Chapter 60 The Autonomic Nervous System and the Adrenal Medulla PDF
Document Details
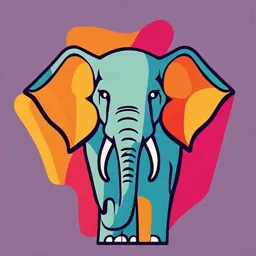
Uploaded by CharitableGoshenite9955
Tags
Summary
This document provides an overview of the autonomic nervous system (ANS), including its subdivisions, functions, and related concepts. It explores the processes of the ANS; including the types of neurons, neurotransmitters, and how the ANS impacts effector organs.
Full Transcript
ANS is a critical component of our nervous system, responsible for regulating involuntary physiological functions. It's integral to maintaining homeostasis within the body. The autonomic nervous system (ANS) controls most visceral functions of the body. The ANS helps regulate: Arteria...
ANS is a critical component of our nervous system, responsible for regulating involuntary physiological functions. It's integral to maintaining homeostasis within the body. The autonomic nervous system (ANS) controls most visceral functions of the body. The ANS helps regulate: Arterial pressure Gastrointestinal motility Gastrointestinal secretion Urinary bladder emptying Sweating Body temperature Other activities, some entirely and some partially controlled by the ANS Key characteristics of the ANS include: Rapid and intense changes in visceral functions Heart rate can double within 3 to 5 seconds Arterial pressure can double or decrease significantly within 10 to 15 seconds, potentially causing fainting Sweating can start within seconds Urinary bladder can empty involuntarily within seconds Efferent Autonomic Signals Transmitted through two subdivisions: Sympathetic Nervous System Parasympathetic Nervous System Physiologic Anatomy of the Sympathetic Nervous System Peripheral organization includes: Two paravertebral sympathetic chains of ganglia Two prevertebral ganglia (celiac and hypogastric) Nerves extending to internal organs Sympathetic nerve fibers originate in the spinal cord between T-1 and L-2 Fibers pass into the sympathetic chain and then to tissues and organs Sympathetic Neurons Pathway includes: Preganglionic neuron: cell body in the intermediolateral horn of the spinal cord, fiber passes through the anterior root into the spinal nerve Postganglionic neuron: originates in sympathetic chain ganglia or peripheral ganglia, travels to various organs Three possible courses for preganglionic fibers: Synapse in the ganglion entered Pass up or down the chain and synapse in another ganglion Pass through the chain and synapse in a peripheral ganglion Sympathetic Nerve Fibers in Skeletal Nerves Postganglionic fibers pass back into spinal nerves through gray rami Extend to blood vessels, sweat glands, and piloerector muscles About 8% of fibers in skeletal nerves are sympathetic fibers Segmental Distribution Sympathetic pathways from spinal segments have specific distributions: T-1: head T-2: neck T-3 to T-6: thorax T-7 to T-11: abdomen T-12 to L-2: legs Distribution relates to embryonic origin of organs Sympathetic Nerve Endings in the Adrenal Medullae Preganglionic fibers pass directly to the adrenal medullae without synapsing End on modified neuronal cells secreting epinephrine and norepinephrine These cells are embryologically derived from nervous tissue and function as postganglionic neurons Parasympathetic Nervous System Anatomy Parasympathetic fibers leave the central nervous system through: Cranial nerves III, VII, IX, and X Lower part of the spinal cord through second and third sacral spinal nerves, occasionally first and fourth sacral nerves About 75% of parasympathetic fibers are in the vagus nerves (cranial nerve X) Vagus nerves supply the heart, lungs, esophagus, stomach, small intestine, proximal half of the colon, liver, gallbladder, pancreas, kidneys, and upper portions of the ureters Specific Cranial Nerve Contributions Cranial nerve III: pupillary sphincter and ciliary muscle of the eye Cranial nerve VII: lacrimal, nasal, and submandibular glands Cranial nerve IX: parotid gland Sacral Parasympathetic Fibers Found in pelvic nerves through the spinal nerve sacral plexus at S-2 and S-3 levels Distribute to descending colon, rectum, urinary bladder, and lower portions of the ureters Supply nerve signals to external genitalia causing erection Preganglionic and Postganglionic Parasympathetic Neurons Preganglionic fibers typically pass uninterrupted to the target organ Postganglionic neurons located in the wall of the target organ Synapse with preganglionic fibers Postganglionic fibers are very short, innervating the tissues of the organ Different from sympathetic system where postganglionic neurons are located in ganglia outside the target organ Cholinergic and Adrenergic Fibers Neurotransmitter Substances Acetylcholine Norepinephrine Cholinergic Fibers Secrete acetylcholine All preganglionic neurons in both sympathetic and