Chapter 3: Cell Structure and Function PDF
Document Details
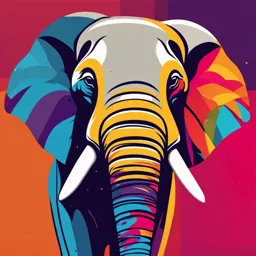
Uploaded by QuickerArchetype
Baumann
Tags
Summary
This document is a chapter on cell structure and function, part of a larger biology textbook. It explores the characteristics of life, prokaryotic and eukaryotic cells, and various cell processes. It includes questions to test understanding.
Full Transcript
3 Cell Structure and Function Before You Begin 1. What is the most abundant substance in organisms? 2. What is the characteristic of phospholipids that allows them to form double-layered membranes spontaneously? 3. Which macromolecules catalyze (speed up) chemical reactions inside cells? 4. Cells ha...
3 Cell Structure and Function Before You Begin 1. What is the most abundant substance in organisms? 2. What is the characteristic of phospholipids that allows them to form double-layered membranes spontaneously? 3. Which macromolecules catalyze (speed up) chemical reactions inside cells? 4. Cells have buffers. What is the function of buffers inside cells? MICRO IN THE CLINIC Kidney Infection Leading to Mental Confusion? PETE IS A 68-YEAR-OLD retired engineer. He remains mentally and physically active and is generally in good health. That’s part of the reason he was so surprised a couple of weeks ago when he found blood in his urine. Pete went to his doctor and was diagnosed with a kidney infection; the doctor prescribed a 14-day course of antibiotics. After the first week or so, Pete started to feel better, so he stopped taking the antibiotics. About two days ago, he noticed that the pain in his back had returned. He also felt a little dizzy and seemed to get winded more quickly than usual. Pete thinks he should go back to the doctor but wants to wait until after his granddaughter, Sara, visits. She doesn’t get the chance to visit very often, and they have a great day planned—a picnic in the park and a nice long walk along the lakeshore. Pete is thrilled when Sara arrives—but Sara looks worried when she sees her grandpa. He’s quite flushed, is a little bit sweaty, and seems to be a little bit confused or disoriented. Although Pete insists that he’s fine, Sara really wants to take him to the emergency room (ER). Pete continues to argue, but Sara guarantees that she won’t have a bit of fun if she’s worried about her grandpa all day long. Finally, Pete agrees to go to the ER. 1. Is Pete’s earlier kidney infection connected to his current symptoms? Turn to the end of the chapter (p. 89) to find out. Macrophage (blue) devouring Escherichia coli (red). Prokaryotic and Eukaryotic Cells: An Overview Processes of Life Many organisms also have the ability to move toward or away from environmental stimuli—a response called taxis. Metabolism. Metabolism can be defined as the ability of organisms to take in nutrients from outside themselves and use the nutrients in a series of controlled chemical reactions to provide the energy and structures needed to grow, reproduce, and be responsive. Metabolism is a unique process of living things; nonliving things cannot metabolize. Cells store metabolic energy in the chemical bonds of adenosine triphosphate ( -den -s n tr -fos f t), or ATP. (Major processes of microbial metabolism, including the generation of ATP, are discussed in Chapters 5–7.) L E A R N I N G O U TCO M E 3.1 Describe four major processes of living cells. Microbiology is the study of particularly small living things. That raises a question: What does living mean; how do we define life? Scientists once thought that living things were composed of special organic chemicals, such as glucose and amino acids, that carried a “life force” found only in living organisms. These organic chemicals were thought to be formed only by living things and to be very different from the inorganic chemicals of nonliving things. The idea that organic chemicals could come only from living organisms had to be abandoned in 1828, when Friedrich Wöhler (1800–1882) synthesized an organic molecule, urea, using only inorganic reactants in his laboratory. Today, we know that all living things contain both organic and inorganic chemicals and that many organic chemicals can be made from inorganic chemicals by laboratory processes. If organic chemicals can be made even in the absence of life, what is the difference between a living thing and a nonliving thing? What is life? At first, this may seem a simple question. After all, you can usually tell when something is alive. However, defining “life” itself can prove troublesome, so biologists generally avoid setting a definition, preferring instead to describe characteristics common to all living things. Biologists agree that all living things share at least four processes of life: growth, reproduction, responsiveness, and metabolism. Growth. Living things can grow; that is, they can increase in size. Reproduction. Organisms normally have the ability to reproduce themselves. Reproduction means that they increase in number, producing more organisms organized like themselves. Reproduction may be accomplished asexually (alone) or sexually with gametes (sex cells). Note that reproduction is an increase in number, whereas growth is an increase in size. Growth and reproduction often occur simultaneously. (We consider several methods of reproduction when we examine microbes in detail in Chapters 11–13.) Responsiveness. All living things respond to their environment. They have the ability to change themselves in reaction to changing conditions around or within them. TABLE 3.1 59 TABLE 3.1 shows how these characteristics, along with cell struc- ture, relate to various kinds of microbes. Organisms may not exhibit these four processes at all times. For instance, in some organisms reproduction may be postponed or curtailed by age or disease or, in humans at least, by choice. Likewise, the rate of metabolism may be reduced, as occurs in a seed, a hibernating animal, or a bacterial endospore,1 and growth often stops when an animal reaches a certain size. However, microorganisms typically grow, reproduce, respond, and metabolize as long as conditions are suitable. (Chapter 6 discusses the proper conditions for the metabolism and growth of various types of microorganisms.) TELL ME WHY The smallest free-living microbe—the bacterium Mycoplasma—is nonmotile. Why is it alive, even though it cannot move? Prokaryotic and Eukaryotic Cells: An Overview L E A R N I N G O U TCO M E 3.2 Compare and contrast prokaryotic and eukaryotic cells. In the 1800s, two German biologists, Theodor Schwann (1810–1882) and Matthias Schleiden (1804–1881), developed the theory that all living things are composed of cells. Cells are living entities, surrounded by a membrane, that are capable of growing, Characteristics of Life and Their Distribution in Microbes Characteristic Bacteria, Archaea, Eukaryotes Viruses Growth: increase in size Occurs in all Growth does not occur Reproduction: increase in number Occurs in all Host cell replicates the virus Responsiveness: ability to react to environmental stimuli Occurs in all Reaction to host cells seen in some viruses Metabolism: controlled chemical reactions of organisms Occurs in all Viruses use host cell’s metabolism Cellular structure: membrane-bound structure capable of all of the above functions Present in all Viruses lack cytoplasmic membrane or cellular structure 1 Endospores are resting stages, produced by some bacteria, that are tolerant of environmental extremes. 60 CHAPTER 3 Cell Structure and Function TEM Inclusions (a) SEM μm (b) LM 0 μm Ribosome ▲ FIGURE 3.1 Examples of types of cells. (a) Escherichia coli bacterial cells. (b) Paramecium, a single-celled eukaryote. Note the differences in magnification. Cytoplasm reproducing, responding, and metabolizing. The smallest living things are single-celled microorganisms. There are many different kinds of cells (FIGURE 3.1). Some cells are free-living, independent organisms; others live together in colonies or form the bodies of multicellular organisms. Cells also exist in various sizes, from the smallest bacteria to bird eggs, which are the largest of cells. All cells may be described as either prokaryotes (pr -kar - ts) or eukaryotes (y -kar - ts). Scientists categorize organisms based on shared characteristics into groups called taxa. Two taxa—domain Archaea and domain Bacteria—consist of organisms that are prokaryotic, but “prokaryote” is not itself a taxon. The distinctive feature of prokaryotes is that they can read their DNA genetic code and simultaneously make proteins—a typical prokaryote does not have a membrane surrounding its genetic material. In other words, a typical prokaryote does not have a nucleus (FIGURE 3.2). (Researchers have discovered a few prokaryotes with internal membranes that look like nuclei, but further investigation is needed to determine what these structures are.) The word prokaryote comes from Greek words meaning “before nucleus.” Moreover, electron microscopy has revealed that prokaryotes typically lack various types of internal structures bound with membranes that are present in eukaryotic cells. Bacteria and archaea differ fundamentally in such ways as the type of lipids in their cytoplasmic membranes and in the chemistry of their cell walls. In many ways, archaea are more like eukaryotes than they are like bacteria. (Chapter 11 discusses archaea and bacteria in more detail.) Eukaryotes have a membrane called a nuclear envelope surrounding their DNA, forming a nucleus (FIGURE 3.3), which sets eukaryotes in domain Eukarya. Indeed, the term eukaryote comes from Greek words meaning “true nucleus.” Besides the nuclear membrane, eukaryotes have numerous other internal membranes that compartmentalize cellular functions. These compartments are membrane-bound organelles—specialized structures that act like tiny organs to carry on the various functions of the cell. Organelles and their functions are discussed later in this chapter. The cells of algae, protozoa, fungi, animals, and plants are eukaryotic. Eukaryotes are usually larger, with cells 1 0 91 0 0 mm in diameter,2 and more complex than prokaryotes, which are often about 1.0 mm in diameter or smaller (FIGURE 3.4). 2 Micrometer (mm), which is one-millionth of a meter. Flagellum Nucleoid 0.5 μm Glycocalyx Cell wall Cytoplasmic membrane ▲ FIGURE 3.2 Typical prokaryotic cell. Prokaryotes include archaea and bacteria. The artist has extended an electron micrograph to show three dimensions. Not all prokaryotic cells contain all these features. Although there are many kinds of cells, they all share the characteristic processes of life as previously described, as well as certain physical features. In this chapter, we will distinguish among bacterial, archaeal, and eukaryotic “versions” of physical features common to cells, including (1) external structures, (2) the cell wall, (3) the cytoplasmic membrane, and (4) the cytoplasm. We will also discuss features unique to each type. (Chapters 11, 12, and 19–23 examine further details of prokaryotic and eukaryotic organisms, their classification, and their ability to cause disease.) MICRO CHECK 1. All living cells take in nutrients, which provide building materials and energy for a complex set of chemical reactions inside the cell. What are these reactions called? 2. Through what process do living cells increase in number? 3. When comparing size, how would you describe prokaryotes versus eukaryotes? 4. What feature is missing in prokaryotes but commonly found in eukaryotes, as hinted at by their names? TELL ME WHY In 1985, an Israeli scientist discovered the single-celled microbe Epulopiscium fishelsoni. This organism is visible with the naked eye. Why did the scientist initially think Epulopiscium was eukaryotic? What discovery revealed that the microbe is really a giant bacterium? Next, we explore characteristics of bacterial cells, beginning with external features and working into the cell. Prokaryotic and Eukaryotic Cells: An Overview 61 b FIGURE 3.3 Typical eukaryotic cell. Not Nuclear envelope all eukaryotic cells have all these features. The artist has extended the electron micrograph to show three dimensions. Note the difference in magnification between this cell and the prokaryotic cell in the previous figure. Besides size, what major difference between prokaryotes and eukaryotes was visible to early microscopists? Figure 3.3 Eukaryotic cells contain nuclei, which are visible with light microscopes, whereas prokaryotes lack nuclei. TEM Nuclear pore Nucleolus Lysosome Mitochondrion Centriole Secretory vesicle Golgi body Cilium Transport vesicles Ribosomes Rough endoplasmic reticulum Smooth endoplasmic reticulum 10 μm Cytoplasmic membrane Cytoskeleton Virus Orthopoxvirus 0.3 μm diameter Bacterium Staphylococcus 1 μm diameter Chicken egg 4.7 cm diameter (47,000 μm)* Parasitic protozoan Giardia 14 μm length *Actually, the inset box on the egg would be too small to be visible. (Width of box would be about 0.002 mm.) b FIGURE 3.4 Approximate size of various types of cells. Birds’ eggs are the largest cells. Note that Staphylococcus, a bacterium, is smaller than Giardia, a unicellular eukaryote. A smallpox virus (Orthopoxvirus) is shown only for comparison; viruses are not cellular. 