 2017 Pearson Education, Inc. Resting Membrane Potential of Neurons Typical neuron Permeable to potassium and sodium 25 times more permeable to potassium Ion distribution Outside cell: sodium and chloride Inside cell: potassium and organic anions © 2017 Pearson Education, Inc. Resting Potential: Neuron Chemical driving forces K+ out Na+ in Na+ ions [Na+] High High [Cl–] Chemical force for K+ High [K+] High [A–] No membrane potential 0 mV Chemical force for Na+ © 2017 Pearson Education, Inc. Resting Potential: Neuron Membrane is more permeable to K+ More K+ leaves the cell than Na+ enters Inside of the cell becomes negative + – – + – Inside of cell becomes (–) – – + © 2017 Pearson Education, Inc. + + Resting Potential: Neuron Electrical forces develop: Na+ into cell K+ into cell Chemical force for K+ + Due to electrical forces: K+ outflow slows Na+ inflow speeds + – – – Inside of cell (–) – + Chemical force for Na+ © 2017 Pearson Education, Inc. Electrical force for K+ – + + Electrical force for Na+ Resting Potential: Neuron Steady state develops Inflow of Na+ is balanced by outflow of K+ + – Resting membrane potential = –70 mV + + – Membrane potential = –70 mV – + © 2017 Pearson Education, Inc. – – + – + – – + + – + – – – + + + Resting Potential: Neuron Sodium pump maintains the resting potential + – + + – © 2017 Pearson Education, Inc. ATP Membrane potential = –70 mV – + Na+/K+ + pump – – + – + – – + – + – – – + + + Resting Membrane Potential The resting membrane potential is closer to the potassium equilibrium potential © 2017 Pearson Education, Inc. Forces Acting on Ions If the membrane potential is not at equilibrium for an ion: The electrochemical force is not 0 The net force acts to move the ion across the membrane in the direction that favors its being at equilibrium The strength of the net force increases the farther away the membrane potential is from the equilibrium potential © 2017 Pearson Education, Inc. Resting Potential: Forces on K+ Resting potential = –70 mV EK = –94 mV Vm is 24 mV less negative than EK Electrical force is into the cell (lower) Chemical force is out of the cell (higher) Net force is weak: K+ flows out of the cell, but the membrane is highly permeable to K+ © 2017 Pearson Education, Inc. Resting Potential: Forces on Na+ Resting potential = –70 mV ENa = +60 mV Vm is 130 mV less negative than ENa Electrical force is into the cell Chemical force is also into the cell Net force is strong: Na+ flows into the cell, but the membrane has low permeability to Na+ © 2017 Pearson Education, Inc. A Neuron at Rest Small Na+ leak at rest (high force, low permeability) Small K+ leak at rest (low force, high permeability) Sodium pump returns Na+ and K+ to maintain gradients © 2017 Pearson Education, Inc. + – + + – ATP Membrane potential = –70 mV – + Na+/K+ + pump – – + – + – – + – + – – – + + + Resting Membrane Potential © 2017 Pearson Education, Inc. 7.4 Electrical Signaling Through Changes in Membrane Potential Describing changes in membrane potential Graded potentials Action potentials Propagation of action potentials © 2017 Pearson Education, Inc. Electrical Signals: Neurons Membrane potential changes Due to gated channels Open or close in response to stimuli Affect movement of ions Ion movement—electrical signal © 2017 Pearson Education, Inc. Electrical Signals: Neurons Types of gated channels Voltage gated Ligand (chemically) gated Mechanically gated © 2017 Pearson Education, Inc. Membrane Potential Changes Resting potential—reference point Depolarization Repolarization Hyperpolarization © 2017 Pearson Education, Inc. Figure 7.11 Changes in membrane potential. Membrane potential (mV) ENa 0 Depolarization Resting potential –70 Hyperpolarization EK © 2017 Pearson Education, Inc. Repolarization Types of Electrical Signals Graded potentials Small Communicate over short distances Action potentials Large Communicate over