parasympathetic systems Most postganglionic neurons in the parasympathetic system Postganglionic sympathetic fibers to sweat glands, piloerector muscles, and some blood vessels Adrenergic Fibers Secrete norepinephrine Most postganglionic neurons in the sympathetic system Function and Excitation Acetylcholine excites both sympathetic and parasympathetic postganglionic neurons Neurotransmitter action: Acetylcholine: parasympathetic effects Norepinephrine: sympathetic effects Secretion Mechanism of Acetylcholine and Norepinephrine Structure of Postganglionic Nerve Endings Parasympathetic nerve endings are similar but smaller than skeletal neuromuscular junctions Many parasympathetic and most sympathetic fibers touch effector cells or terminate in adjacent connective tissue Varicosities: bulbous enlargements where transmitter vesicles are synthesized and stored Mitochondria in varicosities provide ATP for transmitter synthesis Action Potential and Transmitter Release Action potential depolarizes terminal fibers Increased membrane permeability to calcium ions Calcium ions diffuse into nerve terminals or varicosities Calcium influx causes vesicles to release their contents Acetylcholine or norepinephrine is secreted to stimulate the target cells Synthesis and Secretion of Acetylcholine Synthesized in terminal endings and varicosities of cholinergic nerve fibers Stored in vesicles until release Chemical reaction: Acetyl-CoA + Choline → Acetylcholine (catalyzed by choline acetyltransferase) Secretion occurs when action potential increases calcium ion permeability, causing vesicles to release acetylcholine Destruction and Duration of Action of Acetylcholine Acetylcholine persists in the tissue for a few seconds Split into acetate ion and choline by acetylcholinesterase Choline is transported back into the nerve ending for reuse Similar mechanism as in neuromuscular junctions of skeletal nerve fibers Synthesis of Norepinephrine Begins in the axoplasm of terminal nerve endings Completed inside secretory vesicles Basic steps: Tyrosine → Dopa (hydroxylation) Dopa → Dopamine (decarboxylation) Dopamine → Norepinephrine (hydroxylation) In the adrenal medulla, norepinephrine is converted to epinephrine (methylation) Removal of Norepinephrine Removed from secretory site in three ways: Reuptake into adrenergic nerve endings (50-80%) Diffusion into surrounding body fluids and blood Destruction by tissue enzymes (e.g., monoamine oxidase, catechol-O-methyl transferase) Norepinephrine remains active for a few seconds in tissue; 10 to 30 seconds in blood Receptors on Effector Organs Neurotransmitters must bind to specific receptors on effector cells Receptor on cell membrane, part of a protein molecule penetrating the membrane Binding causes conformational change in protein structure, exciting or inhibiting the cell Changes cell membrane permeability to ions Activates or inactivates enzymes attached to receptor protein Excitation/Inhibition by Membrane Permeability Changes Conformational change in receptor protein can open/close ion channels Sodium/calcium ion channels open: depolarizes and excites the cell Potassium channels open: potassium diffuses out, creating hypernegativity and inhibiting the cell Changed ion environment can promote actions such as smooth muscle contraction Receptor Action by Altering Intracellular "Second Messenger" Enzymes Receptors can activate/inactivate enzymes or other chemicals inside the cell Example: norepinephrine binds to receptor, activating adenylyl cyclase inside the cell Adenylyl cyclase increases cyclic adenosine monophosphate (cAMP) formation cAMP initiates various intracellular actions depending on the effector cell's chemical machinery Transmitter effects (inhibition/excitation) depend on receptor protein and its conformational state in different organs Types of Acetylcholine Receptors Muscarinic Receptors Activated by muscarine (poison from toadstools) Found on all effector cells stimulated by postganglionic cholinergic neurons of parasympathetic and sympathetic systems Nicotinic Receptors Activated by nicotine Found in autonomic ganglia at synapses between preganglionic and postganglionic neurons of both sympathetic and parasympathetic systems Also present at neuromuscular junctions in skeletal muscle Importance: Specific drugs can stimulate or block muscarinic or nicotinic receptors Types of Adrenergic Receptors Alpha Receptors Divided into alpha1 and alpha2 receptors Beta Receptors Divided into beta1, beta2, and beta3 receptors Norepinephrine Mainly excites alpha receptors, also excites beta receptors to a lesser extent Epinephrine Excites both alpha and beta receptors approximately equally Effects on effector organs depend on receptor types present: If predominantly beta receptors, epinephrine is more effective Synthetic Hormone (Isopropyl