Chapter 4: Adrenergic Agonists and Antagonists PDF
Document Details
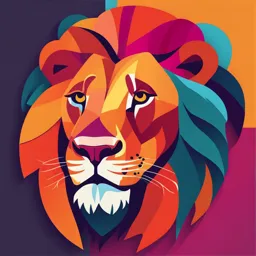
Uploaded by EncouragingAcropolis
Amman Arab University
Tags
Summary
This document is a chapter from a larger work on adrenergic agonists and antagonists, delving into adrenergic receptors, their subtypes, and their roles in neurotransmission. It examines the actions of these agonists and antagonists, including details on catecholamines and non-catecholamines.
Full Transcript
4. Adrenergic agonists 4 & antagonists 4 I. Adrenergic agonists 3 Overview 4 The adrenergic drugs affect receptors that are stimulated by norepinephrine (noradrenaline) or e...
4. Adrenergic agonists 4 & antagonists 4 I. Adrenergic agonists 3 Overview 4 The adrenergic drugs affect receptors that are stimulated by norepinephrine (noradrenaline) or epinephrine (adrenaline). These receptors are known as adrenergic receptors or adrenoceptors. Drugs that activate adrenergic receptors are termed sympathomimetics, and drugs that block activation of adrenergic receptors are termed sympatholytics. Some sympathomimetics directly activate adrenergic receptors (direct-acting agonists), while others act indirectly by enhancing release or blocking reuptake of norepinephrine (indirect-acting agonists). 4 5 THE ADRENERGIC NEURON 4 Adrenergic neurons release norepinephrine as the primary neurotransmitter. These neurons are found in: The central nervous system (CNS) and In the sympathetic nervous system, where they serve as links between ganglia and the effector organs. Adrenergic drugs act on adrenergic receptors, located either: presynaptically on the neuron or postsynaptically on the effector organ. 6 Neurotransmission at adrenergic neuron 4 Neurotransmission in adrenergic neurons closely resembles that described for the cholinergic neurons, except that norepinephrine is the neurotransmitter instead of acetylcholine. Neurotransmission involves the following steps: 1. synthesis, 2. storage, 3. release, and 4. receptor binding of norepinephrine, followed by 5. removal of the neurotransmitter from the synaptic gap 7 4 8 Adrenergic receptors (adrenoceptors) 4 In the sympathetic nervous system, several classes of adrenoceptors can be distinguished pharmacologically. Two main families of receptors, designated α and β, are classified based on response to the adrenergic agonists: epinephrine, norepinephrine, and isoproterenol. Both the α and β receptor types have a number of specific receptor subtypes. Alterations in the primary structure of the receptors influence their affinity for various agents. 9 α-adrenoceptors 4 The α-adrenoceptors show a weak response to the synthetic agonist isoproterenol, but they are responsive to the naturally occurring catecholamines epinephrine and norepinephrine (Figure 6.4). For α receptors, the rank order of potency and affinity is epinephrine ≥ norepinephrine >> isoproterenol. The α-adrenoceptors are divided into two subtypes, α1 and α2, based on their affinities for α agonists and antagonists. E.g., α1 receptors have a higher affinity for phenylephrine than α2 receptors. Conversely, the drug clonidine selectively binds to α2 receptors and has less effect on α1 receptors. 10 α1 receptors 4 α1 receptors are present on the postsynaptic membrane of the effector organs and mediate many of the classic effects, originally designated as α-adrenergic, involving constriction of smooth muscle. Activation of α1 receptors initiates a series of reactions through the G protein activation of phospholipase C, ultimately resulting in the generation of second messengers, inositol-1,4,5-trisphosphate (IP3) and diacylglycerol (DAG). IP3 and DAG initiate the release of Ca2+ from the endoplasmic reticulum into the cytosol. 11 α2 Receptors 4 α2 receptors are located primarily on sympathetic presynaptic nerve endings and control the release of norepinephrine. When a sympathetic adrenergic nerve is stimulated, a portion of the released norepinephrine “circles back” and reacts with α2 receptors on the presynaptic membrane. Stimulation of α2 receptors causes feedback inhibition and inhibits further release of norepinephrine from the stimulated adrenergic neuron. This inhibitory action serves as a local mechanism for modulating norepinephrine output when there is high sympathetic activity [inhibitory autoreceptors]. 12 α2 Receptors 4 α2 Receptors are also found on presynaptic parasympathetic neurons. Norepinephrine released from a presynaptic sympathetic neuron can diffuse to and interact with these receptors on the presynaptic parasympathetic neurons causing inhibition of acetylcholine release [inhibitory heteroreceptors] In contrast to α1 receptors, the effects of binding at α2 receptors are mediated by inhibition of adenylyl cyclase and by a fall in the levels of intracellular cAMP (G-protein coupled receptors (GPCR)) * 13 α1 + α2 subdivisions 4 α1 receptor is further divided into α1A, α1B, α1C, and α1D α2 receptor is further divided into α2A, α2B, and α2C. This extended classification is necessary for understanding the selectivity of some drugs. For example, tamsulosin is a selective α1A antagonist that is used to treat benign prostatic hyperplasia (BPH). The drug has fewer cardiovascular side effects because it targets α1A subtype receptors found primarily in the urinary tract and prostate gland and does not affect the α1B subtype found in the blood vessels. 14 β-Adrenoceptors 4 Responses of β receptors differ from those of α receptors and are characterized by a strong response to isoproterenol, with less sensitivity to epinephrine and norepinephrine (Figure 6.4). For β receptors, the rank order of potency is isoproterenol > epinephrine > norepinephrine. S 15 β-Adrenoceptors subdivisions 4 The β-adrenoceptors can be subdivided into three major subgroups, β1, β2, and β3, based on their affinities for adrenergic agonists and antagonists. β1 receptors have approximately equal affinities for epinephrine and norepinephrine, whereas in heart β2 receptors have a higher affinity for epinephrine than for norepinephrine. Thus, tissues with a predominance of β2 receptors (such as the vasculature of skeletal muscle and bronchial smooth muscle) are particularly responsive to the effects of circulating adrenal muedullary epinephrine. β3 receptors are involved in lipolysis and also have relaxation effects on the detrusor muscle of the bladder. Binding of a neurotransmitter at any of the three types of β receptors results in activation of adenylyl cyclase and result in increased concentrations of cAMP within the cell (G-PCRs) 16 Distribution of β- adrenoceptors 4 Adrenergically innervated organs and tissues usually have a predominant type of receptor. For example, tissues such as the vasculature of skeletal muscle have both α1 and β2 receptors, but the β2 receptors predominate. Other tissues may have one type of receptor almost exclusively. For example, the heart contains predominantly β1 receptors. 17 Characteristic responses mediated by 4 adrenoceptors It is useful to organize the physiologic responses to adrenergic stimulation according to receptor type, because many drugs preferentially stimulate or block one type of receptor. Figure 6.6 summarizes the most prominent effects mediated by the adrenoceptors. 18 Characteristic responses mediated by 4 adrenoceptors As a generalization, characteristically: Stimulation of α1 receptors produces vasoconstriction (particularly in skin and abdominal viscera) and an increase in total peripheral resistance and blood pressure. Stimulation of β1 receptors causes cardiac stimulation (increase in heart rate and contractility), whereas stimulation of β2 receptors produces vasodilation (in skeletal muscle vascular beds) and smooth muscle relaxation. β3 receptors are involved in lipolysis and also mediate relaxant effects in the detrusor muscle of the bladder. 19 Desensitization of receptors 4 Prolonged exposure to the catecholamines reduces the responsiveness of these receptors, a phenomenon known as desensitization. Three mechanisms have been suggested to explain this phenomenon: sequestration of the receptors so that they are unavailable for interaction with the ligand; down-regulation that is, a disappearance of the receptors either by destruction or by decreased synthesis; and an inability to couple to G protein, because the receptor has been phosphorylated on the cytoplasmic side (by a kinase). 4. Adrenergic agonists & 4 antagonists 4 I. Adrenergic agonists 3 Desensitization of receptors 4 Prolonged exposure to the catecholamines reduces the responsiveness of these receptors, a phenomenon known as desensitization. Three mechanisms have been suggested to explain this phenomenon: sequestration of the receptors so that they are unavailable for interaction with the ligand; down-regulation that is, a disappearance of the receptors either by destruction or by decreased synthesis; and an inability to couple to G protein, because the receptor has been phosphorylated on the cytoplasmic side (by a kinase). 