Chapter 25: Drugs Affecting Renal Excretory Function PDF
Document Details
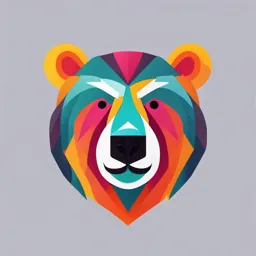
Uploaded by FavoriteObsidian9433
Riga Stradiņš University
Edwin K. Jackson
Tags
Summary
This document describes the pharmacological basis of therapeutics related to drugs affecting renal excretory function. It covers renal physiology, introducing diuretics, vasopressin, and various drug mechanisms related to water homeostasis and plasma osmolality.
Full Transcript
Access Provided by: Goodman & Gilman's: The Pharmacological Basis of Therapeutics, 13e Chapter 25: Drugs Affecting Renal Excretory Function Edwin K. Jackson INTRODUCTION The kidney filters the extracellular fluid volume across the renal glomeruli an average of 12 times a day, and the renal nephrons...
Access Provided by: Goodman & Gilman's: The Pharmacological Basis of Therapeutics, 13e Chapter 25: Drugs Affecting Renal Excretory Function Edwin K. Jackson INTRODUCTION The kidney filters the extracellular fluid volume across the renal glomeruli an average of 12 times a day, and the renal nephrons precisely regulate the fluid volume of the body and its electrolyte content via processes of secretion and reabsorption. Disease states such as hypertension, heart failure, renal failure, nephrotic syndrome, and cirrhosis may disrupt this balance. Diuretics increase the rate of urine flow and Na+ excretion and are used to adjust the volume or composition of body fluids in these disorders. Precise regulation of body fluid osmolality is also essential. It is controlled by a finely tuned homeostatic mechanism that operates by adjusting both the rate of water intake and the rate of solutefree water excretion by the kidneys—that is, water balance. Abnormalities in this homeostatic system can result from genetic diseases, acquired diseases, or drugs and may cause serious and potentially lifethreatening deviations in plasma osmolality. Part I of this chapter first describes renal physiology, then introduces diuretics with regard to mechanism and site of action, effects on urinary composition, and effects on renal hemodynamics, and then integrates diuretic pharmacology with a discussion of mechanisms of edema formation and the role of diuretics in clinical medicine. Specific therapeutic applications of diuretics are presented in Chapters 28 (hypertension) and 29 (heart failure). Part II of this chapter describes the vasopressin system that regulates water homeostasis and plasma osmolality and factors that perturb those mechanisms and examines pharmacological approaches for treating disorders of water balance. ABBREVIATIONS Abbreviations A A : arachidonic acid ACTH: corticotropin (previously adrenocorticotropic hormone) ADH: antidiuretic hormone AIP: aldosteroneinduced protein Aldo: aldosterone Ang: angiotensin ANP: atrial natriuretic peptide A T L : ascending thin limb AVP: arginine vasopressin BL: basolateral membrane BNP: brain natriuretic peptide CA: carbonic anhydrase cGMP: cyclic guanosine monophosphate CHF: congestive heart failure Downloaded 2024417 9:35 A Your IP is 81.198.192.190 Chapter 25: Drugs Affecting Renal Excretory Function, Edwin K. Jackson CNGC:McGraw cyclic nucleotidegated cation channel ©2024 Hill. All Rights Reserved. Terms of Use Privacy Policy Notice Accessibility CNP: Ctype natriuretic peptide Page 1 / 46 BNP: brain natriuretic peptide CA: carbonic anhydrase Access Provided by: cGMP: cyclic guanosine monophosphate CHF: congestive heart failure CNGC: cyclic nucleotidegated cation channel CNP: Ctype natriuretic peptide CNT: connecting tubule COX: cyclooxygenase DAG: diacyglycerol DCT: distal convoluted tubule DDAVP: 1deamino8DAVP (desmopressin) DI: diabetes insipidus D T L : descending thin limb ECFV: extracellular fluid volume ENaC: epithelial Na+ channel ENCC1 or TSC: the absorptive Na+Cl− symporter ENCC2, NKCC2, or BSC1: the absorptive Na+K+2Cl− ENCC3, NKCC1, or BSC2: the secretory symporter FDA: Food and Drug Administration F F : filtration fraction GFR: glomerular filtration rate GPCR: G protein–coupled receptor GTP: guanosine triphosphate HCTZ: hydrochlorothiazide HDL: highdensity lipoprotein HSD: 11βhydroxysteroid dehydrogenase IMCD: inner medullary collecting duct I P3 : inositol trisphosphate LDL: lowdensity