Norepinephrine) Strong action on beta receptors, essentially no action on alpha receptors Excitatory and Inhibitory Actions of Sympathetic and Parasympathetic Stimulation Effects of Stimulation: Sympathetic and parasympathetic stimulation can both cause excitatory and inhibitory effects, but in different organs. Example: Sympathetic stimulation may excite one organ but inhibit another, while parasympathetic stimulation may have the opposite effects on those same organs. Reciprocal Actions: When sympathetic stimulation excites an organ, parasympathetic stimulation may inhibit it, and vice versa. This reciprocal action demonstrates the balance and opposition between the two systems in controlling organ functions. Dominance of Control: Most organs are predominantly controlled by either the sympathetic or parasympathetic system. The dominant system exerts the primary influence on the organ’s function. No Generalization: It is not possible to generalize whether sympathetic or parasympathetic stimulation will cause excitation or inhibition in a particular organ. Understanding the functions requires learning the specific effects of each system on each organ as detailed in Table 60-2. Examples of Effects (from Table 60-2, hypothetical examples for illustration): Heart: Sympathetic: Increases heart rate (excitatory) Parasympathetic: Decreases heart rate (inhibitory) Digestive Tract: Sympathetic: Decreases motility (inhibitory) Parasympathetic: Increases motility (excitatory) Pupils: Sympathetic: Dilates pupils (excitatory) Parasympathetic: Constricts pupils (inhibitory) Understanding the detailed actions of the sympathetic and parasympathetic systems on each organ is crucial for comprehending their overall roles and interactions in maintaining bodily functions. Function of the Adrenal Medullae Stimulation of the sympathetic nerves to the adrenal medullae leads to the release of large quantities of epinephrine and norepinephrine into the bloodstream. These hormones exert widespread effects on various organs throughout the body. Here's an overview of their functions: 1. Composition of Secretion: Approximately 80% epinephrine and 20% norepinephrine. Proportions may vary under different physiological conditions. 2. Effects of Circulating Epinephrine and Norepinephrine: Similar to direct sympathetic stimulation but longer-lasting (2 to 4 minutes). Norepinephrine: Causes constriction of most blood vessels. Increases heart activity, inhibits gastrointestinal tract, and dilates pupils. Epinephrine: Greater effect on cardiac stimulation due to beta receptor stimulation. Weak constriction of muscle vessels compared to norepinephrine. Higher metabolic effect (5 to 10 times greater) compared to norepinephrine. Increases metabolic rate, glycogenolysis, and glucose release into the blood. 3. Value of Adrenal Medullae: Provide a prolonged and indirect means of sympathetic stimulation. Both direct sympathetic pathways and adrenal medullary hormones can stimulate organs. Loss of one mechanism can be compensated by the other. Epinephrine and norepinephrine can stimulate structures not directly innervated by sympathetic fibers. 4. Relation of Stimulus Rate to Effect: Low frequency of stimulation is sufficient for full activation of autonomic effectors. Full activation occurs with nerve impulses discharging 10 to 20 times per second, compared to 50 to 500 or more impulses per second in the skeletal nervous system. The adrenal medullae play a crucial role in modulating sympathetic responses and ensuring physiological responses to stress and other stimuli. Their secretion of epinephrine and norepinephrine provides a systemic, coordinated response throughout the body. Sympathetic and Parasympathetic "Tone" Normal Activity Levels: Both sympathetic and parasympathetic systems are continually active. Basal rates of activity are known as sympathetic tone and parasympathetic tone. Importance of Tone: Allows a single nervous system to regulate both increases and decreases in organ activity. Example: Sympathetic tone maintains systemic arterioles partially constricted; altering stimulation levels can further constrict or dilate vessels. Examples: Sympathetic tone regulates blood vessel constriction. Parasympathetic tone in the gastrointestinal tract maintains normal motility; removal can lead to atony and constipation. Tone can be adjusted by the brain to modulate organ activity. Role of Adrenal Medullae: Basal secretion of epinephrine and norepinephrine contributes to sympathetic tone. Secretion levels are significant enough to maintain blood pressure even if direct sympathetic pathways are removed. Effect of Denervation: Loss of sympathetic or parasympathetic tone immediately affects innervated organs. Blood vessels experience vasodilation followed by intrinsic compensation over time to restore