4 CHARACTERISTICS OF ADRENERGIC AGONISTS 4 Most adrenergic drugs are derivatives of β-phenylethylamine (Figure 6.7). Substitutions on the benzene ring or on the ethylamine side chains produce a variety of compounds with varying abilities to differentiate between α and β receptors and to penetrate the CNS. Two important structural features of these drugs are: the number and location of OH substitutions on the benzene ring and the nature of the substituent on the amino nitrogen. Adrenergic agonists could be catecholamines or noncatecholamines 5 Catecholamines 4 Sympathomimetic amines that contain the 3,4- dihydroxybenzene group (a catechol group to which is attached an amine (nitrogen-containing) group are called catecholamines (CA). Among the catecholamines are: dopamine, epinephrine (adrenaline), norepinephrine (noradrenaline), and isoproterenol (isoprenaline). 6 Common properties of catecholamines (CA) 4 High potency: Catecholamines show the highest potency in directly activating α or β receptors. Rapid inactivation: Catecholamines are metabolized by COMT postsynaptically and by MAO intraneuronally, as well as by COMT and MAO in the gut wall, and by MAO in the liver. Thus, catecholamines have only a brief period of action when given parenterally, and they are inactivated (ineffective) when administered orally. Poor penetration into the CNS: Catecholamines are polar and, therefore, do not readily penetrate into the CNS. Nevertheless, most catecholamines have some clinical effects (anxiety, tremor, and headaches) that are attributable to action on the CNS. 7 Noncatecholamines 4 Compounds lacking the catechol hydroxyl groups have longer half-lives, because they are not inactivated by COMT. Lack of polar hydroxyl groups increases the lipid solubility of many noncatecholamines. This permits greater access to the CNS. These agents are poor substrates for MAO (an important route of metabolism) and, thus, show a prolonged duration of action. These include phenylephrine, ephedrine, amphetamine. 8 Substitutions on the amine nitrogen 4 The nature of the substituent on the amine nitrogen is important in determining β selectivity of the adrenergic agonist. For example, epinephrine, with a –CH3 substituent on the amine nitrogen, is more potent at β receptors than norepinephrine, which has an unsubstituted amine. Similarly, isoproterenol, which has an isopropyl substituent –CH (CH3)2 on the amine nitrogen, is a strong β agonist with little α activity. 9 Mechanism of action of adrenergic agonists 4 Direct-acting agonists: These drugs act directly on α or β receptors, producing effects similar to those that occur following stimulation of sympathetic nerves or release of epinephrine from the adrenal medulla. E.g., include epinephrine, norepinephrine, isoproterenol, dopamine, and phenylephrine. Indirect-acting agonists: These agents may block the reuptake of norepinephrine (e.g., cocaine) or cause the release of norepinephrine from the adrenergic neuron vesicles (e.g., amphetamine). The norepinephrine then traverses the synapse and binds to α or β receptors. 10 Mechanism of action of adrenergic agonists 4 Mixed-action agonists: Ephedrine and its stereoisomer, pseudoephedrine, both stimulate adrenoceptors directly and enhance release of norepinephrine from the adrenergic neuron. 11 Indications and contraindications of adrenergic 4 agonists (sympathomimetics) Sympathomimetics are used in conditions where it is appropriate to raise blood pressure by stimulating the heart and inducing vasoconstriction. They are used for short-term treatment only in Refractory heart failure, Cardiogenic shock, and Hypotension caused by hemorrhage or sepsis Long-term use results in deleterious adverse effects which are generally extensions of their pharmacological activity (that is, stimulation of the sympathetic adrenergic system). 12 Indications and contraindications of adrenergic 4 agonists (sympathomimetics) Overdose with epinephrine or other pressor agents may result in severe hypertension, cardiac arrhythmias due to excessive cardiac stimulation with possible cerebral hemorrhage, and pulmonary edema. Because of these reasons, most of these drugs are high-alert drugs and are only used for short-term cardiovascular therapy with clinical supervision and monitoring. Their long-term use increases mortality in heart failure patients. These drugs are contraindicated in patients with coronary artery disease because of the risk of precipitating myocardial ischemia and angina due to decreased myocardial oxygen supply–demand ratio. -6)m These drugs can also precipitate myocardial infarction and cerebrovascular stroke. 13 post 4 & DIRECT-ACTING ADRENERGIC AGONISTS They bind to adrenergic receptors on effector organs without interacting with the presynaptic neuron. - - This group is widely used in clinical practice. It contains epinephrine, norepinephrine, dopamine which are natural and dobutamine, a synthetic compound. In the adrenal medulla, norepinephrine is methylated to yield epinephrine and upon stimulation, the adrenal medulla releases about 80% epinephrine and 20% norepinephrine directly into the circulation. Epinephrine interacts with both α and β receptors. At low doses, β effects (vasodilation) on the vascular system predominate, whereas at high doses, α effects (vasoconstriction) are the strongest 14 Cardiovascular effects of epinephrine 4 (adrenaline) Epinephrine produces positive inotropic and chronotropic effects mediated via β1 receptor. This will increase in cardiac output (CO) and will increase myocardial oxygen demand. Epinephrine activates β1 receptors on the kidney to cause renin release which is involved in the production of angiotensin II, a potent vasoconstrictor. Epinephrine constricts arterioles in the skin, mucous membranes, and viscera (α effects), and dilates vessels going - to the liver and skeletal muscle (β2 effects). The cumulative effect is an increase in systolic blood pressure, coupled with a slight decrease in diastolic pressure due to β2 receptor–mediated vasodilation in the skeletal muscles 15 Respiratory: Epinephrine causes powerful bronchodilation by acting directly on 4 bronchial smooth muscle (β2 action). It also inhibits the release of allergy mediators such as histamine from mast cells. Hyperglycemia: Epinephrine has a significant hyperglycemic effect because of increased glycogenolysis in the liver (β2 effect), increased release of glucagon (β2 effect), and a decreased release of insulin (α2 effect). Lipolysis: Epinephrine initiates lipolysis through agonist activity on the β3 receptors of adipose tissue. Increased levels of cAMP stimulate a hormone-sensitive lipase, which hydrolyzes triglycerides to free fatty acids and glycerol. 16 Therapeutic uses of epinephrine 4 Bronchospasm: Epinephrine is the primary drug used in emergency conditions when bronchoconstriction has resulted in diminished respiratory function. Within a few minutes after S/C or IM administration, respiratory function greatly improves. Anaphylactic shock: Epinephrine is the drug of choice for the treatment of type I hypersensitivity reactions (including anaphylaxis) in response to allergens (can be life-saving). Cardiac arrest: Epinephrine may be used to restore cardiac rhythm in patients with cardiac arrest. Adjuvant in local anesthesia: Epinephrine greatly increases the duration of local anesthesia by producing vasoconstriction at the site of injection. Epinephrine also reduces systemic absorption of the local anesthetic. Intraocular surgery: Epinephrine is used in the induction and maintenance of mydriasis during intraocular surgery 17 Pharmacokinetics 4 Epinephrine has a rapid onset but a brief duration of action (due to rapid degradation). Intramuscular (IM) route is the preferred route for anaphylaxis in the outpatient setting is due to rapid absorption. Intravenous (IV) epinephrine is given in emergencies, for the most rapid onset of action. Subcutaneous (SC) epinephrine, by the endotracheal tube, and by inhalation may also be given. It is rapidly metabolized by MAO and COMT, and the metabolites metanephrine and vanillylmandelic acid (VMA) are excreted in urine. 18 Adverse effects of epinephrine 4 Epinephrine can produce: CNS effects: anxiety, fear, tension, headache, and tremor. Cardiac arrhythmias or angina: can be triggered particularly if the patient has coronary artery disease (CAD) or hypertension. Pulmonary edema: induced due to increased afterload caused by vasoconstrictive properties of the drug. Afterload, also known as the systemic vascular resistance (SVR), is the amount of resistance the heart must overcome to open the aortic valve and push the blood volume out into the systemic circulation. If you think about the balloon analogy, afterload is represented by the knot at the end of the balloon. 19 Drug interactions 4 Epinephrine may have drug interactions with: Hyperthyroidism: may have an increased production of adrenergic receptors in the vasculature, leading to an enhanced response to epinephrine, and the dose must be reduced in these individuals. Inhalation anesthetics: They sensitize the heart to the effects of epinephrine, which may lead to tachycardia. Diabetes: Because epinephrine increases the release of endogenous stores of glucose, the dose of insulin may have to be increased in diabetic patients. Nonselective β-blockers: They prevent the vasodilatory effects of epinephrine on β2 receptors, leaving α receptor stimulation unopposed. This may lead to increased peripheral resistance and increased blood pressure 20 Norepinephrine 4 Because norepinephrine is the neurotransmitter in adrenergic neurons, it should, theoretically, stimulate all types of adrenergic receptors. However, when administered in therapeutic doses, α-adrenergic receptors are most affected. Cardiovascular actions: Vasoconstriction Baroreceptor reflex 21 Cardiovascular actions of 4 Norepinephrine 1. Vasoconstriction: Norepinephrine causes a rise in peripheral resistance due to intense vasoconstriction of most vascular beds, including the kidney (α1 effect). Both systolic and diastolic blood pressures increase. [Note: Norepinephrine causes greater vasoconstriction than epinephrine, because it does not induce compensatory vasodilation via β2 receptors on blood vessels supplying skeletal muscles. The weak β2 activity of norepinephrine also explains why it is not useful in the treatment of asthma or anaphylaxis.] 