lipoprotein L M : luminal membrane LOX: lipoxygenase L T : leukotriene M R : mineralocorticoid receptor Downloaded 2024417 9:35 A Your IP is 81.198.192.190 MRA: mineralocorticoid receptor antagonist Chapter 25: Drugs Affecting Renal Excretory Function, Edwin K. Jackson ©2024 Hill.RNA All Rights Reserved. Terms of Use Privacy Policy Notice Accessibility mRNA:McGraw messenger NP: natriuretic peptide Page 2 / 46 LOX: lipoxygenase L T : leukotriene Access Provided by: M R : mineralocorticoid receptor MRA: mineralocorticoid receptor antagonist mRNA: messenger RNA NP: natriuretic peptide NPA: asparagineprolinealanine NPR_: natriuretic peptide receptor _ (e.g., NPRA, B, or C) NSAID: nonsteroidal antiinflammatory drug O A T : organic anion transporter P A : phosphatidic acid PG: prostaglandin PK_: protein kinase _ (e.g. PKA, PKB, PKG) PL_: phospholipase _ (e.g., PLC, PLD) PTH: parathyroid hormone PVN: paraventricular nucleus RAAS: reninangiotensinaldosterone system RAS: reninangiotensin system RBF: renal blood flow SGK1: serum and glucocorticoidstimulated kinase 1 SIADH: syndrome of inappropriate secretion of ADH SNS: sympathetic nervous system SON: supraoptic nucleus T A L : thick ascending limb TGF: tubuloglomerular feedback T X : thromboxane VP: vasopressin VRUT: vasopressinregulated urea transporter vWD: von Willebrand disease WCV: water channelcontaining vesicle PART I: RENAL PHYSIOLOGY AND DIURETIC DRUG ACTION Renal Anatomy and Physiology The basic urineforming ofAtheYour kidney is the nephron. The initial part of the nephron, the renal (Malpighian) corpuscle, consists of a capsule Downloaded 2024417unit 9:35 IP is 81.198.192.190 Page and 3 / 46 Chapter 25:capsule) Drugs Affecting Excretory Edwin K. Jackson (Bowman’s and a tuftRenal of capillaries (theFunction, glomerulus) residing within the capsule. The glomerulus receives blood from an afferent arteriole, ©2024exits McGraw Hill. All Rights TermsUltrafiltrate of Use Privacy Policy Notice Accessibility blood the glomerulus via anReserved. efferent arteriole. produced by the glomerulus collects in the space between the glomerulus and capsule (Bowman’s space) and enters a long tubular portion of the nephron, where the ultrafiltrate is reabsorbed and conditioned. Each human kidney is composed of about 1 million nephrons. Figure 25–1 illustrates subdivisions of the nephron. PART I: RENAL PHYSIOLOGY AND DIURETIC DRUG ACTION Access Provided by: Renal Anatomy and Physiology The basic urineforming unit of the kidney is the nephron. The initial part of the nephron, the renal (Malpighian) corpuscle, consists of a capsule (Bowman’s capsule) and a tuft of capillaries (the glomerulus) residing within the capsule. The glomerulus receives blood from an afferent arteriole, and blood exits the glomerulus via an efferent arteriole. Ultrafiltrate produced by the glomerulus collects in the space between the glomerulus and capsule (Bowman’s space) and enters a long tubular portion of the nephron, where the ultrafiltrate is reabsorbed and conditioned. Each human kidney is composed of about 1 million nephrons. Figure 25–1 illustrates subdivisions of the nephron. Figure 25–1 Anatomy and nomenclature of the nephron. Glomerular Filtration In the glomerular capillaries, a portion of plasma water is forced through a filter that has three basic components: the fenestrated capillary endothelial Downloaded 2024417 9:35 A Your IP is 81.198.192.190 cells, a basement membrane just beneath the endothelial cells, and the filtration slit diaphragms formed by epithelial cells that cover the Page basement 4 / 46 Chapter 25: Drugs Affectinglying Renal Excretory Function, Edwin K. Jackson ©2024 McGraw All Rights Reserved. Terms Use Privacy Policy Notice Accessibility membrane on its Hill. urinary space side. Solutes of smallof size flow with filtered water (solvent drag) into Bowman’s space, whereas formed elements and macromolecules are retained by the filtration barrier. Glomerular Filtration Access Provided by: In the glomerular capillaries, a portion of plasma water is forced through a filter that has three basic components: the fenestrated capillary endothelial cells, a basement membrane lying just beneath the endothelial cells, and the filtration slit diaphragms formed by epithelial