62 CHAPTER 3 Cell Structure and Function External Structures of Bacterial Cells Many cells have special external features that enable them to respond to other cells and their environment. In bacteria, these features include glycocalyces, flagella, fimbriae, and pili. Glycocalyces 3.4 Flagella L E A R N I N G O U TCO M E S L E A R N I N G O U TCO M E S 3.3 n -m n - ) and Klebsiella pneumoniae (kleb-s -el n -m n - ) enable these prokaryotes to avoid destruction by defensive cells in the respiratory tract and to cause pneumonia. Unencapsulated strains of these same bacterial species do not cause disease because the body’s defensive cells destroy them. Describe the composition, function, and relevance to human health of glycocalyces. Distinguish capsules from slime layers. Some cells have a gelatinous, sticky substance that surrounds the outside of the cell. This substance is known as a glycocalyx (plural: glycocalyces), which literally means “sweet cup.” The glycocalyx may be composed of polysaccharides, polypeptides, or both. These chemicals are produced inside the cell and are extruded onto the cell’s surface. When the glycocalyx of a bacterium is composed of organized repeating units of organic chemicals firmly attached to the cell’s surface, the glycocalyx is called a capsule (FIGURE 3.5a). In contrast, a loose, water-soluble glycocalyx is called a slime layer (FIGURE 3.5b). Glycocalyces protect cells from drying (desiccation) and can also play a role in the ability of pathogens to survive and cause disease. For example, slime layers are often sticky and provide one means for bacteria to attach to surfaces as biofilms, which are aggregates of many bacteria living together on a surface. Oral bacteria colonize the teeth as a biofilm called dental plaque. Bacteria in a dental biofilm can produce acid and cause dental caries (cavities). The chemicals in many bacterial capsules can be similar to chemicals normally found in the body, preventing bacteria from being recognized or devoured by defensive cells of the host. For example, the capsules of Streptococcus pneumoniae (strep-t -kok s 3.5 3.6 Discuss the structure and function of bacterial flagella. List and describe four bacterial flagellar arrangements. A cell’s motility may enable it to flee from a harmful environment or move toward a favorable environment, such as one where food or light is available. The most notable structures responsible for such bacterial movement are flagella. Bacterial flagella (singular: flagellum) are long structures that extend beyond the surface of a cell and its glycocalyx and propel the cell through its environment. Not all bacteria have flagella, but for those that do, their flagella are very similar in Play Motility: Overview composition, structure, @ Mastering Microbiology and development. Structure Bacterial flagella are composed of three parts: a filament, a hook, and a basal body (FIGURE 3.6). A filament is a long hollow shaft, about 20 nm in diameter,3 that extends out of the cell into its environment. No membrane covers a filament. A bacterial flagellum is composed of many identical globular molecules of a protein called flagellin. A flagellum lengthens by growing at its tip as the cell secretes molecules of flagellin through the hollow core of the flagellum, to be deposited in a clockwise helix at the tip of the filament. Bacterial flagella react to external wetness, inhibiting their own growth in dry habitats. Glycocalyx (capsule) Glycocalyx (slime layer) b FIGURE 3.5 Glycocalyces. (a) Micrograph of a single cell of the bacterium Streptococcus, showing a prominent capsule. (b) An unidentified skin bacterium has a slime layer surrounding the cell. What advantage does a glycocalyx provide a cell? Figure 3.5 A glycocalyx provides protection from drying and from being devoured; it may also help attach cells to one another and to surfaces in the environment. (a) TEM 250 nm TEM (b) 3 250 nm Nanometer (nm), which is a billionth of a meter. External Structures of Bacterial Cells 63 b FIGURE 3.6 Proximal structure of bacterial flagella. (a) Detail of k Figure 3.6 Flagella of Gram-positive cells have a single pair of rings in the basal body that function to attach the flagellum to the cytoplasmic membrane. The flagella of Gram-negative cells have two pairs of rings: one pair anchors the flagellum to the cytoplasmic membrane, the other pair to the cell wall. Filament flagellar structure of a Gram-positive cell. (b) Detail of the flagellum of a Gram-negative bacterium. How do flagella of Gram-positive bacteria differ from those of Gram-negative bacteria? H o o Direction of rotation during run Rod Peptidoglycan layer (cell wall) Protein rings Cytoplasmic membrane (a) Cytoplasm Filament H o o k Outer protein rings Outer membrane Rod Gram + Gram – Basal body Peptidoglycan layer Integral protein Inner protein rings Cytoplasm Cell wall Cytoplasmic membrane Integral protein (b) At its base, a filament inserts into a curved structure, the hook, which is composed of a different protein. The basal body, which is composed of still different proteins, anchors the filament and hook to the cell wall and cytoplasmic membrane by means of a rod and a series of either two or four rings of proteins. Together, the hook, rod, and rings allow the filament to rotate 360°. Differences in the proteins associated with bacterial flagella vary enough to allow classification of Play Flagella: Structure species into strains called @ Mastering Microbiology serovars. Arrangement Bacteria may have one of several flagellar arrangements (FIGURE 3.7). For example, flagella that cover the surface of the cell are termed peritrichous;4 in contrast, polar flagella are only at the ends. Other bacteria have tufts of polar flagella. Some spiral-shaped bacteria, called spirochetes (sp r -k ts),5 have flagella at both ends that spiral tightly around the cell instead of protruding into the surrounding medium. These 4 From Greek peri, meaning “around,” and trichos, meaning “hair.” From Greek speira, meaning “coil,” and chaeta, meaning “hair.” 5 64 CHAPTER 3 (a) Peritrichous flagella Cell Structure and Function SEM 0.5 μm SEM (a) Endoflagella rotate Axial filament 5 μm Axial filament rotates around cell Outer membrane Periplasmic space (b) Single polar flagellum SEM 0.5 μm Cytoplasmic membrane Spirochete corkscrews and moves forward Axial filament (b) ▲ FIGURE 3.8 Axial filament. (a) Scanning electron micrograph of two spirochete cells of Borrelia burgdorferi, which causes Lyme disease. (b) Diagram of axial filament wrapped around a spirochete. Cross section reveals that an axial filament is composed of endoflagella. (c) Tuft of polar flagella SEM 0. 5 μm ▲ FIGURE 3.7 Micrographs of basic arrangements of bacterial flagella. flagella, called endoflagella, form an axial filament that wraps around the cell between its cytoplasmic membrane and an outer membrane (FIGURE 3.8). Rotation of endoflagella evidently causes the axial filament to rotate around the cell, causing the spirochete to “corkscrew” through its medium. Treponema pallidum (trep- n m pal li-d m), the agent of syphilis, and Borrelia burgdorferi (b -r l - burg-d r fer- ), the cause of Lyme disease, are notable spirochetes. Some scientists think that the corkscrew motility of these pathogens allows Play Flagella: Arrangement; Spirochetes them to invade @ Mastering Microbiology human tissues. Function Although the precise mechanism by which bacterial flagella operate is not completely understood, we do know that they rotate 360° like boat or airplane propellers rather than whipping from side to side. The flow of ions (electrically charged atoms) through the cytoplasmic membrane near the basal body powers the rotation, propelling the bacterium through the environment at about 60 cell lengths per second—equivalent to a car traveling at 670 miles per hour! Flagella can rotate at more than 100,000 rpm and can change direction from counterclockwise to clockwise. Bacteria move with a series of “runs” interrupted by “tumbles.” Counterclockwise flagellar rotation produces movements of a cell in a single direction for some time; this is called a run. If 65 External Structures of Bacterial Cells Attractant Run Fimbriae and Pili L E A R N I N G O U TCO M E 3.7 Tumble Run Tumble ▲ FIGURE 3.9 Motion of a peritrichous bacterium. In peritrichous bacteria, runs occur when all of the flagella rotate counterclockwise and become bundled. Tumbles occur when the flagella rotate clockwise, become unbundled, and the cell spins randomly. In positive chemotaxis (shown), runs last longer than tumbles, resulting in motion toward the chemical attractant. What triggers a bacterial flagellum to rotate counterclockwise, producing a run? Figure 3.9 Favorable environmental conditions induce runs. more than one flagellum is present, the flagella align and rotate together as a bundle. Runs are interrupted by brief, abrupt, random changes in direction called tumbles. Tumbles result from clockwise flagellar rotation where each flagellum rotates independently. Receptors for light or chemicals on the surface of the cell send signals to the flagella, which then adjust their speed and direction of rotation. If, during its random movements, a cell finds itself approaching a favorable stimulus, it increases the duration of the runs, which tends to move the cell toward an attractant—the bacterium moves itself into a more favorable environment (FIGURE 3.9). Unfavorable stimuli increase the duration of runs that are in a direction away from a repellent. This tends to move the cell toward a more favorable environment. Movement in response to a stimulus is termed taxis. Stimuli are often either light (phototaxis) or a chemical (chemotaxis). Movement toward a favorable stimulus is positive taxis, whereas movement away from an unfavorable stimulus is negative taxis. For example, movement toward Play Flagella: Movement a nutrient would be positive @ Mastering Microbiology chemotaxis. Compare and contrast the structures and functions of fimbriae, pili, and flagella. Many bacteria have rodlike proteinaceous extensions called fimbriae (fim br - ; singular: fimbria). These sticky, bristlelike projections adhere to one another and to substances in the environment. There may be hundreds of fimbriae per cell, and they are usually shorter than flagella (FIGURE 3.10). An example of a bacterium with fimbriae is Neisseria gonorrhoeae (n -se r go-nor-r ), which causes gonorrhea. Pathogens must be able to adhere to their hosts if they are to survive and cause disease. This bacterium is able to colonize the mucous membrane of the reproductive tract by attaching with fimbriae. Neisseria cells that lack fimbriae are nonpathogenic. Some fimbriae carry enzymes that render soluble, toxic metal ions into insoluble, nontoxic forms. Bacteria may use fimbriae to move across a surface via a process similar to pulling an object with a rope. The bacterium extends a fimbria, which attaches at its tip to the surface; then the bacterium retracts the fimbria, pulling itself toward the attachment point. Flagellum Fimbria SEM μm ▲ FIGURE 3.10 Fimbriae. Proteus vulgaris has flagella and fimbriae. Fimbriae also serve an important function in biofilms, three-dimensional slimy masses of microbes adhering to a substrate and to one another by means of fimbriae and glycoBacteria calyces (FIGURE 3.11). It has been estimated that at least 99% Matrix (slime) MICRO CHECK 5. What are the three parts of bacterial flagella? 6. What term describes the flagellar arrangement where flagella cover the entire surface of a bacterial cell? SEM μm ▲ FIGURE 3.11 Biofilms. Biofilms are organized layered systems of microbes attached to surfaces—here, the inside surface of medical tubing. 