22 Cardiovascular actions of 4 Norepinephrine 2. Baroreceptor reflex: Norepinephrine increases blood pressure, and this stimulates the baroreceptors, inducing a rise in vagal activity. The increased vagal activity produces a reflex bradycardia, which is sufficient to counteract the local actions of norepinephrine on the heart, although the reflex compensation does not affect the positive inotropic effects of the drug. When atropine, which blocks the transmission of vagal effects, is given before norepinephrine, stimulation of the heart by norepinephrine is evident as tachycardia. 23 Therapeutic uses of norepinephrine: 4 Treatment of shock (for example, septic shock), because it increases vascular resistance and, therefore, increases blood pressure but, Metaraminol is favored, because it does not reduce blood flow to the kidney Norepinephrine is never used for asthma or in combination with local anesthetics It has no other clinically significant uses. Pharmacokinetics: Norepinephrine is given IV for rapid onset of action. The duration of action is 1 to 2 minutes, following the end of the infusion. It is rapidly metabolized by MAO and COMT, and inactive metabolites are excreted in the urine. 24 Adverse effects of norepinephrine: 4 These are similar to epinephrine. In addition, norepinephrine is a potent vasoconstrictor and may cause blanching and sloughing of skin along an injected vein. It should not be administered in peripheral veins, if possible. If extravasation (leakage of drug from the vessel into tissues surrounding the injection site) occurs, it can cause tissue necrosis. Impaired circulation from norepinephrine may be treated with the α receptor antagonist phentolamine. Alternatives to phentolamine include intradermal terbutaline and topical nitroglycerin. 25 Dopamine 4 Dopamine is the immediate precursor of norepinephrine. It occurs naturally as a neurotransmitter in the CNS- basal ganglia. It occurs also in the adrenal medulla. Dopamine can activate α- and β-adrenergic receptors additional to D1 and D2 dopaminergic receptors. These Dopaminergic receptors are not affected by α- or β-blocking drugs. at higher doses, Dopamine causes vasoconstriction by activating α1 receptors, whereas at lower doses, it stimulates β1 cardiac receptors. D1 and D2 receptors, occur in the peripheral mesenteric and renal vascular beds, where dopamine binding produces vasodilation. D2 receptors are also found on presynaptic adrenergic neurons, where their activation interferes with norepinephrine release. 26 Actions of dopamine 4 Cardiovascular: Dopamine exerts a stimulatory effect on the β1 receptors of the heart, having both positive inotropic and chronotropic effects. At very high doses, dopamine activates α1 receptors on the vasculature, resulting in vasoconstriction. 1. Renal and visceral: Dopamine dilates renal and splanchnic arterioles by activating dopaminergic receptors, thereby increasing blood flow to the kidneys and other viscera. The increased blood flow to the kidney enhances the glomerular filtration rate and causes diuresis. Dopamine is superior to norepinephrine that diminish blood supply to the kidney and may reduce renal function. 27 Therapeutic uses: Dopamine can be used for: 4 cardiogenic and septic shock and is given by continuous infusion. hypotension, severe heart failure, and bradycardia unresponsive to other therapy. Dopamine raises blood pressure: by stimulating β1 receptors on the heart to increase cardiac output and α1 receptors on blood vessels to increase total peripheral resistance. Adverse effects: An overdose of dopamine produces the same effects as sympathetic stimulation. Dopamine is rapidly metabolized by MAO or COMT, and its adverse effects (nausea, hypertension, and arrhythmias) are, therefore, short-lived. 28 Phenylephrine 4 Phenylephrine is a direct-acting, synthetic noncatechol adrenergic drug that binds primarily to α1 receptors. It is a vasoconstrictor that raises both systolic and diastolic blood pressures. The drug is used to treat hypotension in hospitalized or surgical patients (especially those with a rapid heart rate). Phenylephrine acts as a nasal decongestant when applied topically or taken orally. Phenylephrine is also used in ophthalmic solutions for mydriasis. 29 Phenylephrine 4 Phenylephrine has no effect on the heart itself but, rather, induces reflex bradycardia when given parenterally, making it useful in treating paroxysmal supraventricular tachycardia. Large doses can cause hypertensive headache and cardiac irregularities. Although data suggest it may not be as effective, phenylephrine has replaced pseudoephedrine in many oral decongestants, since pseudoephedrine has been misused to make methamphetamine. 30 Naphazoline, oxymetazoline, and 4 tetrahydrozoline These drugs are direct-acting synthetic adrenergic agonist that stimulates both α1- and α2-adrenergic receptors. They directly stimulates α receptors on blood vessels supplying the nasal mucosa and conjunctiva, thereby producing vasoconstriction and decongestion. They are found in many OTC nasal decongestants, as well as in ophthalmic drops for the relief of red eyes associated with swimming, colds, and contact lenses. Oxymetazoline is absorbed systemically and may produce nervousness, headaches, and trouble sleeping. Local irritation and sneezing may occur with intranasal administration of these drugs. Use for greater than 3 days is not recommended, as rebound congestion and dependence may occur (rhinitis medicamentosa) 31 Midodrine 4 Midodrine is a prodrug, is metabolized to the pharmacologically active desglymidodrine. It is a selective α1 agonist, which acts in the periphery to increase arterial and venous tone. Midodrine is indicated for the treatment of orthostatic hypotension. The drug should be given three times daily, with doses at 3- or 4-hour intervals. To avoid supine hypertension, doses within 4 hours of bedtime are not recommended. 32 Clonidine 4 Clonidine is an α2 agonist used for: The treatment of hypertension. To minimize symptoms of withdrawal from opiates, tobacco smoking, and benzodiazepines. In the management of attention-deficit hyperactivity disorder (ADHD). Clonidine acts centrally on presynaptic α2 receptors to produce inhibition of sympathetic vasomotor centers, decreasing sympathetic outflow to the periphery. 33 Clonidine Side effects 4 The most common side effects of clonidine are: orthostatic hypotension, impotence, lethargy, sedation, constipation, xerostomia, and bradycardia (because of increased vagal stimulation of the SA node as well as sympathetic withdrawal). Fluid retention and edema are problems with chronic therapy; therefore, concurrent therapy with a diuretic is necessary. Abrupt discontinuation must be avoided to prevent rebound hypertension. 34 Dobutamine 4 Dobutamine is a synthetic, direct-acting catecholamine that is primarily a β1 receptor agonist with minor β2 and α1 effects. It increases heart rate and cardiac output with few vascular effects. It does not elevate myocardial oxygen demands as much as other sympathomimetic drugs. Dobutamine is used to: increase cardiac output in acute heart failure inotropic support after cardiac surgery. Dobutamine should be used with caution in atrial fibrillation, because it increases atrioventricular (AV) conduction. Other adverse effects are similar to epinephrine. Tolerance may develop with prolonged use. 35 Isoproterenol 4 Isoproterenol (isoprenaline) is a direct-acting synthetic nonselective catecholamine that stimulates both β1- and β2-adrenergic receptors. Its action on α receptors is insignificant. Isoproterenol produces intense stimulation of the heart (β1 effect), increasing heart rate, contractility, and cardiac output. It is as active as epinephrine in this action. Isoproterenol also dilates the arterioles of skeletal muscle (β2 effect), resulting in decreased peripheral resistance. Because of its cardiac stimulatory action, it increases systolic blood pressure slightly, but it greatly reduces mean arterial and diastolic blood pressures. It is also a potent bronchodilator (β2 effect). 36 Therapeutic indications of isoproterenol 4 Because of its nonselectivity and side effects on the heart, isoproterenol is rarely used therapeutically. It is no longer used to treat bronchial asthma. It is used to treat cardiotoxic shock, cardiac arrest and severe bradycardia It is also used to reverse heart block produced by overdose of β blockers Adverse effects of isoproterenol are: Palpitations and Cardiac arrhythmias 37 Albuterol, levalbuterol, metaproterenol, and 4 terbutaline Albuterol (salbutamol), levalbuterol, metaproterenol and terbutaline are short-acting β2 agonists (SABAs): They are used primarily as bronchodilators and administered by a metered-dose inhaler. Albuterol and levalbuterol are the SABAs of choice for the management of acute bronchospasm because these agents are more selective for β2 receptors than metaproterenol. Inhaled terbutaline: still used in some countries. Injectable terbutaline is used for: The reversal of bronchospasm Off-label as a uterine relaxant to suppress premature labor. 