long distances © 2017 Pearson Education, Inc. Graded Potentials Initiated by a stimulus Small change in membrane potential Magnitude varies (graded) © 2017 Pearson Education, Inc. Membrane potential (mV) Figure 7.12 Effect of stimulus strength on size of graded potential. X © 2017 Pearson Education, Inc. Stronger X Graded Potentials Spread by electrotonic conduction Are decremental Magnitude decays as it spreads © 2017 Pearson Education, Inc. Figure 7.13 Decremental property of graded potentials. Stimulus Membrane potential (mV) Membrane potential (mV) Axon hillock © 2017 Pearson Education, Inc. Recorded at site of stimulus Recorded at axon hillock Purpose of Graded Potentials Graded potentials determine whether an action potential will occur Threshold Level of depolarization necessary to elicit action potential Excitatory Depolarization Inhibitory Hyperpolarization © 2017 Pearson Education, Inc. Graded Potentials Some are depolarizing Some are hyperpolarizing © 2017 Pearson Education, Inc. Membrane potential (mV) Figure 7.14 Effect of stimulus type on graded potentials. Y (hyperpolarizing) Time (msec) © 2017 Pearson Education, Inc. Z (depolarizing) Graded Potentials Can Sum Temporal summation Same stimulus Repeated close together in time Spatial summation Different stimuli Overlap in time © 2017 Pearson Education, Inc. Figure 7.15a-b Temporal and spatial summation. Membrane potential (mV) Membrane potential (mV) Action potential –55 W © 2017 Pearson Education, Inc. X Y Time (msec) –55 Threshold W1 W2 W3 Time (msec) Membrane potential (mV) Figure 7.15c Temporal and spatial summation. –55 W © 2017 Pearson Education, Inc. X W+X Time (msec) Membrane potential (mV) Figure 7.15d Temporal and spatial summation. –55 W © 2017 Pearson Education, Inc. Y W+Y Time (msec) Action Potentials Excitable membranes have the ability to generate action potentials Action potential Rapid large depolarization is used for communication In neurons Action potentials travel along axons from the cell body to the axon terminal (or if an afferent neuron, from the receptor to the terminal) © 2017 Pearson Education, Inc. Phases of an Action Potential Depolarization Repolarization After-hyperpolarization © 2017 Pearson Education, Inc. Figure 7.16a The phases and ionic basis of an action potential. Action potential Membrane potential (mV) +30 Phase 1 –55 –70 Phase 2 Threshold Resting potential Phase 3 Time (msec) © 2017 Pearson Education, Inc. Three phases of an action potential Figure 7.16b The phases and ionic basis of an action potential. Action potential PNa –55 –70 Na+ channels open © 2017 Pearson Education, Inc. PK K+ channels open Time (msec) Permeability changes for Na+ and K+ during an action potential Membrane permeability (P ) Membrane potential (mV) +30 Generation of an Action Potential © 2017 Pearson Education, Inc. © 2017 Pearson Education, Inc. Resting State PK > PNa Membrane potential close to EK Membrane potential far from ENa © 2017 Pearson Education, Inc. Depolarization to Threshold Graded potentials bring membrane to threshold © 2017 Pearson Education, Inc. Threshold Triggers Rapid opening of Na+ channels Regenerative mechanism Slow closing of Na+ channels Slow opening of K+ channels The opening of K+ channels and the closing of Na+ channels is the reason that the action potential does not reach ENa © 2017 Pearson Education, Inc. Repolarization and After-Hyperpolarization Na+ channels closed K+ channels opened K+ channels remain open beyond the resting membrane potential; K+ continues to leave Membrane potential gets close to EK → hyperpolarization © 2017 Pearson Education, Inc. Restoration of Membrane Potential Na+/K+ pump restores the resting membrane potential © 2017 Pearson Education, Inc. Figure 7.16a The phases and ionic basis of an action potential. Action potential Membrane potential (mV) +30 Phase 1 –55 –70 Phase 2 Threshold Resting potential