66 CHAPTER 3 Cell Structure and Function Pilus SEM 0.5 μm ▲ FIGURE 3.12 Pili. Two Escherichia coli cells are connected by a pilus. How are pili different from bacterial flagella? Figure 3.12 Bacterial flagella are flexible structures that rotate to propel the cell; pili are hollow tubes used to transfer DNA from one cell to another. of bacteria in nature exist in biofilms. Some fimbriae act as electrical wires, conducting electrical signals among cells in a biofilm. Researchers are interested in biofilms because of the roles they play in human diseases and in industry. For example, biofilms form plaque on teeth, form slime inside medical catheters, are involved in about two-thirds of bacterial human infections, clog drains, and corrode industrial pipes. Further, the architecture of a biofilm provides protection that free-floating bacteria lack. For example, the lower concentrations of oxygen found in the interior of biofilms thwart the effectiveness of some antibiotics. A special type of fimbria is a pilus (p lus; plural: pili, p l ), also called a conjugation pilus. Pili are usually longer than other fimbriae but shorter than flagella. Typically, only one to a few pili are present per cell in bacteria that have them. Cells use pili to transfer DNA from one cell to the other via a process termed conjugation (FIGURE 3.12). (Chapter 7 deals with conjugation in more detail.) osmotic forces (described shortly). In addition, a cell wall assists some cells in attaching to other cells or in resisting antimicrobial drugs. Note that animal cells do not have cell walls, a difference that plays a key role in treatment of many bacterial diseases with certain types of antibiotics. For example, penicillin attacks the cell wall of bacteria but is harmless to human cells, because the latter lack walls. Cell walls give bacterial cells characteristic shapes. Spherical cells, called cocci (kok s ), may appear in various arrangements, including singly or in chains (streptococci), clusters (staphylococci), or cuboidal packets (sarcinae, sar si-n ) (FIGURE 3.13), depending on the planes of cell division. Rodshaped cells, called bacilli (b -sil ), typically appear singly or in chains. Bacterial cell walls are composed of peptidoglycan (pep ti-d -gl kan), a meshlike complex polysaccharide. Peptidoglycan in turn is composed of two types of regularly alternating sugar molecules, called N-acetylglucosamine (NAG) and N-acetylmuramic acid (NAM), which are structurally similar to glucose (FIGURE 3.14). Millions of NAG and NAM molecules are covalently linked in chains in which NAG and NAM alternate. These chains are the “glycan” portions of peptidoglycan. Chains of NAG and NAM are attached to other NAG-NAM chains by crossbridges of four amino acids (tetrapeptides) (a) (b) ▲ FIGURE 3.13 Bacterial shapes and arrangements. (a) Spherical cocci may be single or in arrangements such as chains (streptococci), clusters (staphylococci), or cuboidal packets (sarcinae). (b) Rod-shaped bacilli may also be single or in arrangements such as chains. TELL ME WHY Glucose Why is a pilus a type of fimbria, but a flagellum is not a type of fimbria? CH2OH H Bacterial Cell Walls HO O H OH H H OH N-acetylglucosamine NAG CH2OH H H O OH L E A R N I N G O U TCO M E S 3.8 3.9 3.10 Describe common shapes and arrangements of bacterial cells. Describe the sugar and peptide portions of peptidoglycan. Compare and contrast the cell walls of Gram-positive and Gram-negative bacteria in terms of structure and Gram staining. The cells of most prokaryotes are surrounded by a cell wall that provides structure and shape to the cell and protects it from OH H H NH CH3 (b) CH2OH O C (a) N-acetylmuramic acid NAM H O H H O O O HC C H CH3 O O H NH C O CH3 OH ▲ FIGURE 3.14 Comparison of the structures of glucose, NAG, and NAM. (a) Glucose. (b) N-acetylglucosamine (NAG) and N-acetylmuramic acid (NAM) molecules linked as in peptidoglycan. Blue shading indicates the differences between glucose and the other two sugars. Orange boxes highlight the difference between NAG and NAM. Bacterial Cell Walls Sugar chain NA NA NA NA Tetrapeptide (amino acid) crossbridge G NA M NA G NA M NA G NA M NA NA M G M NA NA NA M NA NA G G NA NA NA NA M M NA G NA NA M M M NA NA M M M M NA G M M M Connecting chain of amino acids ▲ FIGURE 3.15 Possible structure of peptidoglycan. Peptidoglycan is composed of chains of NAG and NAM linked by tetrapeptide crossbridges and, in some cases, as shown here, connecting chains of amino acids to form a tough yet flexible structure. The amino acids of the crossbridges differ among bacterial species. 67 Gram-positive bacterium retains the crystal violet dye used in the Gram staining procedure, so the stained cells appear purple under magnification. Some additional chemicals are associated with the walls of some Gram-positive bacteria. For example, species of Mycobacterium (m k -bak-t r - m), which include the causative agents of tuberculosis and leprosy, have walls with up to 60% mycolic acid, a waxy lipid. Mycolic acid helps these cells survive desiccation (drying out) and makes them difficult to stain with regular water-based dyes. Researchers have developed a special staining procedure called the acid-fast stain to stain these Gram-positive cells that contain large amounts of waxy lipids. Such cells are called acid-fast bacteria (see Chapter 4). Gram-Negative Bacterial Cell Walls L E A R N I N G O U TCO M E 3.12 Describe the clinical implications of the structure of the Gram-negative cell wall. Gram-positive bacterial cell walls have a relatively thick layer of peptidoglycan that also contains unique chemicals called teichoic6 acids (t -k ik). Some teichoic acids are covalently linked to lipids, forming lipoteichoic acids that anchor the peptidoglycan to the cytoplasmic membrane of the cell (FIGURE 3.16a). Teichoic acids have negative electrical charges, which help give the surface of a Gram-positive bacterium a negative charge and may play a role in the passage of ions through the wall. The thick cell wall of a Gram-negative cell walls have only a thin layer of peptidoglycan outside the cytoplasmic membrane (FIGURE 3.16b), but outside the peptidoglycan there is another, outer membrane. This outer membrane is a bilayer; that is, it is composed of two different layers, or leaflets. The inner leaflet of the outer membrane is composed of phospholipids and proteins, but the outer leaflet is made of lipopolysaccharide (LPS). Integral proteins called porins form channels through both leaflets of the outer membrane, allowing midsized molecules such as glucose to move freely across the outer membrane. LPS is a union of lipid with sugar. The lipid portion of LPS, known as lipid A, can be toxic. The erroneous idea that lipid A is inside Gram-negative cells led to the use of the term endotoxin7 for LPS. Dead Gram-negative cells release lipid A when the outer membrane disintegrates. This is medically important because lipid A may trigger fever, vasodilation, inflammation, shock, and blood clotting in humans. Killing large numbers of Gram-negative bacteria with antimicrobial drugs within a short time period releases large amounts of lipid A, which might threaten the patient more than the live bacteria; thus, any internal infection by Gram-negative bacteria is cause for concern. The outer membrane of a Gram-negative cell can also be an impediment to the treatment of disease. For example, the outer membrane may prevent the movement of penicillin to the underlying peptidoglycan, thus rendering this drug ineffectual against many Gram-negative pathogens. Between the cytoplasmic membrane and the outer membrane of Gram-negative bacteria is a periplasmic space (see Figure 3.16b). The periplasmic space contains the peptidoglycan and periplasm, the name given to the gel between the membranes. Periplasm contains water, nutrients, and substances secreted by 6 7 between neighboring NAMs. FIGURE 3.15 illustrates one possible configuration. Such peptide crossbridges are the “peptido” portion of peptidoglycan. Depending on the bacterium, tetrapeptide bridges are either bonded to one another or held together by short connecting chains of other amino acids, as shown in Figure 3.15. Peptidoglycan covers the entire surface of a cell, which must insert millions of new NAG and NAM subunits if it is to grow and divide. Scientists describe two basic types of bacterial cell walls as Gram-positive cell walls or Gram-negative cell walls. They distinguish Gram-positive and Gram-negative cells by the use of the Gram staining procedure (described in Chapter 4), which was invented long before the structure and chemical nature of bacterial cell walls were known. Gram-Positive Bacterial Cell Walls L E A R N I N G O U TCO M E 3.11 Compare and contrast the cell walls of acid-fast bacteria with typical Gram-positive cell walls. From Greek teichos, meaning “wall.” From Greek endo, meaning “inside,” and toxikon, meaning “poison.” 68 CHAPTER 3 Cell Structure and Function Peptidoglycan layer (cell wall) Cytoplasmic membrane (a) Gram-positive cell wall Lipoteichoic acid Teichoic acid Integral protein Porin Outer membrane of cell wall Porin (sectioned) Peptidoglycan layer of cell wall (b) Gram-negative cell wall Periplasmic space Cytoplasmic membrane Phospholipid layers Lipopolysaccharide (LPS) layer, containing lipid A Integral proteins ▲ FIGURE 3.16 Comparison of cell walls of Gram-positive and Gram-negative bacteria. (a) The Gram-positive cell wall has a thick layer of peptidoglycan and lipoteichoic acids that anchor the wall to the cytoplasmic membrane. (b) The Gram-negative cell wall has a thin layer of peptidoglycan and an outer membrane composed of lipopolysaccharide (LPS), phospholipids, and proteins. What effects can lipid A have on human physiology? Figure 3.16 Lipid A can cause shock, blood clotting, and fever in humans. the cell, such as digestive enzymes and proteins involved in specific transport. The enzymes function to catabolize large nutrient molecules into smaller molecules that can be absorbed or transported into the cell. Because the cell walls of Gram-positive and Gram-negative bacteria differ, the Gram stain is an important diagnostic tool. After the Gram staining procedure, Gram-negative cells appear pink, and Gram-positive cells appear purple. Bacterial Cytoplasmic Membranes Bacteria Without Cell Walls Structure A few bacteria, such as Mycoplasma pneumoniae (m k -plaz-m n -m n - ), lack cell walls entirely. In the past, these bacteria were often mistaken for viruses because of their small size and lack of walls. However, they do have other features of prokaryotic cells, such as prokaryotic ribosomes (discussed later in the chapter). L E A R N I N G O U TCO M E S MICRO CHECK 7. Two of the most common shapes for bacteria are spherical and rod shaped. What is the proper term for spherical cells? 8. Bacteria may live as single cells or may cluster together in specific arrangements. What prefix is used for organisms that grow in chains? 9. One distinction between Gram-positive and Gram-negative bacteria is the relative thickness of peptidoglycan. Which group has a thicker layer of peptidoglycan? 10. What chemical gives the surfaces of Gram-positive and acid-fast bacteria their negative charges? TELL ME WHY Why is the microbe illustrated in Figure 3.2 more likely a Gram-positive bacterium than a Gram-negative one? Bacterial Cytoplasmic Membranes Beneath the glycocalyx and the cell wall is a cytoplasmic membrane. The cytoplasmic membrane may also be referred to as the cell membrane or a plasma membrane. Head, which contains phosphate (hydrophilic) 3.13 3.14 Diagram a phospholipid bilayer, and explain its significance in reference to a cytoplasmic membrane. Explain the fluid mosaic model of membrane structure. Cytoplasmic membranes are about 8 nm thick and are composed of phospholipids (see Chapter 2; Figure 2.16) and associated proteins. Some bacterial membranes also contain sterol-like molecules, called hopanoids, that help stabilize the membrane. The structure of a cytoplasmic membrane is referred to as a phospholipid bilayer (FIGURE 3.17). A phospholipid molecule is bipolar; that is, the two ends of the molecule are different. The phosphate-containing heads of each phospholipid molecule are hydrophilic,8 meaning that they are attracted to water at the two surfaces of the membrane. The hydrocarbon tails of each phospholipid molecule are hydrophobic9 and huddle together with other tails in the interior of the membrane, away from water. Phospholipids placed in a watery environment naturally form a bilayer because of their bipolar nature. About half of a bacterial cytoplasmic membrane is composed of integral proteins inserted amidst the phospholipids. Some integral proteins penetrate the entire bilayer; others are found in only half the bilayer. In contrast, peripheral proteins are loosely attached to the membrane on one side or the other. Proteins of cell membranes may act as recognition proteins, enzymes, receptors, carriers, or channels. The fluid mosaic model describes our current understanding of membrane structure. The term mosaic indicates that the membrane proteins are arranged in a way that resembles the tiles in a mosaic, and fluid indicates that the proteins and lipids are free to move laterally within a membrane. Play Membrane Structure @ Mastering Microbiology Phospholipid Tail (hydrophobic) Integral proteins Cytoplasm Integral protein Phospholipid bilayer Peripheral protein Integral protein ▲ FIGURE 3.17 Structure of a prokaryotic cytoplasmic membrane: a phospholipid bilayer. 