38 Albuterol, levalbuterol, metaproterenol, and 4 terbutaline One of the most common side effects of these agents is tremor, but patients tend to develop tolerance to this effect. Other side effects include restlessness, apprehension, and anxiety. When these drugs are administered orally, they may cause tachycardia or arrhythmia (due to β1 receptor activation), especially in patients with underlying cardiac disease. Concomitant use of Monoamine oxidase inhibitors (MAOIs) with SABAs increase the risk of adverse cardiovascular effects and should be avoided. Use of nonselective β antagonists can diminish or counteract the bronchodilatory effects of SABAs. 39 Formoterol, indacaterol, olodaterol, and 4 salmeterol Formoterol, arformoterol, indacaterol, olodaterol and salmeterol are selective long- acting β2 agonists (LABAs) used for the management of respiratory disorders such as asthma and chronic obstructive pulmonary disease A single dose by a metered dose inhaler device, provides sustained bronchodilation over 12 hours, compared with less than 3 hours for albuterol. LABAs are not recommended as monotherapy for the treatment of asthma, because they have been shown to increase the risk of asthma-related deaths; however, these agents are highly efficacious when combined with an asthma controller medication such as an inhaled corticosteroid. Unlike formoterol, salmeterol has a somewhat delayed onset of action (because of its high lipophilicity). 40 Mirabegron and vibegron 4 Mirabegron and vibegron are β3 agonist that relaxes the detrusor smooth muscle and increases bladder capacity. They are used for patients with overactive bladder. Mirabegron may increase blood pressure and should not be used in patients with uncontrolled hypertension. Both drugs increase levels of digoxin by inhibiting P-gp mediated elimination Mirabegron inhibits the CYP2D6 isozyme, which may enhance the effects of other medications metabolized by this pathway (for example, metoprolol). Vibegron has minimal interaction with the CYP450 enzyme system and its potential for drug interactions is less than mirabegron 41 INDIRECT-ACTING ADRENERGIC AGONISTS 4 Indirect-acting adrenergic agonists: cause the release of epinephrine or norepinephrine, or inhibit the reuptake of epinephrine or norepinephrine , or inhibit the degradation of epinephrine or norepinephrine. They potentiate the effects of epinephrine or norepinephrine produced endogenously, but, They do not directly affect postsynaptic receptors. This group includes following drugs: Amphetamine, Tyramine, Cocaine 42 Amphetamine 4 Amphetamine is an indirect-acting adrenergic drug that has a marked central stimulatory action. It is a drug of abuse. Amphetamine significantly increase blood pressure by α1 agonist action on the vasculature, as well as β1-stimulatory effects on the heart. Its actions are mediated primarily through an increase in non-vesicular release of catecholamines such as dopamine and norepinephrine from nerve terminals. This mechanism is complimented by: 1. inhibition of reuptake of these catecholamines 2. by inhibition of monoamine oxidase (MAO) Therapeutic uses are limited to Attention deficit hyperactivity disorder (ADHD), narcolepsy and appetite suppression. 43 Tyramine 4 Tyramine is not a clinically useful drug, but it is important because it is found in fermented foods, such as aged cheese and some wine. It is a normal by-product of tyrosine metabolism. Normally, it is oxidized by MAO in the G.I.T., but, if the patient is taking MAOIs, it can precipitate serious vasopressor episodes. Like amphetamines, tyramine can enter the nerve terminal and displace stored norepinephrine. The released catecholamine then acts on adrenoceptors. 44 Cocaine 4 Cocaine is a unique local anesthetic. It blocks the sodium-chloride (Na+/Cl−)-dependent norepinephrine transporter required for cellular uptake of norepinephrine into the adrenergic neuron. Consequently, norepinephrine accumulates in the synaptic space, resulting in enhanced sympathetic activity and potentiation of epinephrine and norepinephrine effects. Therefore, in an individual taking cocaine, small doses of the catecholamines produce greatly magnified effects In addition, the duration of action of epinephrine and norepinephrine is increased. Like amphetamines, it can increase blood pressure by α1 agonist actions and β stimulatory effects. 45 MIXED-ACTION ADRENERGIC AGONISTS 4 Ephedrine and pseudoephedrine are mixed-action adrenergic agents. They: Enhance release of stored norepinephrine from nerve endings (presynaptic) Directly stimulate both α and β receptors (postsynaptic) Ephedrine and pseudoephedrine are: Not catecholamines and are Poor substrates for COMT and MAO. Therefore, these drugs have a long duration of action. Ephedrine and pseudoephedrine have excellent absorption after oral administration and penetrate the CNS, but pseudoephedrine has fewer CNS effects. 46 MIXED-ACTION ADRENERGIC AGONISTS 4 Ephedrine is eliminated largely unchanged in urine, and pseudoephedrine undergoes incomplete hepatic metabolism before elimination in urine. Ephedrine raises systolic and diastolic blood pressures by vasoconstriction and cardiac stimulation, and it is indicated in anesthesia-induced hypotension. Ephedrine produces bronchodilation, but it is less potent and slower acting than epinephrine or isoproterenol. It was previously used to prevent asthma attacks but has been replaced by more effective medications. Ephedrine produces a mild stimulation of the CNS. This increases alertness, decreases fatigue, and prevents sleep. It also improves athletic performance. 47 MIXED-ACTION ADRENERGIC AGONISTS 4 [Ephedrine-containing herbal supplements (mainly ephedra- containing products) have been banned by most drug regulatory authorities because of life-threatening cardiovascular reactions.] Oral pseudoephedrine is primarily used to treat nasal and sinus congestion. Pseudoephedrine has been illegally used to produce methamphetamine. Therefore, products containing pseudoephedrine have certain restrictions in some countries. 4 4 4. Adrenergic agonists 4 & antagonists blocker 4 OR inhibitions II. Adrenergic antagonists 3 OVERVIEW 4 The adrenergic antagonists (also called adrenergic blockers or sympatholytics): bind to adrenoceptors but do not trigger the usual receptor-mediated intracellular effects. These drugs attach to the adrenoceptors either: tention reversibly or hyper irreversibly, thus, preventing activation by endogenous or exogenous agonists. Like the agonists, the adrenergic antagonists are classified according to their relative affinities for α or β receptors in the sympathetic nervous system. 4 OVERVIEW 4 Adrenergic antagonists have varying degrees of specificity and are therefore classified into five classes: 1) nonselective adrenergic antagonists, 2) nonselective α-adrenergic antagonists 3) nonselective β-adrenergic antagonists, 4) selective α1-adrenergic antagonists 5) selective β1-adrenergic antagonists. Numerous adrenergic antagonists have important roles in clinical medicine, primarily to treat diseases associated with the cardiovascular system. 5 α-ADRENERGIC BLOCKING AGENTS 4 α-Adrenergic blocking agents antagonize the subtype(s) of α-adrenergic receptors (α1 or α2), depending on the specificity of the agent for the receptor subtype(s). Drugs that block α1 adrenoceptors profoundly affect blood pressure. Because normal sympathetic control of the vasculature occurs in large part through agonist actions on α1-adrenergic receptors, blockade of these α1-receptors reduces the sympathetic - tone of the blood vessels, resulting in decreased peripheral vascular resistance. = This lowered blood pressure induces reflex tachycardia. The magnitude of the response depends on the sympathetic tone of the individual when the agent is given. Selective α2-adrenergic blockers have limited clinical utility. 6 Phenoxybenzamine 4 Phenoxybenzamine is a nonselective, irreversible, noncompetitive blocker of α1- and α2-adrenergic receptors. Phenoxybenzamine binds covalently to both α1 & α2 The body can overcome the block by synthesis of the new adrenoceptors, which requires a day or longer. Therefore, the action of phenoxybenzamine last about 24 hours. biotransformation to the active form is delayed for a few hours, 7 Actions of phenoxybenzamine A. Cardiovascular effects. vasodilatation 4 - The drug prevents α1 receptor mediated vasoconstriction of peripheral blood vessels caused by endogenous catecholamines. This leads to decreased peripheral resistance and reflex tachycardia. However, by blocking presynaptic α2 receptors on the sympathetic nerve terminals in the heart, phenoxybenzamine causes an increase in the release of norepinephrine, which in turn increases heart rate and cardiac output (mediated by β1 receptors). This may also lead to cardiac arrhythmias and anginal pain. Thus, the drug has been unsuccessful in maintaining lowered blood pressure in hypertension, and it is no longer used for this purpose. 