cells that cover the basement membrane on its urinary space side. Solutes of small size flow with filtered water (solvent drag) into Bowman’s space, whereas formed elements and macromolecules are retained by the filtration barrier. Overview of Nephron Function The kidney filters large quantities of plasma, reabsorbs substances that the body must conserve, and leaves behind or secretes substances that must be eliminated. The changing architecture and cellular differentiation along the length of a nephron are crucial to these functions (see Figure 25–1). The two kidneys in humans together produce about 120 mL of ultrafiltrate/min, yet only 1 mL of urine/min of urine; more than 99% of the glomerular ultrafiltrate is reabsorbed at a staggering energy cost. The kidneys consume 7% of totalbody O2 intake despite comprising only 0.5% of body weight. The proximal tubule is contiguous with Bowman’s capsule and takes a tortuous path until finally forming a straight portion that dives into the renal medulla. Normally, about 65% of filtered Na+ is reabsorbed in the proximal tubule, and because this part of the tubule is highly permeable to water, reabsorption is essentially isotonic. Between the outer and inner strips of the outer medulla, the tubule abruptly changes morphology to become the DTL, which penetrates the inner medulla, makes a hairpin turn, and then forms the ATL. At the juncture between the inner and outer medulla, the tubule once again changes morphology and becomes the TAL. Together, the proximal straight tubule, DTL, ATL, and TAL segments are known as the loop of Henle. The DTL is highly permeable to water, yet its permeabilities to NaCl and urea are low. In contrast, the ATL is permeable to NaCl and urea but is impermeable to water. The TAL actively reabsorbs NaCl but is impermeable to water and urea. Approximately 25% of filtered Na+ is reabsorbed in the loop of Henle, mostly in the TAL, which has a large reabsorptive capacity. The TAL passes between the afferent and efferent arterioles and makes contact with the afferent arteriole by means of a cluster of specialized columnar epithelial cells known as the macula densa. The macula densa is strategically located to sense concentrations of NaCl leaving the loop of Henle. If the concentration of NaCl is too high, the macula densa sends a chemical signal (perhaps adenosine or ATP) to the afferent arteriole of the same nephron, causing it to constrict, thereby reducing the GFR. This homeostatic mechanism, known as TGF, protects the organism from salt and volume wasting. The macula densa also regulates renin release from the adjacent juxtaglomerular cells in the wall of the afferent arteriole. Approximately 0.2 mm past the macula densa, the tubule changes morphology once again to become the DCT. Like the TAL, the DCT actively transports NaCl and is impermeable to water. Because these characteristics impart the capacity to produce dilute urine, the TAL and the DCT are collectively called the diluting segment of the nephron, and the tubular fluid in the DCT is hypotonic regardless of hydration status. However, unlike the TAL, the DCT does not contribute to the countercurrentinduced hypertonicity of the medullary interstitium (described in material that follows). The collecting duct system (segments 10–14 in Figure 25–1) is an area of fine control of ultrafiltrate composition and volume. It is here that final adjustments in electrolyte composition are made, a process modulated by the adrenal steroid aldosterone. Vasopressin (also called ADH) modulates water permeability of this part of the nephron as well. The more distal portions of the collecting duct pass through the renal medulla, where the interstitial fluid is markedly hypertonic. In the absence of ADH, the collecting duct system is impermeable to water, and dilute urine is excreted. In the presence of ADH, the collecting duct system is permeable to water, and water is reabsorbed. The movement of water out of the tubule is driven by the steep concentration gradient that exists between tubular fluid and medullary interstitium. The hypertonicity of the medullary interstitium plays a vital role in the capacity of mammals and birds to concentrate urine, which is accomplished by a combination of the