69 8 From Greek hydor, meaning “water,” and philos, meaning “love.” 9 From Greek hydor, meaning “water,” and phobos, meaning “fear.” 70 CHAPTER 3 Cell Structure and Function MICRO CHECK 11. Phospholipid molecules have heads that are described as “loving water.” What is the proper term for this behavior? 12. Which end of a phospholipid orients itself toward the interior of a phospholipid bilayer? Function L E A R N I N G O U TCO M E S 3.15 3.16 3.17 Describe the functions of a cytoplasmic membrane as they relate to permeability. Compare and contrast the passive and active processes by which materials cross a cytoplasmic membrane. Define osmosis, and distinguish among isotonic, hypertonic, and hypotonic solutions. A cytoplasmic membrane does more than separate the contents of a cell from its outside environment. The cytoplasmic membrane also controls the passage of substances into and out of the cell. Nutrients are brought into the cell, and wastes are removed. The membrane also functions for harvesting light energy in photosynthetic bacteria producing molecules of ATP. (Chapter 5 discusses photosynthesis and ATP synthesis.) In its function of controlling the contents of a cell, the cytoplasmic membrane is selectively permeable, meaning that it allows some substances to cross while preventing the crossing of others. How does a membrane exert control over subPlay Membrane Permeability stances that move across it @ Mastering Microbiology and the contents of the cell? A phospholipid bilayer is naturally impermeable to most substances. Large molecules cannot cross between the packed phospholipids; ions and molecules with an electrical charge are repelled by it; and hydrophilic substances cannot easily cross its hydrophobic interior. However, cytoplasmic membranes contain proteins, and some of these proteins function as pores, channels, or carriers that allow substances to cross the membrane. Movement across a cytoplasmic membrane occurs by either passive or active processes. Passive processes do not require the expenditure of a cell’s metabolic energy store (usually ATP), whereas active processes do require the expenditure of cellular energy, either directly or indirectly. Active and passive processes will be discussed shortly, but first you must understand another feature of selectively permeable cytoplasmic membranes: their ability to maintain a concentration gradient. Membranes enable a cell to concentrate chemicals on one side of the membrane or the other. The difference in concentration of a chemical on the two sides of a membrane is the chemical’s concentration gradient (also known as a chemical gradient). Because many of the substances that have concentration gradients across cell membranes are electrically charged chemicals, a corresponding electrical gradient, or voltage, also exists across the membrane (FIGURE 3.18). For example, a greater concentration of negatively charged proteins exists inside the membrane, and positively charged sodium ions are more concentrated outside the membrane. One result of the segregation of electrical charges by a membrane is that the interior of a cell is usually electrically negative compared to the exterior. This tends to repel negatively charged chemicals and attract positively charged subPlay Passive Transport: Principles of Diffusion stances into @ Mastering Microbiology cells. CLINICAL CASE STUDY The Big Game College sophomore Nadia is a star point guard for her school’s basketball team. She is excited about the divisional finals Friday night—she’s even heard rumors that a professional scout will be in the stands. On Thursday morning, she wakes up with a sore throat. Her forehead doesn’t feel warm, so she forces herself to attend her Thursday classes; but when she wakes up on Friday morning, her throat is noticeably worse. Still, she forces herself to attend Friday morning class but feels tired and much worse by noon. It is downright painful to swallow, and she skips lunch. Nearly crying, she heads back to the dorm and checks her temperature—101°F. Desperate, she walks to the student health center, where a nurse practitioner notices white patches on the back of Nadia’s throat and on her tonsils. The divisional basketball game starts in six hours, but it only takes a few minutes for the nurse practitioner to perform a rapid streptococcal antigen test and determine that Nadia has streptococcal, also known as group A Streptococcus (GAS), pharyngitis—strep throat. She will miss the big game. Strep throat is caused by an encapsulated, Gram-positive bacterium, Streptococcus pyogenes. The only good news is that by taking the prescribed penicillin, Nadia should be ready for her next big game—hopefully, the quarterfinals. 1. How does the capsule of Streptococcus contribute to the bacterium’s ability to cause disease? 2. What bacterial structures, besides the capsule, may be allowing Streptococcus to infect Nadia’s throat? 3. Penicillin works by interrupting the formation of peptidoglycan. What bacterial structure contains peptidoglycan? 4. In a Gram-positive organism such as Streptococcus, is peptidoglycan typically thicker or thinner than it would be in a Gram-negative bacterium? Bacterial Cytoplasmic Membranes Cell exterior (extracellular fluid) + + + – + + + + + + + + + + + Passive processes – Cl– In passive processes, electrochemical gradients provide energy to move substances across the membrane; the cell does not expend its ATP. Passive processes include diffusion, facilitated diffusion, and osmosis. 0 mV + + – + Na+ –30 –70 + + + + + Cytoplasmic membrane – Integral protein + – – – – – + – + _ _ – – – – – – _ – _ – – + Protein – DNA – Protein – – Cell interior (cytoplasm) ▲ FIGURE 3.18 Electrical potential of a cytoplasmic membrane. The electrical potential, in this case -70 mV, exists across a membrane because there are more negative charges inside the cell than outside it. Diffusion Diffusion is the net movement of a chemical down its concentration gradient—that is, from an area of higher concentration to an area of lower concentration. It requires no energy output by the cell, a common feature of all passive processes. In fact, diffusion occurs even in the absence of cells or their membranes. In the case of diffusion into or out of cells, only chemicals that are small or lipid soluble can diffuse through the lipid portion of the membrane (FIGURE 3.19a). For example, oxygen, carbon dioxide, alcohol, and fatty acids can freely diffuse through the cytoplasmic membrane, but molecules such as glucose and proteins cannot. Facilitated Diffusion The phospholipid bilayer blocks the movement of large or electrically charged molecules, so they do not cross the membrane unless there is a pathway for diffusion. As we have seen, cytoplasmic membranes contain integral proteins. Some of these proteins act as channels or carriers to allow certain molecules to diffuse down their concentration gradients into or out of the cell. This process is called facilitated diffusion because the proteins facilitate the process by providing a pathway for diffusion. The cell expends no energy in facilitated diffusion; electrochemical gradients provide all of the energy necessary. Some channel proteins allow the passage of a range of chemicals that have the right size or electrical charge (FIGURE 3.19b). Other channel proteins, known as permeases, are more specific, carrying only certain substrates (FIGURE 3.19c). A permease has a binding site that is selective for one substance. b FIGURE 3.19 Passive processes of movement across Extracellular fluid a cytoplasmic membrane. Passive processes always involve movement down an electrochemical gradient. Cytoplasm (a) Diffusion of small or lipid-soluble chemicals through the membrane 71 (b) Facilitated diffusion of several types of chemicals through a nonspecific channel (c) Facilitated diffusion through a permease of a specific chemical (d) Osmosis (net movement of water through a specific channel or through the membrane) CHAPTER 3 Cell Structure and Function Osmosis When discussing simple and facilitated diffusion, we considered a solution in terms of the solutes (dissolved chemicals) it contains because it is those solutes that move into and out of the cell. In contrast, with osmosis it is useful to consider the concentration of the solvent, which in organisms is always water. Osmosis is the special name given to the diffusion of water across a semipermeable membrane—that is, across a membrane that is permeable to water molecules but not to most solutes that are present, such as proteins, amino acids, salts, or glucose (FIGURE 3.19d). Because these solutes cannot freely penetrate the membrane, they cannot diffuse across the membrane, no matter how unequal their concentrations on either side may be. Instead, the water diffuses. Water molecules cross from the side of the membrane that contains a higher concentration of water (lower concentration of solute) to the side that contains a lower concentration of water (higher concentration of solute). In osmosis, water moves across the membrane until equilibrium is reached, or until the pressure of water is equal to the force of osmosis (FIGURE 3.20). We commonly compare solutions according to their concentrations of solutes. When solutions on either side of a selectively permeable membrane have the same concentration of solutes, the two solutions are said to be isotonic.10 In an isotonic situation, neither side of a selectively permeable membrane will experience a net loss or gain of water (FIGURE 3.21a). When the concentrations of solutions are unequal, the solution with the higher concentration of solutes is said to be hypertonic11 to the other. The solution with a lower concentration of solutes is hypotonic12 in comparison. Note that the terms hypertonic and hypotonic refer to the concentration of solute, even though osmosis refers to the movement of water. The terms isotonic, hypertonic, and hypotonic are relative. For example, a glass of tap water is isotonic to another glass of the same water, but it is hypertonic compared to distilled water, and hypotonic when compared to seawater. In biology, the three terms are traditionally used relative (a) Solutes (chemicals dissolved in water) (b) Semipermeable membrane allows movement of water, but not of solutes ▲ FIGURE 3.20 Osmosis, the diffusion of water across a semiper- meable membrane. (a) A membrane separates two solutions of different concentrations in a U-shaped tube. The membrane is permeable to water, but not to the solute. (b) After time has passed, water has moved down its concentration gradient until water pressure prevented the osmosis of any additional water. Which side of the tube more closely represents a living cell? Figure 3.20 The right-hand side represents the cell, because cells are typically hypertonic to their environment. 