Phase 3 Time (msec) © 2017 Pearson Education, Inc. Three phases of an action potential Voltage-Gated Na+ Channel Two gates are associated with the channel Activation gate Voltage dependent Opens at threshold and depolarization Positive feedback Inactivation gate Voltage and time dependent Opens during depolarization Closes during depolarization © 2017 Pearson Education, Inc. Figure 7.17 A model for the operation of voltage-gated sodium channels. Resting membrane potential Extracellular fluid Na+ Activation gate (closed but capable of opening) Depolarization Plasma membrane Intracellular fluid Na+ channel Inactivation gate (open) Activation gate (open) Na+ Na+ Inactivation gate (open) Activation gate (open) 1 msec Inactivation gate (closed and incapable of opening until return to resting state) © 2017 Pearson Education, Inc. Voltage-Gated Potassium Channels One gate Depends on voltage and time Negative feedback © 2017 Pearson Education, Inc. Figure 7.18 Gating of sodium and potassium channels during an action potential. Slide 1 Threshold stimulus Depolarization of membrane Open sodium channels Positive feedback Membrane sodium permeability Net positive charge in cell (depolarization) Sodium flow into cell Negative feedback Delayed effect (1 msec) Delayed effect (1 msec) Sodium channel inactivation gates close Open potassium channels Membrane sodium permeability Membrane potassium permeability Potassium flow out of cell Initial stimulus Physiological response Result © 2017 Pearson Education, Inc. Sodium flow into cell Net positive charge in cell (repolarization) Figure 7.18 Gating of sodium and potassium channels during an action potential. Threshold stimulus Initial stimulus Physiological response Result © 2017 Pearson Education, Inc. Slide 2 Figure 7.18 Gating of sodium and potassium channels during an action potential. Threshold stimulus Depolarization of membrane Initial stimulus Physiological response Result © 2017 Pearson Education, Inc. Slide 3 Figure 7.18 Gating of sodium and potassium channels during an action potential. Threshold stimulus Depolarization of membrane Open sodium channels Initial stimulus Physiological response Result © 2017 Pearson Education, Inc. Slide 4 Figure 7.18 Gating of sodium and potassium channels during an action potential. Threshold stimulus Depolarization of membrane Open sodium channels Membrane sodium permeability Initial stimulus Physiological response Result © 2017 Pearson Education, Inc. Slide 5 Figure 7.18 Gating of sodium and potassium channels during an action potential. Threshold stimulus Depolarization of membrane Open sodium channels Membrane sodium permeability Sodium flow into cell Initial stimulus Physiological response Result © 2017 Pearson Education, Inc. Slide 6 Figure 7.18 Gating of sodium and potassium channels during an action potential. Threshold stimulus Depolarization of membrane Open sodium channels Membrane sodium permeability Net positive charge in cell (depolarization) Initial stimulus Physiological response Result © 2017 Pearson Education, Inc. Sodium flow into cell Slide 7 Figure 7.18 Gating of sodium and potassium channels during an action potential. Threshold stimulus Depolarization of membrane Open sodium channels Positive feedback Membrane sodium permeability Net positive charge in cell (depolarization) Initial stimulus Physiological response Result © 2017 Pearson Education, Inc. Sodium flow into cell Slide 8 Figure 7.18 Gating of sodium and potassium channels during an action potential. Threshold stimulus Depolarization of membrane Open sodium channels Positive feedback Membrane sodium permeability Net positive charge in cell (depolarization) Sodium flow into cell Delayed effect (1 msec) Initial stimulus Physiological response Result © 2017 Pearson Education, Inc. Slide 9 Figure 7.18 Gating of sodium and potassium channels during an action potential. Threshold stimulus