8 B. Epinephrine reversal 4 All α-adrenergic blockers reverse the α agonist actions of epinephrine. For example, the vasoconstrictive action of epinephrine is interrupted by α- adrenergic blockers, but vasodilation of other vascular beds caused by stimulation of β2 receptors is not blocked. Therefore, in the presence of phenoxybenzamine, the systemic blood pressure decreases in response to epinephrine. [Note: The actions of norepinephrine are not reversed but are diminished because norepinephrine lacks significant β agonist action on the vasculature.] Phenoxybenzamine has no effect on the actions of isoproterenol, which is a pure β agonist (Figure 7.2). 9 4 10 Therapeutic uses of phenoxybenzamine 4 Treatment of pheochromocytoma, a catecholamine-secreting tumor of cells derived from the adrenal medulla. Treatment of Raynaud disease and frostbite. 11 Adverse effects of phenoxybenzamine 4 Phenoxybenzamine can cause: postural hypotension, nasal stuffiness, nausea and vomiting. It may inhibit ejaculation. It may also induce reflex tachycardia, which is mediated by the baroreceptor reflex. Phenoxybenzamine should be used with caution in patients with cerebrovascular or cardiovascular disease. 12 Phentolamine 4 In contrast to phenoxybenzamine, phentolamine produces a competitive block of α1 and α2 receptors. Effects last for approximately 4 hours after a single injection. Pharmacological effects of phentolamine are very similar to those of phenoxybenzamine. Uses of phentolamine: For the diagnosis and short-term management of pheochromocytoma. Prevention of dermal necrosis following extravasation of norepinephrine. Treatment of hypertensive crisis. In dental procedures, local injection can reverse anesthesia of soft tissues (lip and tongue) produced by injection of local anesthesia containing a vasoconstrictor. 13 Prazosin, terazosin, doxazosin, 4 Tamsulosin, alfuzosin, silodosin Prazosin, terazosin, and doxazosin are selective competitive blockers of the α1 receptors. They are useful in the treatment of hypertension but not as monotherapy. Tamsulosin, alfuzosin, and silodosin are other selective α1 antagonists. They are indicated for the treatment of benign prostatic hyperplasia (BPH)] An additive antihypertensive effect occurs when α1 antagonists are given with vasodilators such as nitrates or PDE-5 inhibitors (for example, sildenafil), requiring cautious dose titration and use at the lowest possible doses. 14 Prazosin, terazosin, doxazosin, 4 Tamsulosin, alfuzosin, silodosin Individuals with elevated blood pressure treated with one of these drugs do not become tolerant to its action. However, the first dose of these drugs may produce an exaggerated orthostatic hypotensive response that can result in syncope (fainting). This action, termed a “first-dose” effect, may be minimized by adjusting the first dose to one-third or one-fourth of the normal dose and by giving the drug at bedtime. Doxazosin is the longest acting of these drugs. Metabolism leads to inactive products excreted in urine except for metabolites of doxazosin, which appear in feces. 15 Mechanism of action 4 These agents decrease peripheral vascular resistance and lower blood pressure by causing relaxation of both arterial and venous smooth muscle. Tamsulosin, alfuzosin, and silodosin have less pronounced effects on blood pressure because they are: less selective for α1B receptors found in the blood vessels and more selective for α1A receptors found in the prostate and bladder. Blockade of the α1A receptors decreases tone in the smooth muscle of the bladder neck and prostate and improves urine flow. 16 Adverse effects 4 α1-Blockers may cause dizziness, lack of energy, nasal congestion, headache, drowsiness, orthostatic hypotension (although to a lesser degree than that observed with phenoxybenzamine and phentolamine). Reduced ejaculation (due to reduced vasoconstriction Increased risk of congestive heart failure (CHF) when α1- receptor blockers used as monotherapy in hypertension 17 β-ADRENERGIC BLOCKING AGENTS 4 All clinically available β-blockers are competitive antagonists. Nonselective β-blockers act at both β1 and β2 receptors, whereas cardioselective β antagonists primarily block β1 receptors. [Note: There are no clinically useful β2 selective antagonists.] These drugs also differ in: intrinsic sympathomimetic activity, CNS effects, vasodilation, and pharmacokinetics. 18 β-ADRENERGIC BLOCKING AGENTS 4 Although all β-blockers lower blood pressure, they do not induce postural hypotension, because the α adrenoceptors remain functional. Therefore, normal sympathetic control of the vasculature is maintained. β-Blockers are effective in treating: systemic as well as portal hypertension, angina, cardiac arrhythmias, myocardial infarction, heart failure, hyperthyroidism, Note: Glaucoma, and The names of all β-blockers end in “-olol” except for: prophylaxis of migraine headaches. labetalol carvedilol. 19 Propranolol: A nonselective β antagonist 4 Propranolol is the prototype β-adrenergic antagonist and blocks both β1 and β2 receptors with equal affinity. Nonselective β-blockers, including propranolol, have the ability to block the actions of isoproterenol (β1, β2 agonist) on the cardiovascular system. Thus, in the presence of a β-blocker, isoproterenol does not produce cardiac stimulation (β1 mediated) or reductions in mean arterial pressure and diastolic pressure (β2 mediated). 20 Propranolol: A nonselective β antagonist 4 Note: In the presence of a nonselective β-blocker, epinephrine no longer lowers diastolic blood pressure or stimulates the heart, but its vasoconstrictive action (mediated by α receptors) remains unimpaired. The actions of norepinephrine on the cardiovascular system are mediated primarily by α receptors and are, therefore, mostly unaffected.] 21 Actions of propranolol 4 a. Cardiovascular: Propranolol reduces cardiac output, having negative inotropic and chronotropic effects. Cardiac output, workload, and oxygen consumption are decreased by blockade of β1 receptors, and these effects are useful in the treatment of angina. β-blockers attenuate the expected increase in heart rate during exercise or stress, when the sympathetic nervous system is activated. It directly depresses SA and AV nodal activity. Resulting bradycardia limits the drug dose. β-blockers are effective in supraventricular arrhythmias and exercise-induced ventricular arrhythmias. 22 Actions of propranolol 4 b. Peripheral vasoconstriction: Nonselective blockade of β receptors prevents β2-mediated vasodilation in skeletal muscles, leading to: increasing peripheral vascular resistance. The reduction in cardiac output produced by all β-blockers leads to decreased blood pressure, which triggers a reflex peripheral vasoconstriction that is reflected in reduced blood flow to the periphery. There is a gradual reduction of both systolic and diastolic blood pressures in hypertensive patients. No postural hypotension occurs because (α1 is not affected) 23 Actions of propranolol 4 c. Bronchoconstriction: Blocking β2 receptors in the lungs of susceptible patients causes contraction of the bronchiolar smooth muscle. This can precipitate an exacerbation in patients with chronic obstructive pulmonary disease (COPD) or asthma. Therefore, β-blockers, particularly nonselective ones, are contraindicated in patients with asthma and should be avoided in COPD. 24 Actions of propranolol 4 d. Disturbances in glucose metabolism: β Blockade leads to: decreased glycogenolysis decreased glucagon secretion. Therefore, if propranolol is given to a diabetic patient receiving insulin, careful monitoring of blood glucose is essential, because pronounced hypoglycemia may occur after insulin injection. β-Blockers also attenuate the normal physiologic response to hypoglycemia (increased heart rate, cardiac output and systolic blood pressure, tremors, anxiety, palpitations, sweating). [Note: Diaphoresis with hypoglycemia still occurs, as this is mediated through the neurotransmitter acetylcholine.] 25 Therapeutic uses 4 a. Hypertension: Propranolol lowers blood pressure in hypertension by several different mechanisms of action. Decreased cardiac output is the primary mechanism, Following mechanisms also contribute to the antihypertensive effects. 1. inhibition of renin release from the kidney, 2. decrease in total peripheral resistance with long- term use, and 3. decreased sympathetic outflow from the CNS 26 4 b. Glaucoma β-blockers reduce intraocular pressure by reducing the secretion of aqueous humor. Topical timolol maleate is 5 times more potent than propranolol in this regards c. Angina pectoris: Propranolol is useful in the management of chronic stable angina. Propranolol decreases the oxygen requirement of heart muscle and, therefore, is effective in reducing chest pain on exertion that is common in angina. 27 d. Myocardial infarction: Propranolol and other β-blockers have a protective effect on the 4 myocardium. Thus, patients who have had one myocardial infarction seem to be protected against a second heart attack by prophylactic use of β-blockers. In addition, administration of a β-blocker immediately following a myocardial infarction reduces infarct size and early mortality. The mechanism for these effects may be a reduction in the actions of circulating catecholamines that increase the oxygen demand in an already ischemic heart muscle. Propranolol also reduces the incidence of sudden arrhythmic death after myocardial infarction. 