72 to the interior of cells. Most cells are hypertonic to their environments. A hypertonic solution, with its higher concentration of solutes, necessarily means a lower concentration of water; that is, a hypertonic solution has a lower concentration of water than does a hypotonic solution. Like other chemicals, water moves down its concentration gradient from a hypotonic solution into a hypertonic solution. A cell placed in a hypertonic solution will therefore lose water and shrivel (FIGURE 3.21b). b FIGURE 3.21 Effects of isotonic, Cells without a wall (e.g., mycoplasmas, animal cells) H2O H2O H2O Solutes Cell wall Cells with a wall (e.g., plants, fungal and bacterial cells) Cell wall Cytoplasmic membrane (a) Isotonic solution 10 From Greek isos, meaning “equal,” and tonos, meaning “tone.” From Greek hyper, meaning “more” or “over.” 12 From Greek hypo, meaning “less” or “under.” 11 H2O H2O H 2O Solutes Cell membrane (b) Hypertonic solution (c) Hypotonic solution hypertonic, and hypotonic solutions on cells. (a) Cells in isotonic solutions experience no net movement of water. (b) Cells in hypertonic solutions shrink because of the net movement of water out of the cell. (c) Cells in hypotonic solutions undergo a net gain of water. Animal cells burst because they lack a cell wall; in cells with a cell wall, the pressure of water pushing against the interior of the wall eventually stops the movement of water into the cell. Bacterial Cytoplasmic Membranes Active Processes As stated previously, active processes expend energy (often stored in ATP) to move material against its electrochemical gradient across a membrane. As we will see, ATP may be utilized directly during transport or indirectly at some other site or at some other time. Active processes in bacteria include active transport by means of carrier proteins and a spePlay Active Transport: Overview cial process termed group @ Mastering Microbiology translocation. Active Transport Like facilitated diffusion, active transport utilizes transmembrane permease proteins; however, the functioning of active transport proteins requires the cell to expend ATP to move molecules across the membrane. Some such proteins are referred to as gated channels or ports because they are controlled. When the cell is in need of a substance, the protein becomes functional (the gate “opens”). At other times, the gate is “closed.” If only one substance is transported at a time, the permease is called a uniport (FIGURE 3.22a). In contrast, antiports simultaneously transport two chemicals, but in opposite directions; that is, one substance is transported into the cell at the same time that a second substance is transferred out of the cell (FIGURE 3.22b). In other types of active transport, two substances move together in the same direction across the membrane by means of a single carrier protein. Such proteins are known as symports (FIGURE 3.22c). In all cases, active transport moves substances against their electrochemical gradients. Typically, the transport protein acts as an ATPase—an enzyme that breaks down ATP into ADP during transport, releasing energy that is used to move the substance across the membrane against its electrochemical gradient. With symports and antiports, one chemical’s electrochemical gradient may provide the energy needed to transport a second chemical, a mechanism called coupled transport (Figure 3.22c). For example, a uniport can pump H+ out of a cell using ATP to establish an electrochemical gradient of hydrogen ions. Then H+ moving back into the cell down its electrochemical gradient provides energy to bring a second Uniport ATP ATP ADP ADP ATP ADP Symport (a) Uniport (c) Coupled transport (in this case, a uniport and a symport) (b) Antiport ▲ FIGURE 3.22 Mechanisms of active transport. Cellular energy is used to transport the chemical against its electrochemical gradient. (a) Via a uniport. (b) Via an antiport. (c) Via a uniport coupled with a symport. In this example, the membrane uses ATP energy to pump one substance out through a uniport. As this substance flows back into the cell, it brings another substance with it through the symport. What is the usual source of energy for active transport? Figure 3.22 ATP is the usual source of energy for active transport processes. In contrast, water will diffuse into a cell placed in a hypotonic solution because the cell has a higher solutes-to-water concentration. As water moves into the cell, water pressure against its cytoplasmic membrane increases, and the cell expands (FIGURE 3.21c). One function of a cell wall, such as the peptidoglycan of bacteria, is to resist further osmosis and prevent cells from bursting. It is useful to compare solutions to the concentration of solutes in a patient’s blood cells. Isotonic saline solutions administered to a patient have the same percent dissolved solute (in this case, salt) as do the patient’s cells. Thus, the patient’s intracellular and extracellular environments remain in equilibrium when an isotonic saline solution is administered. However, if the patient is infused with a hypertonic solution, water will move out of the patient’s cells, and the cells will shrivel, a condition called crenation. Conversely, if a patient is infused with a hypotonic solution, water will move into the patient’s cells, Play Passive Transport: Special Types of which will swell Diffusion @ Mastering Microbiology and possibly burst. 73 chemical with it through a symport. At first glance it may appear that the second chemical is moving passively against its electrochemical gradient—no ATP is directly involved in movement through the symport. However, active transport utilizing ATP is involved in pumping hydrogen ions out of the cell, and this is coupled with the movement Play Active Transport: Types of the second chemical @ Mastering Microbiology into the cell. Group Translocation Many bacteria use an active process called group translocation. In group translocation, the substance transported across the membrane is chemically changed during transport (FIGURE 3.23). The membrane is impermeable to the altered C Extracellular fluid Cytoplasm P C P ▲ FIGURE 3.23 Group translocation. This process involves a chemical change to the substance being transported, in this case, the addition of phosphate. 74 CHAPTER 3 TABLE 3.2 Cell Structure and Function Transport Processes Across Bacterial Cytoplasmic Membranes Description Examples of Transported Substances Passive Transport Processes Processes require no use of energy by the cell; the electrochemical gradient provides energy. Diffusion Molecules move down their electrochemical gradient through the phospholipid bilayer of the membrane. Oxygen, carbon dioxide, lipid-soluble chemicals Facilitated diffusion Molecules move down their electrochemical gradient through channels or carrier proteins. Glucose, fructose, urea, some vitamins Osmosis Water molecules move down their concentration gradient across a selectively permeable membrane. Water Active Transport Processes Cell expends energy in the form of ATP to move a substance against its electrochemical gradient. Active transport ATP-dependent carrier proteins bring substances into cell. Na+, K +, Ca2+, H +, Cl - Group translocation The substance is chemically altered during transport. Glucose, mannose, fructose substance, trapping it inside the cell. Group translocation is very efficient at bringing substances into a cell. It can operate effectively even if the external concentration of the chemical being transported is as low as 1 part per million (ppm). One well-studied example of group translocation is the accumulation of glucose inside a bacterial cell. As glucose is transported across the membrane, the glucose is phosphorylated; that is, a phosphate group is added to change it into glucose 6-phosphate, a sugar that can be used in the ATP-producing metabolism of the cell. Other carbohydrates, fatty acids, purines, and pyrimidines are also brought into bacterial cells by group translocation. A summary of bacterial transport processes is shown in TABLE 3.2. MICRO CHECK 13. The cell membrane determines what is permitted to enter or exit a cell. What is the term used to describe this property? 14. What form of diffusion is used by large molecules that must use a passageway provided by integral proteins? 15. What term describes an aqueous solution in which animal cells swell and burst? TELL ME WHY When the bacterium Escherichia coli is grown in a hypertonic solution, it turns on a gene to synthesize a protein that transports potassium into the cell. Why? Cytoplasm of Bacteria L E A R N I N G O U TCO M E S 3.18 Describe bacterial cytoplasm and its basic contents. 3.19 Define inclusion, and give two examples. 3.20 Describe the formation and function of endospores. Cytoplasm is the general term used to describe the gelatinous material inside a cell. Cytoplasm is semitransparent, fluid, elastic, and aqueous. It is composed of cytosol, inclusions, ribosomes, and, in many cells, a cytoskeleton. Some bacterial cells produce internal, resistant, dormant forms called endospores. Cytosol The liquid portion of the cytoplasm is called cytosol. It is mostly water, but it also contains dissolved and suspended substances, including ions, carbohydrates, proteins (mostly enzymes), lipids, and wastes. The cytosol of prokaryotes also contains the cell’s DNA in a region called the nucleoid. Recall that a distinctive feature of prokaryotes is lack of a membrane surrounding this DNA. Most bacteria have a single, circular DNA molecule organized as a chromosome. Some bacteria, such as Vibrio cholerae (vib r kol er- ), the bacterium that causes cholera, have two chromosomes. The cytosol is the site of some chemical reactions. For example, enzymes within the cytosol function to produce amino acids and degrade sugar. Inclusions Deposits, called inclusions, are often found within bacterial cytosol. Rarely, a cell surrounds its inclusions with a polypeptide membrane. Inclusions may include reserve deposits of lipids, starch, or compounds containing nitrogen, phosphate, or sulfur. Such chemicals may be taken in and stored in the cytosol when nutrients are in abundance and then utilized when nutrients are scarce. The presence of specific inclusions is diagnostic for several pathogenic bacteria. Some bacteria store carbon and energy in molecules of a lipid polymer called polyhydroxybutyrate (PHB) (FIGURE 3.24). Long chains of PHB accumulate as inclusion granules in the cytoplasm. Slight chemical modification of PHB produces a plastic that can be used for packaging and other applications (see Beneficial Microbes: Plastics Made Perfect?). PHB plastics are biodegradable, breaking down in a landfill in a few weeks rather than persisting for years as petroleum-based plastics do. Many aquatic cyanobacteria (blue-green photosynthetic bacteria) contain inclusions called gas vesicles that store gases in protein sacs. The gases buoy the cells to the surface and into the light needed for photosynthesis. Cytoplasm of Bacteria ▲ FIGURE 3.24 Granules of PHB in a bacterium. TEM μm Endospores Some bacteria, notably Bacillus (ba-sil s) and Clostridium (klos-trid - m), are characterized by the ability to produce unique dormant entities called endospores. Endospores are important for several reasons, including their durability and potential pathogenicity. Though some people refer to endospores simply as “spores,” endospores should not be confused with the reproductive spores of actinobacteria, algae, and fungi. A bacterial cell, called a vegetative cell to distinguish it from an endospore, transforms into only one endospore, which later germinates to grow into only one vegetative cell; therefore, endospores are not reproductive structures. Instead, endospores constitute a defensive strategy against hostile or unfavorable conditions. A vegetative cell normally transforms itself into an endospore only when one or more nutrients (such as carbon or nitrogen) are in limited supply. The process of endospore formation, called sporulation, requires 8–10 hours (FIGURE 3.25). During the process, two membranes, a thick layer of peptidoglycan, and a spore coat form around a copy of the cell’s DNA and a small portion of cytoplasm. The cell deposits large quantities of dipicolinic acid, calcium, and DNA-binding proteins within the endospore while removing most of the water. The remains of the vegetative cell die, and the endospore is released. Depending on the species, a cell forms an endospore either centrally, subterminally (near one end), or terminally (at one end). Sometimes an endospore is so large it swells its vegetative cell. Endospores are extremely resistant to drying, heat, radiation, and lethal chemicals. For example, they remain alive in boiling water for several hours; are unharmed by alcohol, peroxide, bleach, and other toxic chemicals; and