vasoconstriction. Other organs similarly adjust to compensate for lost tone. Denervation Supersensitivity: After nerve destruction, organs become more sensitive to injected norepinephrine or acetylcholine. Increased responsiveness, known as denervation supersensitivity, occurs due to up-regulation of receptors in postsynaptic membranes. Mechanism enhances effector reaction to circulating hormones. Autonomic Reflexes in Visceral Functions Cardiovascular Autonomic Reflexes: Baroreceptor reflex regulates arterial blood pressure and heart rate. Baroreceptors in major arteries detect pressure changes, signaling the brain stem to adjust sympathetic and parasympathetic activity to maintain pressure within normal range. Gastrointestinal Autonomic Reflexes: Upper gastrointestinal tract and rectum are primarily controlled by autonomic reflexes. Appetizing food smell or food presence in mouth triggers signals to brain stem nuclei, initiating parasympathetic signals to secretory glands for digestive juice secretion. Rectal stretching from fecal matter activates spinal cord reflexes, leading to parasympathetic signals promoting peristaltic contractions and defecation. Urinary Bladder Emptying: Similar to rectal emptying, bladder stretching triggers reflex contraction and urinary sphincter relaxation via sacral spinal cord signals, facilitating micturition. Sexual Reflexes: Initiated by both psychological and genital stimuli. Converging impulses in sacral cord lead to erection (parasympathetic) and ejaculation (sympathetic) in males. Other Autonomic Control Functions: Regulation of pancreatic secretion, gallbladder emptying, kidney excretion, sweating, blood glucose concentration, among others, involves reflex contributions. Each function is finely regulated by neural reflex arcs, ensuring appropriate responses to internal and external stimuli. Sympathetic System Responses and the "Alarm" or "Stress" Response Mass Discharge of Sympathetic Nervous System: Occurs when large portions of the sympathetic system discharge simultaneously, often triggered by fright, fear, or severe pain. Results in a widespread reaction throughout the body known as the alarm or stress response. Localized Activation of Sympathetic Nervous System: Examples include heat regulation, local reflexes, and gastrointestinal reflexes. Each function controlled by the sympathetic system can be activated independently in isolated portions as needed. Parasympathetic System and Specific Localized Responses: Parasympathetic functions are often highly specific, causing localized responses in individual organs. Examples include cardiovascular reflexes, salivary and gastric secretion, and rectal emptying reflex. " Alarm" or "Stress" Response: Mass discharge of sympathetic system enhances body's ability for vigorous muscle activity. Effects include increased arterial pressure, blood flow to active muscles, cellular metabolism, blood glucose concentration, muscle strength, mental activity, and blood coagulation rate. Facilitates performance of strenuous physical activity in states of stress or heightened emotion, often referred to as the sympathetic stress response. Sympathetic Alarm Reaction: Activation of sympathetic system during emotional states like rage, elicited by hypothalamic stimulation. Triggers massive sympathetic discharge throughout the body, preparing for vigorous activity ("fight or flight" response). Impact on Behavioral Responses: Behavioral responses mediated through hypothalamus, brain stem reticular areas, and autonomic nervous system. Higher brain areas can strongly influence autonomic function, potentially leading to autonomic-induced diseases such as peptic ulcers, constipation, or heart palpitations. Medullary, Pontine, and Mesencephalic Control of Autonomic Nervous System Brain Stem Autonomic Centers: Various areas in the brain stem regulate autonomic functions such as arterial pressure, heart rate, glandular secretion, gastrointestinal peristalsis, and bladder contraction. Control centers located along the medulla, pons, and mesencephalon, as well as in special nuclei, modulate different autonomic functions. Association with Cardiovascular Regulatory Centers: Cardiovascular and respiratory regulatory centers closely associated in the brain stem. Transection above midpontine level allows basal arterial pressure control, while transection below medulla leads to decreased arterial pressure. Higher Area Influence on Brain Stem Autonomic Centers: Signals from hypothalamus and cerebral cortex can affect brain stem autonomic control centers. Hypothalamic centers can modulate cardiovascular, temperature, salivary, gastrointestinal, and bladder functions, among others. Autonomic centers in the brain stem serve as relay stations for higher brain control activities, especially those originating from the hypothalamus.