28 e. Migraine: Propranolol is effective in reducing migraine episodes when used 4 prophylactically. It is one of the more useful β-blockers for this indication, due to its lipophilic nature that allows it to penetrate the CNS. [Note: For the acute management of migraine, serotonin agonists such as sumatriptan are used, as well as other drugs.] f. Hyperthyroidism: Propranolol and other β-blockers are effective in blunting the widespread sympathetic stimulation that occurs in hyperthyroidism. In acute hyperthyroidism (thyroid storm), β-blockers may be lifesaving in protecting against serious cardiac arrhythmias 29 Pharmacokinetics 4 After oral administration, propranolol is almost completely absorbed. It is subject to first-pass effect, and only about 25% of an administered dose reaches the circulation. The volume of distribution of propranolol is quite large (4 L/kg), and the drug readily crosses the blood–brain barrier due to its high lipophilicity. Propranolol is extensively metabolized, and most metabolites are excreted in the urine. 30 Adverse effects of propranolol 4 a. Bronchoconstriction: Propranolol has the potential to cause significant bronchoconstriction due to blockade of β2 receptors. Death by asphyxiation has been reported for patients with asthma who were administered the drug. Therefore, propranolol is contraindicated in patients with COPD or asthma. 31 Adverse effects of propranolol 4 b. Arrhythmias: Treatment with β-blockers must never be stopped abruptly because of the risk of precipitating cardiac arrhythmias, which may be severe. The β-blockers must be tapered off gradually over a period of at least a few weeks. Long-term treatment with a β antagonist leads to up-regulation of the β receptor and on the suspension of therapy: the increased receptors can precipitate worsened angina or hypertension through action of endogenous catecholamines on the up-regulated β receptors. 32 4 c. Sexual impairment: Impaired sexual activity (erectile dysfunction, ED) has been reported in male patients taking propranolol. The reasons for this are not clear It was proposed that inhibition of the sympathetic nervous system, which is involved in the integration of erection, emission and ejaculation may play a role. However, β blockers do not affect ejaculation (mediated by α receptors). d. CNS effects: Propranolol has numerous CNS-mediated effects, including depression, dizziness, lethargy, fatigue, weakness, visual disturbances, hallucinations, short-term memory loss, emotional lability, vivid dreams (including nightmares), and depression. Fewer CNS effects may be seen with more hydrophilic β-blockers (for example, atenolol) because they do not cross the blood–brain barrier as readily. 33 e. Metabolic disturbances: β Blockade leads to decreased glycogenolysis and decreased glucagon 4 secretion. Fasting hypoglycemia may occur. In addition, β-blockers can blunt the perception of symptoms of hypoglycemia such as tremor, tachycardia, and nervousness. Patients administered nonselective β-blockers have increased LDL-C, increased triglycerides, and reduced HDL cholesterol) through β2 blockade. These effects may be less pronounced with the use of β1- selective antagonists such as metoprolol. Note: β receptors mobilize energy molecules such as free fatty acids. Lipases are activated by β2 and β3 receptor stimulation, leading to metabolism of triglycerides to free fatty acids 34 Drug interactions: 4 Propranolol is metabolized in the liver mainly by CYP 1A2 and CYP 2D6 enzymes. Drugs that interfere with, or inhibit, the metabolism of propranolol, such as cimetidine, fluoxetine, paroxetine, and ritonavir, may potentiate its antihypertensive effects. Conversely, those that stimulate or induce its metabolism, such as barbiturates, phenytoin, and rifampin, can decrease its effects. Nonselective β-blockers such as propranolol may prevent the bronchodilatory effects of β2 agonists in asthma and the rescue effects of epinephrine in anaphylaxis. 35 Nonselective β antagonists Nadolol and timolol: 4 Nadolol and timolol also block β1- and β2-adrenoceptors and are more potent than propranolol. Nadolol has a very long duration of action. Timolol reduces the production of aqueous humor in the eye. It is used topically in the treatment of chronic open-angle glaucoma. 36 β-Blockers in the treatment of glaucoma 4 β-Blockers, such as topically applied timolol, are effective in diminishing intraocular pressure (IOP) in glaucoma. This occurs by decreasing the secretion of aqueous humor by the ciliary body. Carteolol, and levobunolol are nonselective β antagonists, whereas betaxolol is a β1-selective agent. Unlike the cholinergic drugs, these agents neither affect the ability of the eye to focus for near vision nor change pupil size. When administered intraocularly, the onset is about 30 minutes, and the effects last for 12 to 24 hours. The β-blockers are only used for chronic management of glaucoma. In an acute attack of glaucoma, pilocarpine is still the drug of choice for emergency lowering of IOP. 37 β-Blockers in the treatment of portal 4 hypertension Nonselective β-Blockers, such as nadolol and propranolol are used in the treatment of portal hypertension in patients with cirrhosis. Treatment with these agents reduces the risk of variceal hemorrhage. 38 Selective β1 antagonists 4 Acebutolol, atenolol, betaxolol, bisoprolol, esmolol, metoprolol, and nebivolol are selective β1 antagonists Drugs that preferentially block the β1 receptors minimize the unwanted bronchoconstriction (β2 effect) seen with the use of nonselective agents in asthma patients. Cardioselective β blockers, such as acebutolol, atenolol, and metoprolol, antagonize β1 receptors at doses 50- to 100-fold less than those required to block β2 receptors. This cardioselectivity is most pronounced at low doses and is lost at high doses. [Note: Because β1 selectivity of these agents is lost at high doses, they may antagonize β2 receptors.] 39 4 These drugs lower blood pressure in hypertension and increase exercise tolerance in angina. Esmolol (available as IV only), with a rapid onset of action of 90 seconds, has a very short duration of action (half-life of only 9 to 10 minutes) due to rapid hydrolysis by red blood cell esterase. It is used to control blood pressure or heart rhythm in critically ill patients. Esmolol can be used safely in patients with bronchospastic and vascular disease but still asthmatics need to be carefully monitored. Nebivolol (cardioselective β-blocker) releases nitric oxide from endothelial cells and causes vasodilation. Cardioselective β-blockers have fewer effects on pulmonary function, peripheral resistance, and carbohydrate metabolism. Because these drugs have less effect on peripheral vascular β2 receptors, coldness of extremities (Raynaud phenomenon), a common side effect of β- blockers, is less frequent. 40 Therapeutic uses of selective β1 antagonists 4 The cardioselective β-blockers are useful in hypertensive patients with impaired pulmonary function. These agents are also first-line therapy for chronic stable angina. Bisoprolol and the extended-release formulation of metoprolol are indicated for the management of chronic heart failure. 41 Antagonists with partial agonist activity 4 Acebutolol (β1-selective antagonist) and pindolol (nonselective β- blocker) are not pure antagonists. These drugs can also weakly stimulate both β1 and β2 receptors and are said to have intrinsic sympathomimetic activity (ISA). These partial agonists stimulate the β receptor to which they are bound, yet they inhibit stimulation by the more potent endogenous catecholamines, epinephrine and norepinephrine. The result of these opposing actions is a diminished effect on reduction of cardiac rate and cardiac output compared to that of β-blockers without ISA. X 42 Antagonists with partial agonist activity 4 β-Blockers with ISA minimize the disturbances of lipid and carbohydrate metabolism seen with other β-blockers. For example, these agents do not decrease plasma HDL levels. Therapeutic use: β-Blockers with ISA are effective in hypertensive patients with moderate bradycardia because a further decease in heart rate is less pronounced with these drugs. [Note: β-Blockers with ISA are not used for stable angina or arrhythmias due to their partial agonist effect.] Overall, β-blockers with ISA are infrequently used in clinical practice. 43 Antagonists of both α and β adrenoceptors 4 Labetalol and carvedilol are nonselective β-blockers with concurrent α1- blocking actions that produce peripheral vasodilatation thereby reducing blood pressure (no reflex tachycardia). They contrast with the other β-blockers that produce initial peripheral vasoconstriction and these agents are therefore useful in treating hypertensive patients for whom increased peripheral vascular resistance is undesirable. Carvedilol also decreases lipid peroxidation and vascular wall thickening, effects that have benefit in heart failure. Lipid peroxidation is the chain of reactions of oxidative degradation of lipids. It is the process in which free radicals "steal" electrons from the lipids in cell membranes, resulting in cell damage. 