unique topography of the loop of Henle and the specialized permeabilities of the loop’s subsegments. The “passive countercurrent multiplier hypothesis” proposes that active transport in the TAL concentrates NaCl in the interstitium of the outer medulla. Because this segment of the nephron is impermeable to water, active transport in the ascending limb dilutes the tubular fluid. As the dilute fluid passes into the collecting duct system, water is extracted if, and only if, ADH is present. Because the cortical and outer medullary collecting ducts have low permeability to urea, urea is concentrated in the tubular fluid. The IMCD, however, is permeable to urea, so urea diffuses into the inner medulla, where it is trapped by countercurrent exchange in the vasa recta (medullary capillaries that run parallel to the loop of Henle). Because the DTL is impermeable to salt and urea, the high urea concentration in the inner medulla extracts water from the DTL and concentrates NaCl in the tubular fluid of the DTL. As the tubular fluid enters the ATL, NaCl diffuses out of the saltpermeable ATL, thus contributing to the hypertonicity of the medullary interstitium. General Mechanism of Renal Epithelial Transport There are multiple mechanisms by which solutes may cross cell membranes (see Figure 5–4). The kinds of transport achieved in a nephron segment depend mainly on which transporters are present and whether they are embedded in the luminal or basolateral membrane. Figure 25–2 presents a Downloaded 9:35 A Your IPthat is 81.198.192.190 general model2024417 of renal tubular transport be summarized as follows: Page 5 / 46 Chapter 25: Drugs Affecting Renal Excretory Function, Edwin K. Jackson ©2024+ McGraw Hill. All Rights Reserved. Terms of Use Privacy Policy Notice Accessibility 1. Na , K+ATPase (sodium pump) in the basolateral membrane transports Na+ into the intercellular and interstitial spaces and K+ into the cell, establishing an electrochemical gradient for Na+ across the cell membrane directed inward. concentration in the inner medulla extracts water from the DTL and concentrates NaCl in the tubular fluid of the DTL. As the tubular fluid enters the ATL, NaCl diffuses out of the saltpermeable ATL, thus contributing to the hypertonicity of the medullary interstitium. General Mechanism of Renal Epithelial Transport Access Provided by: There are multiple mechanisms by which solutes may cross cell membranes (see Figure 5–4). The kinds of transport achieved in a nephron segment depend mainly on which transporters are present and whether they are embedded in the luminal or basolateral membrane. Figure 25–2 presents a general model of renal tubular transport that be summarized as follows: 1. Na+, K+ATPase (sodium pump) in the basolateral membrane transports Na+ into the intercellular and interstitial spaces and K+ into the cell, establishing an electrochemical gradient for Na+ across the cell membrane directed inward. 2. Na+ can diffuse down this Na+ gradient across the luminal membrane via Na+ channels and via membrane symporters that use the energy stored in the Na+ gradient to transport solutes out of the tubular lumen and into the cell (e.g., Na+glucose, Na+H2PO4, and Na+amino acid) and antiporters (e.g., Na+H+) that move solutes into the lumen as Na+ moves down its gradient and into the cell. 3. Na+ exits the basolateral membrane into intercellular and interstitial spaces via the Na+ pump. 4. The action of Na+linked symporters in the luminal membrane causes the concentration of substrates for these symporters to rise in the epithelial cell. These substrate/solute gradients then permit simple diffusion or mediated transport (e.g., symporters, antiporters, uniporters, and channels) of solutes into the intercellular and interstitial spaces. 