can tolerate over 400 rad of radiation, which is more than five times the dose that is lethal to most humans. Endospores are stable resting stages that barely metabolize—they are essentially in a state of suspended animation—and they germinate only when conditions improve. Scientists do not know how endospores are able to resist harsh conditions, but it appears that the double membrane, spore coats, dipicolinic acid, calcium, and DNA-binding proteins serve to stabilize DNA and enzymes, protecting them from adverse conditions. The ability to survive harsh conditions makes endospores the most resistant and enduring cells. In one case, scientists were BENEFICIAL MICROBES Plastics Made Perfect? Petroleum-based plastics play a considerable role in modern life, appearing in packaging, bottles, appliances, furniture, automobiles, disposable diapers, and many other synthetic goods. Despite the good that plastic brings to our lives, this artificial polymer also brings problems. Manufacturers make plastic from oil, PHB bottle caps. One exacerbating U.S. dependence on foreign partially biodegraded supplies of crude oil. Consumers discard in 60 days. plastic, filling landfills with more than 15 million tons of plastic every year in the United States. Because plastic is artificial, microorganisms do not break it down effectively, and discarded plastic might remain in landfills for decades or centuries. What is needed is a functional “green” plastic—a plastic that is strong and light and that can be shaped and colored as needed yet is more easily biodegradable. Enter the bacteria. Many bacterial cells, particularly Gramnegative bacteria, use polyhydroxybutyrate (PHB) as a storage 75 molecule and energy source, much as humans use fat. PHB and similar storage molecules turn out to be rather versatile plastics that are produced when bacteria metabolizing certain types of sugar are simultaneously deprived of an essential element, such as nitrogen, phosphorus, or potassium. The bacteria, faced with such a nutritionally stressed environment, convert the sugar to PHB, which they store as intracellular inclusions. Scientists harvest these biologically created molecules by breaking the cells open and treating the cytoplasm with chemicals to isolate the plastics and remove dangerous endotoxin. Purified PHB possesses many of the properties of petrochemically derived plastic—its melting point, crystal structure, molecular weight, and strength are very similar. Further, PHB has a singular, overwhelming advantage compared to artificial plastic: PHB is naturally and completely biodegradable—bacteria catabolize PHB into carbon dioxide and water. The positive effect that increasing PHB use would have on our overtaxed landfills would be tremendous, and replacing just half of the oil-based plastic used in the United States with PHB could reduce oil usage by more than 250 million barrels per year. 76 CHAPTER 3 Cell Structure and Function (a) Steps in Endospore Formation Cell wall Cytoplasmic membrane 1 DNA is replicated. DNA Vegetative cell 2 Cytoplasmic membrane invaginates to form forespore. Forespore 3 Cytoplasmic membrane grows and engulfs forespore within a second membrane. Vegetative cell’s DNA disintegrates. First membrane able to revive endospores of Clostridium that had been sealed in a test tube for 34 years. This record pales, however, beside other researchers’ claim to have revived Bacillus endospores from inside 250-million-year-old salt crystals retrieved from an underground site near Carlsbad, New Mexico. Some scientists question this claim, suggesting that the bacteria might be recent contaminants. In any case, there is little doubt that endospores can remain viable for a minimum of tens, if not thousands, of years. Endospore formation is a serious concern to food processors, health care professionals, and governments because endospores are resistant to treatments that inhibit other microbes, and because endospore-forming bacteria produce deadly toxins that cause such fatal diseases as anthrax, tetanus, and gangrene. (Chapter 9 considers techniques for controlling endospore formers.) Nonmembranous Organelles L E A R N I N G O U TCO M E Second membrane 4 A cortex of peptidoglycan is deposited between the membranes; meanwhile, dipicolinic acid and calcium ions accumulate within the center of the endospore. Cortex 3.21 Describe the structure and function of ribosomes and the cytoskeleton. As previously noted, prokaryotes do not usually have membranes surrounding their organelles. However, two types of nonmembranous organelles are found in direct contact with the cytosol in bacterial cytoplasm: ribosomes and the cytoskeleton. Some investigators do not consider them to be true organelles because they lack a membrane, but other scientists consider them nonmembranous organelles. Spore coat 5 Spore coat forms around endospore. Ribosomes Outer spore coat 6 Endospore matures: Completion of spore coat. Increase in resistance to heat and chemicals by unknown processes. Endospore 7 Endospore is released from original cell. (b) Endospore inside Bacillus subtilis Endospores TEM 0.5 μm ▲ FIGURE 3.25 Endospores. (a) Formation of an endospore occurs over Ribosomes synthesize proteins. Bacterial cells have thousands of ribosomes in their cytoplasm, which gives cytoplasm a grainy appearance (see Figure 3.2). The approximate size of ribosomes—and indeed other cellular structures—is expressed in Svedbergs (S)13 and is determined by their sedimentation rate: the rate at which they move to the bottom of a test tube during centrifugation. As you might expect, large, compact, heavy particles sediment faster than small, loosely packed, or light ones and are assigned a higher number. Prokaryotic ribosomes are 70S; in contrast, the larger ribosomes of eukaryotes are 80S. All ribosomes are composed of two subunits, each of which is composed of polypeptides and molecules of RNA called ribosomal RNA (rRNA). The subunits of prokaryotic 70S ribosomes are a smaller 30S subunit and a larger 50S subunit; the 30S subunit contains polypeptides and a single rRNA molecule, whereas the 50S subunit has polypeptides and two rRNA molecules. Because sedimentation rates depend not only on mass and size but also on shape, the sedimentation rates of subunits do not add up to the sedimentation rate of a whole ribosome. Many antibacterial drugs act on bacterial 70S ribosomes or their subunits without deleterious effects on the larger 80S ribosomes of eukaryotic cells (see Chapter 10). This is why such 13 Svedberg units are named for Theodor Svedberg, a Nobel Prize winner and the inventor of the ultracentrifuge. a period of 8-10 hours. (b) An endospore inside a vegetative cell of Bacillus. M03_BAUM2302_06_SE_C03.indd 76 08/06/19 7:59 AM External Structures of Archaea 77 External Structures of Archaea Archaeal cells have external structures similar to those seen in bacteria. These include glycocalyces, flagella, and fimbriae. Some archaea have another kind of proteinaceous appendage called a hamus. We consider each of these in order beginning with the outermost structures—glycocalyces. Glycocalyces L E A R N I N G O U TCO M E LM μm ▲ FIGURE 3.26 A simple helical cytoskeleton. The rod-shaped bacterium Bacillus subtilis has a helical cytoskeleton composed of only a single protein, which has been stained with a fluorescent dye. drugs can stop protein synthesis in bacteria without affecting protein synthesis in a patient. Cytoskeleton Cells have an internal scaffolding called a cytoskeleton, which is composed of three or four types of protein fibers. Bacterial cytoskeletons play a variety of roles in the cell. For example, one type of cytoskeleton fiber wraps around the equator of a cell and constricts, dividing the cell into two. Another type of fiber forms a helix down the length of some cells (FIGURE 3.26). Such helical fibers appear to play a role in the orientation and deposition of strands of NAG and NAM sugars in the peptidoglycan wall, thereby determining the shape of the cell. Other fibers help keep DNA molecules segregated to certain areas within bacterial cells. An unusual motile bacterium, Spiroplasma (sp r -plaz-m ), which lacks flagella, uses contractile elements of its cytoskeleton to swim through its environment. MICRO CHECK 16. What is the term for the region inside a bacterial cell where the DNA can be found? 17. Some bacteria store energy reserves and other useful molecules. What are these stored deposits called? 18. Why do some bacteria form endospores? 19. What organelle is found in both prokaryotes and eukaryotes and produces new proteins? TELL ME WHY The 2001 bioterrorist anthrax attacks in the United States involved Bacillus anthracis. Why is B. anthracis able to survive in mail? We have considered bacterial cells. Next we turn our attention to archaea—the other prokaryotic cells—and compare them to bacterial cells. 3.22 Compare the structure and chemistry of archaeal and bac- terial glycocalyces. Like those of bacteria, archaeal glycocalyces are gelatinous, sticky, extracellular structures composed of polysaccharides, polypeptides, or both. Scientists have not studied archaeal glycocalyces as much as those of bacteria, but archaeal glycocalyces function at a minimum in the formation of biofilms— adhering cells to one another, to other types of cells, and to nonliving surfaces in the environment. Organized glycocalyces (capsules) of bacteria and bacterial biofilms are often associated with disease. Some research has demonstrated the presence of archaea in some biofilms associated with oral gum disease; however, no archaeon has been shown conclusively to be pathogenic. Flagella L E A R N I N G O U TCO M E S 3.23 Describe the structure and formation of archaeal flagella. 3.24 Compare and contrast archaeal flagella with bacterial flagella. Archaea use flagella to move through their environments, though at a slower speed than bacteria. An archaeal flagellum is superficially similar to a bacterial flagellum: it consists of a basal body, hook, and filament, each composed of protein. The basal body anchors the flagellum in the cell wall and cytoplasmic membrane. As with bacterial flagella, archaeal flagella extend outside the cell, are not covered by a membrane, and rotate like propellers; however, scientists have discovered many differences between archaeal and bacterial flagella: Archaeal flagella are 10–14 nm in diameter, which is about half the thickness of bacterial flagella. Archaeal flagella are not hollow. Archaeal flagella, lacking a central channel, grow with the addition of subunits at the base of the filament rather than at the tip. The proteins making up archaeal flagella share common amino acid sequences across archaeal species. These sequences are very different from the amino acid sequences common to bacterial flagella. Sugar molecules are attached to the filaments of many archaeal flagella, a condition that is rare in bacteria. 78 CHAPTER 3 Cell Structure and Function Archaeal flagella are powered with energy stored in molecules of ATP, whereas the flow of hydrogen ions across the membrane powers bacterial flagella. Archaeal flagella rotate together as a bundle both when they rotate clockwise and when they rotate counterclockwise. In contrast, bacterial flagella operate independently when rotating clockwise. These differences indicate that archaeal flagella arose independently of bacterial flagella; they are analogous structures— having similar structure without having a common ancestor. Each hamus is a helical filament with tiny prickles sticking out at regular intervals, much like barbed wire. The end of the hamus is frayed into three distinct arms, each of which has a thickened end and bends back toward the cell to make the entire structure look like a grappling hook. Indeed, hami function to securely attach archaea to surfaces. TELL ME WHY Why do scientists consider bacterial and archaeal flagella to be analogous rather than evolutionary relations? Fimbriae and Hami L E A R N I N G O U TCO M E S 3.25 Compare the structure and function of archaeal and bacte- rial fimbriae. 3.26 Describe the structure and function of hami. L E A R N I N G O U TCO M E S Many archaea have fimbriae—nonmotile, rodlike, sticky projections. As with bacteria, archaeal fimbriae are composed of protein and anchor the cells to one another and to environmental surfaces. Some archaea make unique proteinaceous, fimbriae-like structures called hami14 (singular: hamus). More than 100 hami may radiate from the surface of a single archaeon (FIGURE 3.27). Hamus Grappling hook Prickles SEM SEM 50 nm 500 nm ▲ FIGURE 3.27 Archaeal hami. Archaea use hami, which are shaped like grappling hooks on barbed wire, to attach themselves to structures in the environment. 