Depolarization of membrane Open sodium channels Positive feedback Membrane sodium permeability Net positive charge in cell (depolarization) Sodium flow into cell Delayed effect (1 msec) Sodium channel inactivation gates close Initial stimulus Physiological response Result © 2017 Pearson Education, Inc. Slide 10 Figure 7.18 Gating of sodium and potassium channels during an action potential. Threshold stimulus Depolarization of membrane Open sodium channels Positive feedback Membrane sodium permeability Net positive charge in cell (depolarization) Sodium flow into cell Delayed effect (1 msec) Sodium channel inactivation gates close Membrane sodium permeability Initial stimulus Physiological response Result © 2017 Pearson Education, Inc. Slide 11 Figure 7.18 Gating of sodium and potassium channels during an action potential. Threshold stimulus Depolarization of membrane Open sodium channels Positive feedback Membrane sodium permeability Net positive charge in cell (depolarization) Sodium flow into cell Delayed effect (1 msec) Sodium channel inactivation gates close Membrane sodium permeability Initial stimulus Physiological response Result © 2017 Pearson Education, Inc. Sodium flow into cell Slide 12 Figure 7.18 Gating of sodium and potassium channels during an action potential. Slide 13 Threshold stimulus Depolarization of membrane Open sodium channels Positive feedback Membrane sodium permeability Net positive charge in cell (depolarization) Sodium flow into cell Delayed effect (1 msec) Sodium channel inactivation gates close Membrane sodium permeability Net positive charge in cell (repolarization) Initial stimulus Physiological response Result © 2017 Pearson Education, Inc. Sodium flow into cell Figure 7.18 Gating of sodium and potassium channels during an action potential. Slide 14 Threshold stimulus Depolarization of membrane Open sodium channels Positive feedback Membrane sodium permeability Net positive charge in cell (depolarization) Sodium flow into cell Delayed effect (1 msec) Delayed effect (1 msec) Sodium channel inactivation gates close Membrane sodium permeability Net positive charge in cell (repolarization) Initial stimulus Physiological response Result © 2017 Pearson Education, Inc. Sodium flow into cell Figure 7.18 Gating of sodium and potassium channels during an action potential. Slide 15 Threshold stimulus Depolarization of membrane Open sodium channels Positive feedback Membrane sodium permeability Net positive charge in cell (depolarization) Sodium flow into cell Delayed effect (1 msec) Delayed effect (1 msec) Sodium channel inactivation gates close Open potassium channels Membrane sodium permeability Net positive charge in cell (repolarization) Initial stimulus Physiological response Result © 2017 Pearson Education, Inc. Sodium flow into cell Figure 7.18 Gating of sodium and potassium channels during an action potential. Slide 16 Threshold stimulus Depolarization of membrane Open sodium channels Positive feedback Membrane sodium permeability Net positive charge in cell (depolarization) Sodium flow into cell Delayed effect (1 msec) Delayed effect (1 msec) Sodium channel inactivation gates close Open potassium channels Membrane sodium permeability Membrane potassium permeability Net positive charge in cell (repolarization) Initial stimulus Physiological response Result © 2017 Pearson Education, Inc. Sodium flow into cell Figure 7.18 Gating of sodium and potassium channels during an action potential. Slide 17 Threshold stimulus Depolarization of membrane Open sodium channels Positive feedback Membrane sodium permeability Net positive charge in cell (depolarization) Sodium flow into cell Delayed effect (1 msec) Delayed effect (1 msec) Sodium channel inactivation gates close Open potassium channels Membrane sodium permeability Membrane potassium permeability Potassium flow out of cell Initial stimulus Physiological response