44 Therapeutic use in hypertension and heart failure 4 Labetalol is used as an alternative to methyldopa in the treatment of pregnancy- induced hypertension. Intravenous labetalol is also used to treat hypertensive emergencies, because it can rapidly lower blood pressure particularly in patients with an elevated heart rate. β-blockers should not be used in acute exacerbation of heart failure, as they can worsen the condition. However, carvedilol as well as metoprolol and bisoprolol are beneficial in patients with stable chronic heart failure. These agents work by blocking the effects of sympathetic stimulation on the heart, which causes worsening heart failure over time. Orthostatic hypotension and dizziness are associated with α1 blockade Summary of β-adrenergic antagonists. 4 antagonists & Y Chapter- 2 Pharmacokinetics & Pharmacodynamics 2 2 Branches of pharmacology Pharmacology comprises two main branches: 1. Pharmacokinetics and 2. Pharmacodynamics. 3 2 Pharmacokinetics 1. Pharmacokinetics (what the body does to drugs): The study of what happens to the drug in the body is called pharmacokinetics. E.g., Chlorpromazine is absorbed at a faster rate by the parenteral route than the oral route; it binds with plasma and tissue protein, it is metabolized in the liver and is excreted in 15 to 30 hours. 4 2 Overview of Pharmacokinetics Pharmacokinetic properties determine the onset, intensity, and duration of drug action: 1. Absorption, 2. Distribution, These properties are abbreviated as 3. Metabolism, (ADME) 4. Elimination Knowledge of pharmacokinetic parameters, helps clinicians to design optimal drug regimens, including the route of administration, dose, frequency, and duration of treatment. 5 2 Routes of drug administration There are two major routes of drug administration, Enteral Parenteral 1) injectables 2) Miscellaneous Each route has advantages and disadvantages Routes of administration of a drug should be safe and effective 6 2 Enteral route of drug administration Enteral administration (administering a drug by mouth) is the most common, convenient, and economical method of drug administration. The drug may be swallowed, allowing oral delivery, or it may be placed under the tongue (sublingual) or between the gums and cheek (buccal), facilitating direct absorption into the bloodstream. 7 2 Oral route of administration Oral administration is the most convenient dosage form and provides many advantages. 1. Oral drugs are easily self-administered, and 2. Toxicities and/or overdose of oral drugs may be overcome with antidotes, such as activated charcoal. However, the pathways involved in oral drug absorption are the most complicated, and the low gastric pH inactivates some drugs. A wide range of oral preparations is available including enteric coated and extended-release preparations. 8 2 Parenteral route of drug administration The parenteral route Introduces drugs directly into the systemic circulation and provides the most control over the dose of drug delivered to the body. Parenteral route is used for: Drugs that are poorly absorbed from the GI tract (for example, heparin) or Drugs that are unstable in the GI tract e.g., insulin. Patients unable to take oral medications and When rapid onset of action is required. However, This route is irreversible and may cause pain, fever, local tissue damage, and infections. 9 Parenteral route of drug 2 administration The three major injectable parenteral routes are: 1. Intravenous, 2. intramuscular, 3. subcutaneous Other injectable parenteral routes: Intradermal, intra-arterial, intraperitoneal, intra- articular, intravesical, intracardiac 10 2 Other Parenteral routes 1. Oral inhalation and nasal preparations 2. Intrathecal/intraventricular 3. Topical 4. Transdermal 5. Rectal 11 2 Absorption of drugs Absorption is the transfer of a drug from the site of administration to the bloodstream. Factors influencing absorption include: pH at the absorption site. Blood flow at the absorption site Total surface available for absorption Contact time at absorption surface Expression of P-glycoprotein 12 Mechanisms of absorption of 2 drugs from the GI tract Depending on their chemical properties, drugs may be absorbed from the GI tract by: 1. passive diffusion, 2. facilitated diffusion, 3. active transport, or 4. endocytosis 13 Mechanisms of absorption of 2 drugs from the GI tract 14 2 Bioavailability Bioavailability is the rate and extent to which an administered drug reaches the systemic circulation. For example, if 100 mg of a drug is administered orally and 70 mg is absorbed unchanged, the bioavailability is 0.7% or 70%. 15 2 Determination of bioavailability Bioavailability is determined by comparing plasma levels of a drug after a particular route of administration (for example, oral administration) with the levels achieved after IV administration. 16 2 After IV administration, 100% of the drug rapidly enters the circulation. When the drug is given orally, only part of the administered dose appears in the plasma. By plotting plasma concentrations of the drug versus time, the area under the curve (AUC) can be measured. 17 2 Drug distribution Drug distribution is the process by which a drug reversibly leaves the bloodstream and enters the extracellular fluid and tissues. For drugs administered IV, absorption is not a factor, and the initial phase immediately following administration represents the distribution phase, during which the drug rapidly leaves the circulation and enters the tissues. 18 2 Factors affecting drug distribution 1. Cardiac output Cardiac output affects drug distribution by regulating blood flow to tissues. In clinical practice, understanding the impact of cardiac output on drug distribution is crucial. Conditions that affect cardiac output (e.g., heart failure, shock) can significantly alter the pharmacokinetics and pharmacodynamics of drugs. Adjustments in drug dosing may be necessary in patients with altered cardiac output to achieve therapeutic efficacy and avoid potential toxicity. 2. Local blood flow, blood flow to “vessel-rich organs” (brain, liver, and kidney) is greater than that to the skeletal muscles. Adipose tissue, skin, and viscera have still lower rates of blood flow. 19 2 3. Capillary permeability, Capillary permeability determines how easily drugs can pass from the bloodstream into tissues. Higher permeability allows drugs to penetrate tissues more readily, affecting their distribution throughout the body and affect the extent to which drugs accumulate in specific organs or regions. Organs with fenestrated capillaries (e.g., liver, kidneys) may accumulate drugs more than tissues with tighter endothelial junctions (e.g., brain). In the brain, the capillary structure is continuous, and there are no slit junctions (they form BBB). Ionized or polar drugs generally fail to enter the CNS because they cannot pass through the endothelial cells that have no slit junctions 20 2 4. Degree of binding of the drug to plasma and tissue proteins, Many drugs bind to plasma proteins in the bloodstream. Albumin is the major drug-binding protein, and it may act as a drug reservoir. Only free (unbound) drug molecules are pharmacologically active and can distribute into tissues. Drugs that are highly protein-bound have a lower fraction of free drug available for distribution into tissues. This can limit their ability to reach therapeutic targets unless there is a mechanism to release them from protein binding. Some drugs bind extensively to tissues after distribution from the bloodstream. Tissue binding can affect the distribution of drugs by sequestering them within tissues or prolonging their presence in specific organs. This tissue reservoirs may serve as a major source of the drug and prolong its actions or cause local drug toxicity. 21 2 5. Relative lipophilicity of the drug. Lipophilic drugs readily move across most biologic membranes. These drugs dissolve in the lipid membranes and penetrate the entire cell surface. The major factor influencing the distribution of lipophilic drugs is blood flow to the area. By contrast, hydrophilic drugs do not readily penetrate cell membranes and must pass through slit junctions. 22 2 Volume of distribution The apparent volume of distribution, Vd, is defined as the fluid volume that is required to contain the entire drug in the body at the same concentration measured in the plasma. It is calculated by dividing the dose that ultimately gets into the systemic circulation by the plasma concentration at time zero (C0). Vd = Amount of drug in the body C0 Chapter- 2 Pharmacokinetics & Pharmacodynamics 2 2 Drug clearance through metabolism Once a drug enters the body, the process of elimination begins. Elimination is irreversible removal of drug from the body. It involves: Biotransformation (drug metabolism) and Excretion (removal of drugs from the body). It includes the elimination of intact drug molecules that have not undergone metabolism, as well as the removal of metabolites that result from biotransformation (drug metabolism). The three major routes of elimination are hepatic metabolism, biliary elimination, and urinary excretion. 3 2 Reactions of drug metabolism The kidney cannot efficiently excrete lipophilic drugs that readily cross cell membranes and are reabsorbed in the distal convoluted tubules. Therefore, lipid-soluble agents are first metabolized into more polar (hydrophilic) substances in the liver via two general sets of reactions, called phase I and phase II. Products of metabolism may have lesser, greater, or qualitatively different pharmacologic activity from the parent compounds. 