14 From Latin hamus, meaning “prickle,” “claw,” “hook,” or “barb.” Archaeal Cell Walls and Cytoplasmic Membranes 3.27 Contrast types of archaeal cell walls with each other and with bacterial cell walls. 3.28 Contrast the archaeal cytoplasmic membrane with that of bacteria. Most archaea, like most bacteria, have cell walls. All archaea have cytoplasmic membranes. However, there are distinct differences between archaeal and bacterial walls and membranes, further emphasizing the uniqueness of archaea. Archaeal cell walls are composed of specialized proteins or polysaccharides. In some species, the outermost protein molecules form an array that coats the cell like chain mail. Archaeal walls lack peptidoglycan, which is common to all bacterial cell walls. Gram-negative archaeal cells, which appear pink when Gram stained, have an outer layer of protein rather than an outer lipid bilayer as seen in Gram-negative bacteria. Gram-positive archaea have a thick cell wall and Gram stain purple, like Gram-positive bacteria. Archaeal cells are typically spherical or rod shaped, though irregularly shaped, needle-like, rectangular, and square archaea exist (FIGURE 3.28). The cytoplasmic membranes of archaea often contain lipids that have branched hydrocarbons linked to glycerol by ether linkages rather than by ester linkages as in bacterial membranes (see Table 2.3 on p. 41). Ether linkages are stronger in many ways than ester linkages. They are mechanically stronger, more stable at high temperatures, and more salt tolerant, allowing archaea to live in extreme environments such as near-boiling water and hypersaline lakes. An archaeal cytoplasmic membrane maintains electrical and chemical gradients in the cell. It also functions to control the import and export of substances from the cell using membrane proteins as ports and pumps, just as proteins are used in bacterial cytoplasmic membranes. TELL ME WHY Why did scientists in the 19th and early 20th centuries think that archaea were bacteria? 79 Cytoplasm of Archaea SEM (a) 0.5 μm SEM (b) μm (c) LM 5 μm ▲ FIGURE 3.28 Representative shapes of archaea. (a) Cocci, Pyrococcus furiosus, attached to rod-shaped Methanopyrus kandleri. (b) Irregularly shaped archaeon, Thermoplasma acidophilum. (c) Square archaeon, Haloquadratum walsbyi. What are the stringlike extensions of Pyrococcus? Figure 3.28 The extensions are fimbriae or hami. Cytoplasm of Archaea L E A R N I N G O U TCO M E 3.29 Compare and contrast the cytoplasm of archaea with that To this point, we have discussed basic features of bacterial and archaeal prokaryotic cells. (Chapter 11 discusses the classification of prokaryotic organisms in more detail.) Next we turn our attention to eukaryotic cells. of bacteria. Cytoplasm is the gel-like substance found in all cells, including archaea. Like bacteria, archaeal cells have 70S ribosomes, a fibrous cytoskeleton, and circular DNA suspended in a liquid cytosol. Also like bacteria, they lack membranous organelles. However, archaeal cytoplasm differs from that of bacteria in several ways. For example, the protein molecules in archaeal ribosomes differ from those in the ribosomes of bacteria; indeed, archaeal ribosomal proteins are more similar to eukaryotic ribosomal proteins. Scientists further distinguish archaea from bacteria in that archaea use different metabolic enzymes to make RNA and use a genetic code more similar to the code used by eukaryotes. (Chapter 7 discusses these genetic differences in more detail.) TABLE 3.3 contrasts features of archaea and bacteria. TABLE 3.3 MICRO CHECK 20. What are archaeal glycocalyces composed of? 21. What are the grappling-hook-like structures used by archaea to attach to surfaces in their environment? 22. What substance do bacterial cell walls contain that archaeal cell walls lack? 23. What are the benefits of the ether linkages in archaeal cytoplasmic membranes, compared to the ester linkages in bacterial cytoplasmic membranes? 24. How do archaeal ribosomes differ from bacterial ribosomes? Some Structural Characteristics of Prokaryotes Feature Archaea Bacteria Glycocalyx Polypeptide or polysaccharide Polypeptide or polysaccharide Flagella Present in some; 10–14 nm in diameter; grow at base; rotate both counterclockwise and clockwise as bundles Present in some; about 20 nm in diameter; grow at the tip; rotate counterclockwise in bundles to cause runs; rotate independently clockwise to cause tumbles Fimbriae Proteinaceous; used for attachment and in formation of biofilms Proteinaceous; used for attachment, gliding motility, and in formation of biofilms Pili None discovered Present in some; proteinaceous; used in bacterial exchange of DNA Hami Present in some; used for attachment Absent Cell Walls Present in most; composed of polysaccharides (not peptidoglycan) or proteins Present in most; composed of peptidoglycan, a polysaccharide Cytoplasmic Membrane Present in all; membrane lipids are made with ether linkages; some have single lipid layer Present in all; phospholipids are made with ester linkages in bilayer Cytoplasm Cytosol contains circular DNA molecule and 70S ribosomes; ribosomal proteins are similar to eukaryotic ribosomal proteins Cytosol contains a circular DNA molecule and 70S ribosomes with bacterial proteins 80 CHAPTER 3 Cell Structure and Function TELL ME WHY Why do some scientists consider archaea, which are prokaryotic, more closely related to eukaryotes than they are to the other prokaryotes—bacteria? External Structure of Eukaryotic Cells Some eukaryotic cells have glycocalyces, which are similar to those of prokaryotes. Glycocalyces L E A R N I N G O U TCO M E The walls of plant cells are composed of cellulose, a polysaccharide that is familiar to you as paper and dietary fiber. Fungi also have walls of polysaccharides, including cellulose, chitin, or glucomannan. The walls of algae (FIGURE 3.29) are composed of a variety of polysaccharides or other chemicals, depending on the type of alga. These chemicals include cellulose, proteins, agar, carrageenan, silicates, algin, calcium carbonate, or a combination of these substances. (Chapter 12 discusses fungi and algae in more detail.) All eukaryotic cells have cytoplasmic membranes (FIGURE 3.30). A eukaryotic cytoplasmic membrane, like those of bacteria, is a fluid mosaic of phospholipids and proteins, which act as recognition molecules, enzymes, receptors, carriers, or channels. Channel proteins for facilitated diffusion are Cell wall Cytoplasmic membranes 3.30 Describe the composition, function, and importance of eukaryotic glycocalyces. Animal and most protozoan cells lack cell walls, but such a cell may have a sticky glycocalyx15 that is anchored to the cell’s cytoplasmic membrane via covalent bonds to membrane proteins and lipids. Eukaryotic glycocalyces are not as structurally organized as prokaryotic capsules. Animal cell glycocalyces help to anchor cells to each other, strengthen the cell surface, provide some protection against dehydration, and function in cell-to-cell recognition and communication. Glycocalyces are absent in eukaryotes that have cell walls, such as plants and fungi. TELL ME WHY Why are eukaryotic glycocalyces covalently bound to cytoplasmic membranes, and why don’t eukaryotes with cell walls have glycocalyces? TEM L E A R N I N G O U TCO M E S ▲ FIGURE 3.29 A eukaryotic cell wall. The cell wall of the red alga Gelidium is composed of layers of the polysaccharide called agar. What is the function of a cell wall? Figure 3.29 The cell wall provides support, protection, and resistance to osmotic forces. Eukaryotic Cell Walls and Cytoplasmic Membranes μm 3.31 Compare and contrast prokaryotic and eukaryotic cell walls and cytoplasmic membranes. Cytoplasm of one cell 3.32 Contrast exocytosis and endocytosis. 3.33 Describe the role of pseudopods in eukaryotic cells. The eukaryotic cells of fungi, algae, plants, and some protozoa have cell walls. Recall that glycocalyces are absent from eukaryotes with cell walls; instead, the cell wall takes on some of the functions of a glycocalyx by providing protection from the environment and anchoring neighboring cells to one another. The wall also provides shape and support against osmotic pressure. Most eukaryotic cell walls are composed of various polysaccharides, but not the peptidoglycan seen in the walls of bacteria. Cytoplasmic membrane Intercellular matrix Cytoplasm of second cell Cytoplasmic membrane TEM 15 From Greek glykys, meaning “sweet,” and kalyx, meaning “cup” or “husk.” 100 nm ▲ FIGURE 3.30 Eukaryotic cytoplasmic membrane. Note that this micrograph depicts the cytoplasmic membranes of two adjoining cells. 81 Eukaryotic Cell Walls and Cytoplasmic Membranes Pseudopod LM 50 μm ▲ FIGURE 3.31 Endocytosis. Pseudopods extend to surround solid and/or liquid nutrients, which become incorporated into a food vesicle inside the cytoplasm. What is the difference between phagocytosis and pinocytosis? Figure 3.31 Phagocytosis is endocytosis of a solid; pinocytosis is endocytosis of a liquid. more common in eukaryotes than in prokaryotes. Additionally, within multicellular organisms some membrane proteins serve to anchor cells to each other. Eukaryotic cytoplasmic membranes may differ from prokaryotic membranes in several ways. Eukaryotic membranes contain steroid lipids (sterols), such as cholesterol in animal cells, that help maintain membrane fluidity. Paradoxically, at high temperatures sterols stabilize a phospholipid bilayer by making it less fluid, but at low temperatures sterols have the opposite effect—they prevent phospholipid packing, making the membrane more fluid. Eukaryotic cytoplasmic membranes may contain small, distinctive assemblages of lipids and proteins that remain together in the membrane as a functional group and do not “float” independently amidst other membrane components. Such distinct regions are called membrane rafts. Eukaryotic cells use membrane rafts to localize cellular processes, including signaling the inside of the cell, protein sorting, and some kinds of cell movement. Some viruses, including those of AIDS, Ebola, measles, and flu, use membrane rafts to enter human cells or during viral replication. Researchers hope that blocking molecules in membrane rafts will provide a way to limit the spread of these viruses. Eukaryotic cells frequently attach chains of sugar molecules to the outer surfaces of lipids and proteins in their cytoplasmic membranes; prokaryotes rarely do this. Sugar molecules may act in intercellular signaling, in cellular attachment, and in other roles. TABLE 3.4 Like its prokaryotic counterpart, a eukaryotic cytoplasmic membrane controls the movement of materials into and out of a cell. Eukaryotic cytoplasmic membranes use both passive processes (diffusion, facilitated diffusion, and osmosis; see Figure 3.19) and active transport (see Figure 3.22). Eukaryotic membranes do not perform group translocation, which occurs only in prokaryotes, but many perform another type of active transport—endocytosis (FIGURE 3.31), which involves physical manipulation of the cytoplasmic membrane around the cytoskeleton. Endocytosis occurs when the membrane distends to form pseudopods16 (soo d -podz) that surround a substance, bringing it into the cell. Endocytosis is termed phagocytosis if a solid is brought into the cell and pinocytosis if only liquid is brought into the cell. Nutrients brought into a cell by endocytosis are then enclosed in a food vesicle. Vesicles and digestion of the nutrients they contain are discussed in more detail shortly. (Chapter 15 considers the process of phagocytosis as it relates to the defense of the body against disease.) Some eukaryotes also use pseudopods as a means of locomotion. The cell extends a pseudopod, and then the cytoplasm streams into it, a process called amoeboid action. Exocytosis, another solely eukaryotic process, is the reverse of endocytosis in that it enables substances to be exported from the cell. Not all eukaryotic cells can perform endocytosis or exocytosis. TABLE 3.4 lists some of the features of endocytosis and exocytosis. Active Transport Processes Found Only in Eukaryotes: Endocytosis and Exocytosis Description Examples of Transported Substances Endocytosis: Phagocytosis and Pinocytosis Substances are surrounded by pseudopods and brought into the cell. Phagocytosis involves solid substances; pinocytosis involves liquids. Bacteria, viruses, aged and dead cells; liquid nutrients in extracellular solutions Exocytosis Vesicles containing substances are fused with cytoplasmic membrane, dumping their contents to the outside. Wastes, secretions 16 From Greek pseudes, meaning “false,” and podos, meaning “foot.” 82 CHAPTER 3 Cell Structure and Function MICRO CHECK Function 25. Eukaryotic cells contain steroid-based lipids in their cytoplasmic membranes that help maintain membrane fluidity. What example of this is found in animal cells? 