Result © 2017 Pearson Education, Inc. Sodium flow into cell Net positive charge in cell (repolarization) Figure 7.18 Gating of sodium and potassium channels during an action potential. Slide 18 Threshold stimulus Depolarization of membrane Open sodium channels Positive feedback Membrane sodium permeability Net positive charge in cell (depolarization) Sodium flow into cell Delayed effect (1 msec) Delayed effect (1 msec) Sodium channel inactivation gates close Open potassium channels Membrane sodium permeability Membrane potassium permeability Potassium flow out of cell Initial stimulus Physiological response Result © 2017 Pearson Education, Inc. Sodium flow into cell Net positive charge in cell (repolarization) Figure 7.18 Gating of sodium and potassium channels during an action potential. Slide 19 Threshold stimulus Depolarization of membrane Open sodium channels Positive feedback Membrane sodium permeability Net positive charge in cell (depolarization) Sodium flow into cell Negative feedback Delayed effect (1 msec) Delayed effect (1 msec) Sodium channel inactivation gates close Open potassium channels Membrane sodium permeability Membrane potassium permeability Potassium flow out of cell Initial stimulus Physiological response Result © 2017 Pearson Education, Inc. Sodium flow into cell Net positive charge in cell (repolarization) © 2017 Pearson Education, Inc. 30 Threshold –55 –70 Stimulus strength Membrane potential (mV) Figure 7.19 The concept of a threshold stimulus. Subthreshold stimuli © 2017 Pearson Education, Inc. Threshold Suprathreshold stimulus stimulus Time (msec) All-or-None Principle Threshold Minimum depolarization necessary to induce the regenerative mechanism for the opening of sodium channels Threshold depolarization action potential Subthreshold depolarization no action potential Suprathreshold depolarization action potential Action potentials from threshold and suprathreshold stimuli are the same magnitude 100 mV (–70 mV → +30 mV) © 2017 Pearson Education, Inc. Refractory Period Period of time following an action potential Marked by decreased excitability Two types: Absolute Relative © 2017 Pearson Education, Inc. Refractory Periods Absolute Spans all of depolarization and most of the repolarization phase Second action potential cannot be generated Na+ gates are inactivated © 2017 Pearson Education, Inc. Refractory Periods Relative Spans the last part of the repolarization phase and hyperpolarization Second action potential can be generated—with a stronger stimulus Some Na+ gates are closed; some are inactivated © 2017 Pearson Education, Inc. Figure 7.20a Refractory periods associated with an action potential. Relative refractory period Membrane potential (mV) Absolute refractory period © 2017 Pearson Education, Inc. Stimulus Time (msec) Figure 7.20b Refractory periods associated with an action potential. Strength of stimulus required to generate a second action potential No stimulus, regardless of strength, can generate a second action potential during the absolute refractory period A stimulus much stronger than normal is necessary to generate a second action potential early in the relative refractory period. A stimulus a little stronger than normal is necessary to generate an action potential late in the relative refractory period Stimulus © 2017 Pearson Education, Inc. Time (msec) 100 100 % Na+ channels inactivated % Voltage-gated K+ channels open Stimulus © 2017 Pearson Education, Inc. Time (msec) % Voltage-gated K+ channels open % Na+ channels inactivated Figure 7.20c Refractory periods associated with an action potential. Consequences of Refractory Periods All-or-none principle Frequency coding Unidirectional propagation of action potentials © 2017 Pearson Education, Inc. Membrane potential (mV) Figure 7.21a Frequency coding: how action potentials convey intensity of stimuli. 