4 2 Phase I reactions Phase I reactions convert lipophilic drugs into more polar molecules. Phase I reactions involve: reduction, oxidation, or hydrolysis reactions. 5 2 Phase I reactions utilizing the P450 system Phase I reactions are mostly catalyzed by the cytochrome P450 (CYP450) system. Cytochrome P450 is a superfamily of heme-containing isozymes located in most cells, but primarily in the liver and GI tract. CYP450 enzymes exhibit considerable genetic variability among individuals and racial groups (genetic polymorphism). Polymorphism with CYP2D6- Codeine Polymorphism with CYP2C19- Clopidogrel 6 Cytochrome P450 (CYP) 2 CYP inducers Certain drugs (for example, phenobarbital, rifampin, phenytoin, carbamazepine, and oral contraceptives) are capable of inducing CYP isozymes. This results in increased biotransformation of drugs and can lead to significant decreases in plasma concentrations of drugs metabolized by these CYP isozymes, often with concurrent loss of pharmacologic effect. 7 Cytochrome P450 (CYP) 2 CYP inhibitors Inhibition of drug metabolism can lead to significant increases in plasma drug concentration and resultant adverse effects or drug toxicity. For example, omeprazole is a potent inhibitor of three CYP isozymes involved in warfarin metabolism. When taken with omeprazole, plasma concentrations of warfarin increase, which leads to greater anticoagulant effect and increased risk of bleeding. 8 2 Phase II reactions This phase consists of conjugation reactions. If the metabolite from phase I is sufficiently polar, it can be excreted by the kidneys. However, many phase I metabolites are still too lipophilic to be excreted. A subsequent conjugation reaction with an endogenous substrate, such as glucuronic acid, sulfuric acid, acetic acid, or an amino acid, results in: polar, usually more water-soluble compounds that are often therapeutically inactive and which are readily excreted in urine or bile. 9 2 Phase II reactions Glucuronidation is the most common and the most important conjugation reaction. [Note: Drugs already possessing an –OH, –NH2, or –COOH group may enter phase II directly and become conjugated without prior phase I metabolism (Figure 1.32).] 10 2 Phase II reactions The highly polar drug conjugates are then excreted by the kidney or in bile. Patients who are deficient in acetylation capacity are called slow acetylators. They may have prolonged or toxic responses to normal doses of certain drugs because of decreased metabolism. The opposite may be seen with fast acetylators. Phenytoin, caffeine, and sulphonamides are examples of drugs that undergo acetylation. In general, drugs are metabolized more slowly in elderly, neonates, and children than in adults. The fast acetylation phenotype is found primarily among Eskimos and Japanese, and slow acetylation among Scandinavians, Jews, and North Africans. 11 2 Drug clearance by the kidney Drugs must be sufficiently polar to be eliminated from the body. Removal of drugs from the body occurs via several routes: the most important is elimination through the kidney into the urine. If the drug is mainly renally excreted, patients with renal dysfunction may be unable to excrete drugs and are at risk for drug accumulation and adverse effects. 12 Renal elimination of 2 a drug A drug passes through several processes in the kidney before elimination: 1. Glomerular filtration, 2. Active tubular secretion, 3. Passive tubular reabsorption. 13 2 Excretion by other routes Drug excretion may also occur via the intestines, bile, lungs, and breast milk, among others. Drugs that are not absorbed after oral administration or drugs that are secreted into the bile are excreted in the feces. The lungs are primarily involved in the elimination of anesthetic gases (for example, desflurane). Elimination of drugs in breast milk may be a potential source of undesirable side effects to the infant. Excretion of most drugs into sweat, saliva, tears, hair, and skin occurs only to a small extent. 14 2 Drug clearance Total body clearance and drug half-life are important measures of drug clearance that are used to optimize drug therapy and minimize toxicity. Clearance is the volume of plasma from which the drug is completely removed per unit time. The amount eliminated is proportional to the concentration of the drug in the blood stream. 15 2 Total body clearance The total body clearance, CLtotal, is the sum of all clearances from the drug- metabolizing and drug-eliminating organs. Total clearance (CLtotal) is calculated using the following equation: where CLhepatic + CLrenal are typically the most important. Care should be exercised while administering drugs that may further reduce renal function e.g., NSAIDs 16 2 Drug half life Half-life (t1/2): time taken for the drug’s plasma concentration to decrease by 50%. The longer the half-life, the longer the drug remains in the body. When patients have abnormalities that alter the t1/2 of drugs, adjustment in dosages will be required. 17 2 Increased drug half-life require a decrease in dosage or less frequent dosing intervals. Examples of clinical situations that increase drug t1/2: Diminished renal or hepatic blood flow, e.g., in cardiogenic shock, heart failure, or hemorrhage; Decreased ability to extract drug from plasma, e.g., in renal disease; and Decreased metabolism, e.g., when a drug inhibits the metabolism of a concomitant drug or, in hepatic insufficiency, as with cirrhosis. 18 2 Optimization of dose The goal of drug therapy is to achieve and maintain concentrations within a therapeutic response window while minimizing toxicity and/ or adverse effects. With careful titration, most drugs can achieve this goal. If the therapeutic window of the drug is small (for example, digoxin or lithium), extra caution should be taken in selecting a dosage regimen, and drug levels should be monitored to ensure attainment of the therapeutic range. 19 2 Optimization of dose Drug regimens are administered as: 1. Maintenance doses or 2. Loading doses may be required if rapid effects are warranted. Maintenance dose Drugs are generally administered to maintain a steady-state plasma concentration (Css) within the therapeutic window. It takes four to five half-lives for a drug to achieve Css. Steady State: Refers to a stable drug concentration in the body where drug input equals drug output. 20 2 Prodrug A prodrug is a pharmacologically inactive drug originally but is biotransformed to an active therapeutic agent inside the body. For example, carbamazepine is metabolized to active metabolite oxcarbamazepine. The potential utility of this prodrug lies in its ability to retain its therapeutic action while eliminating or reducing the adverse effects of a drug. 4. Adrenergic agonists 4 & antagonists 4 I. Adrenergic agonists 3 Overview 4 The adrenergic drugs affect receptors that are stimulated by norepinephrine (noradrenaline) or epinephrine (adrenaline). These receptors are known as adrenergic receptors or adrenoceptors. Drugs that activate adrenergic receptors are termed sympathomimetics, and drugs that block activation of adrenergic receptors are termed sympatholytics. Some sympathomimetics directly activate adrenergic receptors (direct-acting agonists), while others act indirectly by enhancing release or blocking reuptake of norepinephrine (indirect-acting agonists). 4 5 THE ADRENERGIC NEURON 4 Adrenergic neurons release norepinephrine as the primary neurotransmitter. These neurons are found in: The central nervous system (CNS) and In the sympathetic nervous system, where they serve as links between ganglia and the effector organs. Adrenergic drugs act on adrenergic receptors, located either: presynaptically on the neuron or postsynaptically on the effector organ. 6 Neurotransmission at adrenergic neuron 4 Neurotransmission in adrenergic neurons closely resembles that described for the cholinergic neurons, except that norepinephrine is the neurotransmitter instead of acetylcholine. Neurotransmission involves the following steps: 1. synthesis, 2. storage, 3. release, and 4. receptor binding of norepinephrine, followed by 5. removal of the neurotransmitter from the synaptic gap 7 4 8 Adrenergic receptors (adrenoceptors) 4 In the sympathetic nervous system, several classes of adrenoceptors can be distinguished pharmacologically. Two main families of receptors, designated α and β, are classified based on response to the adrenergic agonists: epinephrine, norepinephrine, and isoproterenol. Both the α and β receptor types have a number of specific receptor subtypes. Alterations in the primary structure of the receptors influence their affinity for various agents. 9 α-adrenoceptors 4 The α-adrenoceptors show a weak response to the synthetic agonist isoproterenol, but they are responsive to the naturally occurring catecholamines epinephrine and norepinephrine (Figure 6.4). For α receptors, the rank order of potency and affinity is epinephrine ≥ norepinephrine >> isoproterenol. The α-adrenoceptors are divided into two subtypes, α1 and α2, based on their affinities for α agonists and antagonists. E.g., α1 receptors have a higher affinity for phenylephrine than α2 receptors. Conversely, the drug clonidine selectively binds to α2 receptors and has less effect on α1 receptors.