26. Some eukaryotic cells bring solid materials inside the cell by extending portions of the cell membrane. What is the term for those elongated extensions? The flagella of eukaryotes also move differently from those of prokaryotes. Rather than rotating, the flagella of eukaryotes undulate rhythmically (FIGURE 3.33a). Some eukaryotic flagella push the cell through the medium (as occurs in animal sperm), whereas others pull the cell through the medium (as occurs in many protozoa). Positive and negative phototaxis and chemotaxis are seen in eukaryotic cells, but such cells do not move in runs and tumbles. TELL ME WHY Cilia Many antimicrobial drugs target bacterial cell walls. Why aren’t there many drugs that act against bacterial cytoplasmic membranes? Cytoplasm of Eukaryotes L E A R N I N G O U TCO M E S 3.34 Compare and contrast the cytoplasm of prokaryotes and eukaryotes. 3.35 Identify nonmembranous and membranous organelles. The gel-like cytoplasm of eukaryotic cells is more complex than that of either bacteria or archaea. The most distinctive difference is the presence of numerous membranous organelles in eukaryotes. However, before we discuss these membranous organelles, we will consider organelles of locomotion and other nonmembranous organelles in eukaryotes. Flagella L E A R N I N G O U TCO M E 3.36 Compare and contrast the structure and function of pro- karyotic and eukaryotic flagella. L E A R N I N G O U TCO M E S 3.37 Describe the structure and function of cilia. 3.38 Compare and contrast eukaryotic cilia and flagella. Other eukaryotic cells move by means of motile, hairlike structures called cilia, which extend the surface of the cell and are shorter and more numerous than flagella (FIGURE 3.32b). No prokaryotic cells have cilia. Like flagella, cilia are surrounded by the cytoplasmic membrane (they are inside the cell) and are composed of tubulin microtubules arranged in a “9 + 2 ” arrangement of pairs in their shafts and a “9 + 0 ” arrangement of triplets in their basal bodies. A single cell may have hundreds or even thousands of motile cilia. Such cilia beat rhythmically, much like a swimmer doing a butterfly stroke (FIGURE 3.33b). Coordinated beating of cilia propels single-celled eukaryotes through their environment. Cilia are also used within some multicellular eukaryotes to move substances in the local environment past the surface of the cell. For example, such movement of cilia helps cleanse the human respiratory tract of dust and microorganisms. Other Nonmembranous Organelles L E A R N I N G O U TCO M E S 3.39 Describe the structure and function of ribosomes, cyto- Structure and Arrangement Some eukaryotic cells have whiplike extensions called flagella. Flagella of eukaryotes (FIGURE 3.32a) differ structurally and functionally from flagella of prokaryotes. First, eukaryotic flagella are inside the cell; the cytoplasmic membrane completely surrounds a eukaryotic flagellum. Second, the shaft of a eukaryotic flagellum is composed of molecules of a globular protein called tubulin arranged in chains to form hollow microtubules. Nine pairs of microtubules surround two microtubules in the center (FIGURE 3.32c). This “9 + 2 ” arrangement of microtubules is common to all flagellated eukaryotic cells, whether they are found in protozoa, algae, animals, or plants. The filaments of eukaryotic flagella are anchored in the cytoplasm by a basal body, but no hook connects the two parts as it does in prokaryotes. The basal body has triplets of microtubules instead of pairs, and there are no microtubules in the center, so scientists say it has a “9 + 0 ” arrangement of microtubules. Eukaryotic flagella may be single or multiple and are generally found at one pole of the cell. skeletons, and centrioles. 3.40 Compare and contrast the ribosomes of prokaryotes and eukaryotes. 3.41 List and describe the three filaments of a eukaryotic cytoskeleton. Here we discuss three nonmembranous organelles found in eukaryotes: ribosomes and cytoskeleton (both of which are also present in prokaryotes) and centrioles (which are present only in certain kinds of eukaryotic cells). Ribosomes The cytosol of eukaryotes, like that of prokaryotes, is a semitransparent fluid composed primarily of water containing dissolved and suspended proteins, ions, carbohydrates, lipids, and wastes. Within the cytosol of eukaryotic cells are protein-synthesizing ribosomes that are larger than prokaryotic ribosomes; instead of 70S ribosomes, eukaryotic ribosomes are 80S and are composed of 60S and 40S subunits. In addition to the 80S 83 Cytoplasm of Eukaryotes TEM 200 nm Flagellum Cytoplasmic membrane Cytosol Central pair microtubules (a) SEM Microtubules (doublet) 15 μm Cilia “9 + 2” arrangement (c) Cytoplasmic membrane Basal body (b) SEM Portion cut away to show transition area from doublets to triplets and the end of central microtubules 10 μm Microtubules (triplet) “9 + 0” arrangement ▲ FIGURE 3.32 Eukaryotic flagella and cilia. (a) Micrograph of Euglena, which possesses a single flagellum. (b) Scanning electron micrograph of a protozoan, Blepharisma, which has numerous cilia. (c) Details of the arrangement of microtubules of eukaryotic flagella and cilia. Both flagella and cilia have the same internal structure. How do eukaryotic cilia differ from flagella? Figure 3.32 Flagella are longer and less numerous than cilia. ribosomes found within the cytosol, many eukaryotic ribosomes are attached to the membranes of endoplasmic reticulum (discussed shortly). Cytoskeleton Eukaryotic cells contain an extensive cytoskeleton composed of an internal scaffolding of fibers and tubules that help maintain the basic shape of the cell. Eukaryotic cytoskeletons also act to anchor organelles, function in cytoplasmic streaming, and can move organelles within the cytosol. Cytoskeletons in some cells enable the cells to move their cytoplasmic membranes for endocytosis and amoeboid action. Eukaryotic cytoskeletons are made up of tubulin microtubules (also found in flagella and cilia), thinner microfilaments composed of actin, and intermediate filaments composed of various proteins (FIGURE 3.34). Centrioles and Centrosome Animal cells and some fungal cells contain two centrioles, which lie at right angles to each other near the nucleus, in a region of the cytoplasm called the centrosome (FIGURE 3.35). Plants, algae, and most fungi lack centrioles but usually have a region of cytoplasm corresponding to a centrosome. Centrioles are composed of nine triplets of tubulin microtubules arranged in a way that 84 CHAPTER 3 Cell Structure and Function Direction of motion Microtubule Microfilament Actin subunit 7 nm 25 nm Intermediate filament (a) Flagella Protein subunits Tubulin Direction of motion 10 nm (a) (b) Cilia ▲ FIGURE 3.33 Movement of eukaryotic flagella and cilia. (a) Eukaryotic flagella undulate in waves that begin at one end and traverse the length of the flagellum. (b) Cilia move with a power stroke followed by a return stroke. In the power stroke, a cilium is stiff; it relaxes during the return stroke. How is the movement of eukaryotic flagella different from that of prokaryotic flagella? Figure 3.33 Eukaryotic flagella undulate in a wave that moves down the flagellum; the flagella of prokaryotes rotate about the basal body. resembles the “9 + 0 ” arrangement seen at the base of eukaryotic flagella and cilia. Centrosomes play a role in mitosis (nuclear division), cytokinesis (cell division), and the formation of flagella and cilia. However, because many eukaryotic cells that lack centrioles, such as (b) LM ▲ FIGURE 3.34 Eukaryotic cytoskeleton. The cytoskeleton of eukaryotic cells serves to anchor organelles, provides a “track” for the movement of organelles throughout the cell, and provides shape to animal cells. Eukaryotic cytoskeletons are composed of microtubules, microfilaments, and intermediate filaments. (a) Artist’s rendition of cytoskeleton filaments. (b) Various elements of the cytoskeleton shown here have been stained with different fluorescent dyes. DNA in the nucleus is stained yellow-orange. Microtubules Centrosome (made up of two centrioles) Triplet (a) TEM 100 nm 10 μm (b) ▲ FIGURE 3.35 Centrosome. A centrosome is a region of cytoplasm that in animal cells contains two centrioles at right angles to one another; each centriole has nine triplets of microtubules. (a) Transmission electron micrograph of centrosome and centrioles. (b) Artist’s rendition of a centrosome. How do centrioles compare with the basal body and shafts of eukaryotic flagella and cilia (see Figure 3.32c)? Figure 3.35 Centrioles have the same “9 + 0 ” arrangement of microtubules that is found in the basal bodies of eukaryotic cilia and flagella. Cytoplasm of Eukaryotes TABLE 3.5 85 Nonmembranous and Membranous Organelles of Cells General Function Prokaryotes Eukaryotes Ribosomes Protein synthesis Present in all Present in all Cytoskeleton Shape in prokaryotes; support, cytoplasmic streaming, and endocytosis in eukaryotes Present in some Present in all Centrosome Appears to play a role in mitosis, cytokinesis, and formation of flagella and cilia in animal cells Absent in all Present in animals Membranous Organelles Sequester chemical reactions within the cell Nucleus “Control center” of the cell Absent in all Present in all Endoplasmic reticulum Transport within the cell; lipid synthesis Absent in all Present in all Golgi bodies Exocytosis; secretion Absent in all Present in some Lysosomes Breakdown of nutrients; self-destruction of damaged or aged cells Absent in all Present in some Peroxisomes Neutralization of toxins Absent in all Present in some Vacuoles Storage Absent in all Present in some Vesicles Storage, digestion, transport Absent in all Present in all Mitochondria Aerobic ATP production Absent in all Present in most Chloroplasts Photosynthesis Absent in all, though infoldings of cytoplasmic membrane called photosynthetic lamellae have same function in photosynthetic prokaryotes Present in plants and algae Nonmembranous Organelles brown algal sperm and numerous one-celled algae, are still able to form flagella and undergo mitosis and cytokinesis, scientists are still researching the function of centrioles. TABLE 3.5 summarizes characteristics of nonmembranous organelles of cells and contrasts them with characteristics of membranous organelles, which we consider next. MICRO CHECK 27. The ribosomes found in bacteria are significantly different from those in eukaryotes. What is the Svedberg value for uniquely eukaryotic ribosomes? 28. The names of both the largest and smallest cytoskeletal fibers in eukaryotic cells begin with the same prefix, which (perhaps confusingly) means “small.” What is the name of the largest cytoskeletal fibers? Membranous Organelles L E A R N I N G O U TCO M E S 3.42 Discuss the function of each of the following membranous organelles: nucleus, endoplasmic reticulum, Golgi body, lysosome, peroxisome, vesicle, vacuole, mitochondrion, and chloroplast. 3.43 Label the structures associated with each of the membranous organelles. Eukaryotic cells contain a variety of organelles that are surrounded by phospholipid bilayer membranes similar to the cytoplasmic membrane. These membranous organelles include the nucleus, endoplasmic reticulum, Golgi body, lysosomes, peroxisomes, vacuoles, vesicles, mitochondria, and chloroplasts. Prokaryotic cells lack these structures. Nucleus The nucleus is usually spherical to ovoid and is often the largest organelle in a cell17 (FIGURE 3.36). Some eukaryotic cells have a single nucleus; others are multinucleate, while still others lose their nuclei. The nucleus is often referred to as “the control center of the cell” because it contains most of the cell