30 Stimulus strength –55 –70 10-msec subthreshold stimulus 10-msec threshold stimulus Time (sec) © 2017 Pearson Education, Inc. 20-msec threshold stimulus Membrane potential (mV) Figure 7.21b Frequency coding: how action potentials convey intensity of stimuli. 30 Threshold Stimulus strength –55 –70 Subthreshold Threshold stimulus stimulus Suprathreshold stimuli Time (sec) © 2017 Pearson Education, Inc. Propagation of Action Potentials Mechanisms depend on the presence or absence of myelin © 2017 Pearson Education, Inc. Figure 7.22 Action potential conduction in unmyelinated axons. Extracellular fluid Slide 1 Axon hillock Unmyelinated axon + + Extracellular fluid + + – + + + + + + + + + + + + + + + + – – – – – – – – – – – – – – – – – – – + Intracellular fluid + + + – + + + + + + + + + + + + + + + + + – – – – – – – – – – – – – – – – – – – Extracellular fluid – Site of original action potential + + + – – – – + + + + + + + + + + + + – – – + + + + – – – – – – – – – – – – Site A Initiation Resting Site B + + + – + + + + + + + + + + + + – – – – + – – – – – – – – – – – – – + + + + – – Plasma membrane Region of depolarization Direction of action potential propagation – + + + + + + + – – – – + + + + + + + + – – – – – – – + + + + – – – – – – – – Site A Site B Site C + + + – + + + + + + + + – – – – + + + + + – – – – – – – – – – + + + + – – – – – Refractory Region of state depolarization Propagation © 2017 Pearson Education, Inc. + – + + + + + + + + + + + – – – – + + + + – – – – – – – – – – – + + + + – – – – Site A Site B Site C Site D + + + – + + + + – – – – + + + + + + + + + – – – – – – + + + + – – – – – – – – – + Region of Refractory Region of repolarization state depolarization (resting state) Propagation continues Figure 7.22 Action potential conduction in unmyelinated axons. Extracellular fluid Axon hillock Unmyelinated axon © 2017 Pearson Education, Inc. Slide 2 Figure 7.22 Action potential conduction in unmyelinated axons. Extracellular fluid Axon hillock Unmyelinated axon Plasma membrane + – Extracellular fluid + + + + + + + + + + + + + + + + + + – – – – – – – – – – – – – – – – – – – + Intracellular fluid + + + – + + + + + + + + + + + + + + + + + – – – – – – – – – – – – – – – – – – – Extracellular fluid Resting © 2017 Pearson Education, Inc. Slide 3 Figure 7.22 Action potential conduction in unmyelinated axons. Extracellular fluid Slide 4 Axon hillock Unmyelinated axon + + Extracellular fluid + + – + + + + + + + + + + + + + + + + – – – – – – – – – – – – – – – – – – – + Intracellular fluid + + + – + + + + + + + + + + + + + + + + + – – – – – – – – – – – – – – – – – – – Extracellular fluid Resting © 2017 Pearson Education, Inc. – Site of original action potential Initiation + + + – – – – + + + + + + + + + + + + – – – + + + + – – – – – – – – – – – – Site A Site B + + + – + + + + + + + + + + + + – – – – + – – – – – – – – – – – – – + + + + – – Plasma membrane Region of depolarization Figure 7.22 Action potential conduction in unmyelinated axons. Extracellular fluid Slide 5 Axon hillock Unmyelinated axon + + Extracellular fluid + + – + + + + + + + + + + + + + + + + – – – – – – – – – – – – – – – – – – – + Intracellular fluid + + + – + + + + + + + + + + + + + + + + + – – – – – – – – – – – – – – – – – – – Extracellular fluid – Site of original action potential Initiation Resting Direction of action potential propagation + – + + + + + + + – – – – + + + + + + + + – – – – – – – + + + + – – – – – – – – Site A Site B Site C + + + – + + + + + + + + – – – – + + + + + – – – – – – – – – – + + + + – – – – – Refractory Region of state depolarization Propagation © 2017 Pearson Education, Inc. + + + – – – – + + + + + + + + + + + + – – – + + + + – – – – – – – – – – – – Site A Site B + + + – + + + + + + + + + + + + – – – – + – – – – – – – – – – – – – + + + + – – Plasma membrane Region of depolarization Figure 7.22 Action potential conduction in unmyelinated axons. Extracellular fluid Slide 6 Axon hillock