5. Accumulation of Na+ and other solutes in the intercellular space creates a small osmotic pressure differential across the epithelial cell. In water permeable epithelium, water moves into the intercellular spaces driven by the osmotic pressure differential. Water moves through aqueous pores in both the luminal and the basolateral cell membranes, as well as through tight junctions (paracellular pathway). Bulk water flow carries some solutes into the intercellular space by solvent drag. 6. Movement of water into the intercellular space concentrates other solutes in the tubular fluid, resulting in an electrochemical gradient for these substances across the epithelium. Membranepermeable solutes then move down their electrochemical gradients into the intercellular space by both the transcellular (e.g., simple diffusion, symporters, antiporters, uniporters, and channels) and paracellular pathways. Membraneimpermeable solutes remain in the tubular lumen and are excreted in the urine with an obligatory amount of water. 7. As water and solutes accumulate in the intercellular space, hydrostatic pressure increases, thus providing a driving force for bulk water flow. Bulk water flow carries solute out of the intercellular space into the interstitial space and, finally, into the peritubular capillaries. Figure 25–2 Generic mechanism of renal epithelial cell transport (see text for details). A, antiporter; ATPase, Na+, K+ATPase (sodium pump); CH, ion channel; I, membraneimpermeable solutes; P, membranepermeable solutes; PD, potential difference across indicated membrane or cell; S, symporter; U, uniporter; WP, water pore; X and Y, transported solutes. Downloaded 2024417 9:35 A Your IP is 81.198.192.190 Chapter 25: Drugs Affecting Renal Excretory Function, Edwin K. Jackson ©2024 McGraw Hill. All Rights Reserved. Terms of Use Privacy Policy Notice Accessibility Page 6 / 46 Generic mechanism of renal epithelial cell transport (see text for details). A, antiporter; ATPase, Na+, K+ATPase (sodium pump); CH, ion channel; I, membraneimpermeable solutes; P, membranepermeable solutes; PD, potential difference across indicated membrane or cell; S, symporter; U, Access Provided by: uniporter; WP, water pore; X and Y, transported solutes. Organic Acid and Organic Base Secretion The kidney is a major organ involved in the elimination of organic chemicals from the body. Organic molecules may enter the renal tubules by glomerular filtration or may be actively secreted directly into tubules. The proximal tubule has a highly efficient transport system for organic acids and an equally efficient but separate transport system for organic bases. Current models for these secretory systems are illustrated in Figure 25–3. Both systems are powered by the sodium pump in the basolateral membrane, involve secondary and tertiary active transport, and use a facilitated diffusion step. There are many organic acid and organic base transporters (see Chapter 5). A family of OATs links countertransport of organic anions with dicarboxylates (Figure 25–3A). Figure 25–3 Mechanisms of organic acid (A) and organic base (B) secretion in the proximal tubule. The numbers 1, 2, and 3 refer to primary, secondary, and tertiary active transport, respectively. A−, organic acid (anion); C+, organic base (cation); αKG2−, αketoglutarate but also other dicarboxylates. BL and LM indicate basolateral and luminal membranes, respectively. Downloaded 2024417 9:35 A Your IP is 81.198.192.190 Chapter 25: Drugs Affecting Renal Excretory Function, Edwin K. Jackson ©2024 McGraw Hill. All Rights Reserved. Terms of Use Privacy Policy Notice Accessibility Page 7 / 46 Mechanisms of organic acid (A) and organic base (B) secretion in the proximal tubule. The numbers 1, 2, and 3 refer to primary, secondary, and tertiary active transport, respectively. A−, organic acid (anion); C+, organic base (cation); αKG2−, αketoglutarate but also other dicarboxylates. BL and LM indicate Access Provided by: basolateral and luminal membranes, respectively. Renal Handling of Specific Anions and Cations Reabsorption of Cl− generally follows reabsorption of Na+. In segments of the tubule with lowresistance tight junctions (i.e., “leaky” epithelium), such as the proximal tubule and TAL, Cl− movement can occur paracellularly. Cl− crosses the luminal membrane by antiport with formate and oxalate (proximal tubule), symport with Na+/K+ (TAL), symport with Na+ (DCT), and antiport with HCO3 (collecting duct system). Cl− crosses the basolateral membrane via symport with K+ (proximal tubule and TAL), antiport with Na+/HCO3 (proximal tubule), and Cl− channels (TAL, DCT, collecting duct system). Of filtered K+, 80%–90% is reabsorbed in the proximal tubule (diffusion and