Unmyelinated axon + + Extracellular fluid + + – + + + + + + + + + + + + + + + + – – – – – – – – – – – – – – – – – – – + Intracellular fluid + + + – + + + + + + + + + + + + + + + + + – – – – – – – – – – – – – – – – – – – Extracellular fluid – Site of original action potential + + + – – – – + + + + + + + + + + + + – – – + + + + – – – – – – – – – – – – Site A Initiation Resting Site B + + + – + + + + + + + + + + + + – – – – + – – – – – – – – – – – – – + + + + – – Plasma membrane Region of depolarization Direction of action potential propagation – + + + + + + + – – – – + + + + + + + + – – – – – – – + + + + – – – – – – – – Site A Site B Site C + + + – + + + + + + + + – – – – + + + + + – – – – – – – – – – + + + + – – – – – Refractory Region of state depolarization Propagation © 2017 Pearson Education, Inc. + – + + + + + + + + + + + – – – – + + + + – – – – – – – – – – – + + + + – – – – Site A Site B Site C Site D + + + – + + + + – – – – + + + + + + + + + – – – – – – + + + + – – – – – – – – – + Region of Refractory Region of repolarization state depolarization (resting state) Propagation continues Factors Affecting Propagation Refractory period Unidirectional Axon diameter Larger: less resistance, faster Smaller: more resistance, slower Myelination Saltatory conduction Faster propagation © 2017 Pearson Education, Inc. Figure 7.23 Saltatory conduction in myelinated axons. Slide 1 Extracellular fluid Axon hillock Myelinated axon + Myelin sheath Node of Ranvier + + + + + + + + + + + + + + – + + + – – – – – – – – – – – – – – – – – – – – – Intracellular fluid – + + + – – – – – – – – – – – – – – – – – – – – – – – – + + + + + + + + + + + + + + + + + + + + + + Extracellular fluid – – – + + + + + + + Direction of action potential propagation + + + + + + + + + + + + + + + + – – – + + + + + + – – – – – – – – – – – + + + – – – – – – – – – – – – – – – – – – – – – – + + + – – – – – – – – – – – – – – + + + + + + + + + + + + + + + + + + + + + + © 2017 Pearson Education, Inc. Figure 7.23 Saltatory conduction in myelinated axons. Myelinated axon + – – – + + + + + + + Myelin sheath + + + + + + + Slide 2 Node of Ranvier + + + + + + + – + + + – – – – – – – – – – – – – – – – – – – – – Intracellular fluid – + + + – – – – – – – – – – – – – – – – – – – – – – – – + + + + + + + + + + + + + + + + + + + + + + Extracellular fluid © 2017 Pearson Education, Inc. Figure 7.23 Saltatory conduction in myelinated axons. Myelinated axon + – – – + + + + + + + Myelin sheath + + + + + + + Slide 3 Node of Ranvier + + + + + + + – + + + – – – – – – – – – – – – – – – – – – – – – Intracellular fluid – + + + – – – – – – – – – – – – – – – – – – – – – – – – + + + + + + + + + + + + + + + + + + + + + + Extracellular fluid Direction of action potential propagation + + + + + + + + – – – + + + + + + + + + + + + + + – – – – – – – – – – – + + + – – – – – – – – – – – – – – – – – – – – – – + + + – – – – – – – – – – – – – – + + + + + + + + + + + + + + + + + + + + + + © 2017 Pearson Education, Inc. Figure 7.23 Saltatory conduction in myelinated axons. Slide 4 Extracellular fluid Axon hillock Myelinated axon + Myelin sheath Node of Ranvier + + + + + + + + + + + + + + – + + + – – – – – – – – – – – – – – – – – – – – – Intracellular fluid – + + + – – – – – – – – – – – – – – – – – – – – – – – – + + + + + + + + + + + + + + + + + + + + + + Extracellular fluid – – – + + + + + + + Direction of action potential propagation + + + + + + + + + + + + + + + + – – – + + + + + + – – – – – – – – – – – + + + – – – – – – – – – – – – – – – – – – – – – – + + + – – – – – – – – – – – – – – + + + + + + + + + + + + + + + + + + + + + + © 2017 Pearson Education, Inc. © 2017 Pearson Education, Inc. 7.5 Maintaining Neural Stability Graded potentials and action potentials tend to dissipate Na+ and K+ concentration gradients Only a small percentage of ions actually move The Na+/K+ pump prevents dissipation © 2017 Pearson Education, Inc.