solvent drag) and TAL (diffusion), largely through the paracellular pathway. The DCT and collecting duct system secrete variable amounts of K+ by a channelmediated pathway. Modulation of the rate of K+ secretion in the collecting duct system, particularly by aldosterone, allows urinary K+ excretion to be matched with dietary intake. The transepithelial potential difference VT, lumen positive in the TAL and lumen negative in the collecting duct system, drives K+ reabsorption and secretion, respectively. Most of the filtered Ca2+ (∼70%) is reabsorbed by the proximal tubule by passive diffusion through a paracellular route. Another 25% of filtered Ca2+ is reabsorbed by the TAL in part by a paracellular route driven by the lumenpositive VT and in part by active transcellular Ca2+ reabsorption modulated by PTH (see Chapter 43). Most of the remaining Ca2+ is reabsorbed in DCT by a transcellular pathway. The transcellular pathway in the TAL and DCT involves passive Ca2+ influx across the luminal membrane through Ca2+ channels (TRPV5, transient receptor potential cation channel V5), followed by Ca2+ extrusion across the basolateral membrane by a Ca2+ATPase. Also, in DCT and CNT, Ca2+ crosses the basolateral membrane by Na+Ca2+ exchanger (antiport). Pi is largely reabsorbed (80% of filtered load) by the proximal tubule. The Na+Pi symporter uses the free energy of the Na+ electrochemical gradient to transport Pi into the cell. The Na+Pi symporter is inhibited by PTH. The renal tubules reabsorb HCO3 and secrete protons (tubular acidification), thereby participating in acidbase balance. These processes are described in the section on carbonic anhydrase inhibitors. Principles of Diuretic Action Diuretics are drugs that increase the rate of urine flow; clinically useful diuretics also increase the rate of Na+ excretion (natriuresis) and of an accompanying anion, usually Cl−. Most clinical applications of diuretics are directed toward reducing extracellular fluid volume by decreasing totalbody NaCl content. Although continued diuretic administration causes a sustained net deficit in totalbody Na+, the time course of natriuresis is finite because renal compensatory mechanisms bring Na+ excretion in line with Na+ intake, a phenomenon known as diuretic braking. These compensatory mechanisms include activation of the sympathetic nervous system, activation of the reninangiotensinaldosterone axis, decreased arterial blood pressure (which reduces pressure natriuresis), renal epithelial cell hypertrophy, increased renal epithelial transporter expression, and perhaps alterations in natriuretic hormones such as ANP. The net effects on extracellular volume and body weight are shown in Figure 25–4. Figure 25–4 Downloaded 2024417 9:35 A Your IP is 81.198.192.190 Changes fluid volume and weightFunction, with diuretic therapy. The period of diuretic administration is shown in the shaded box alongPage with its 8 / 46 Chapter in 25:extracellular Drugs Affecting Renal Excretory Edwin K. Jackson 2+ 2+ ©2024on McGraw Hill. All Reserved. Use Policy Accessibility effects body weight inRights the upper part of theTerms figureof and Na Privacy excretion in the Notice lower half of the figure. Initially, when Na excretion exceeds intake, body weight and ECFV decrease. Subsequently, a new steady state is achieved where Na+ intake and excretion are equal but at a lower ECFV and body weight. This results from activation of the RAAS and SNS, “the braking phenomenon.” When the diuretic is discontinued, body weight and ECFV rise during a include activation of the sympathetic nervous system, activation of the reninangiotensinaldosterone axis, decreased arterial blood pressure (which reduces pressure natriuresis), renal epithelial cell hypertrophy, increased renal epithelial transporter expression, and perhaps alterations in natriuretic hormones such as ANP. The net effects on extracellular volume and body weight are shown in Figure 25–4. Access Provided by: Figure 25–4 Changes in extracellular fluid volume and weight with diuretic therapy. The period of diuretic administration is shown in the shaded box along with its effects on body weight in the upper part of the figure and Na2+ excretion in the lower half of the figure. Initially, when Na2+ excretion exceeds intake, body weight and ECFV decrease. Subsequently, a new steady state is achieved where Na+ intake and excretion are equal but at a lower ECFV and body weight. This results from activation of the RAAS and SNS, “the braking phenomenon.” When the diuretic is discontinued, body weight and ECFV rise during a period when Na2+ intake exceeds excretion. A new steady state is then reached as stimulation of the RAAS and SNS wane. Diuretics may modify renal handling of other cations (e.g., K+, H+, Ca2+, and Mg2+), anions (e.g., Cl−, HCO3, and H2PO4), and uric acid. In addition, diuretics may alter renal hemodynamics indirectly. Table 25–1 compares the general effects of the major diuretic classes. Table 25–1 Excretory and Renal Hemodynamic Effects of Diureticsa DIURETIC CATIONS ANIONS URIC ACID RENAL HEMODYNAMICS MECHANISM (Primary site of N a+ K+ H+ b C a2 + M g2 + C l− HCO3 − H 2 P O4 − Acute Chronic RBF GFR FF TGF + ++ – NC V (+) ++ ++ I – – – NC + ++ + I + ++ + + + + I + NC – I (loop of Henle) Downloaded 2024417 9:35 A Your IP is 81.198.192.190 Chapter 25: Drugs Affecting Renal Excretory Function, Edwin K. Jackson ©2024Inhibitors McGrawofHill. Reserved. Policy Notice Accessibility Na+All Rights ++ ++ + Terms of ++Use Privacy ++ ++ +c +c + – V(+) NC V(–) action) Inhibitors of CA (proximal tubule) Osmotic diuretics K+2Cl– symport Page 9 / 46 – Diuretics may modify renal handling of other cations (e.g., K+, H+, Ca2+, and Mg2+), anions (e.g., Cl−, HCO3, and H2PO4), and uric acid. In addition, diuretics Access Provided by: may alter renal hemodynamics indirectly. Table 25–1 compares the general effects of the major diuretic classes. Table 25–1 Excretory and Renal Hemodynamic Effects of Diureticsa DIURETIC CATIONS ANIONS URIC ACID RENAL HEMODYNAMICS MECHANISM (Primary site of action) Inhibitors of CA N a+ K+ H+ b C a2 + M g2 + C l− HCO3 − H 2 P O4 − Acute Chronic RBF GFR FF TGF + ++ – NC V (+) ++ ++ I – – – NC + ++ + I + ++ + + + + I + NC – I ++ ++ + ++ ++ ++ +c +c + – V(+) NC V(–) – + ++ + V(–) V(+) + +c +c + – NC V(–) V(–) NC + – – – – + (+) NC I – NC NC NC NC + – – I – + (+) I I – NC NC NC NC (proximal tubule) Osmotic diuretics (loop of Henle) Inhibitors of Na+ K+2Cl– symport (thick ascending limb) Inhibitors of Na+ Cl– symport (distal convoluted tubule) Inhibitors of renal epithelial Na+ channels (late distal tubule, collecting duct) Antagonists of mineralocorticoid receptors (late distal tubule, collecting duct) a Except for uric acid, changes are for acute effects of diuretics in the absence of significant volume depletion, which would trigger complex physiological adjustments. b H+ includes titratable acid and NH4+. c In general, these effects are restricted to those individual agents that inhibit carbonic anhydrase. However, there are notable exceptions in which symport inhibitors increase bicarbonate and phosphate (e.g., metolazone, bumetanide). ++, +, (+),–, NC, V, V(+), V(–) and I indicate marked increase, mildtomoderate increase, slight increase, decrease, no change, variable effect, variable increase, variable decrease, and insufficient data, respectively. For cations and anions, the indicated effects refer to absolute changes in fractional excretion. Inhibitors Carbonic Downloaded of 2024417 9:35Anhydrase A Your IP is 81.198.192.190 Chapter 25: Drugs Affecting Renal Excretory Function, Edwin K. Jackson ©2024are McGraw Hill. All Rights Reserved. of Use Privacyacetazolamide, Policy Noticedichlorphenamide, Accessibility and methazolamide (Table 25–2). There three orally administered carbonic Terms anhydrase inhibitors— Table 25–2 Page 10 / 46 In general, these effects are restricted to those individual agents that inhibit carbonic anhydrase. However, there are notable exceptions in which symport inhibitors increase bicarbonate and phosphate (e.g., metolazone, bumetanide). ++, +, (+),–, NC, V, V(+), V(–) and I indicate marked increase, mildtomoderate increase, slight increase, decrease, no change, variable effect, variable increase, variable decrease, and insufficient data, respectively. For cations and anions, the indicated effects refer to absolute changes in fractional excretion. Access Provided by: Inhibitors of Carbonic Anhydrase There are three orally administered carbonic anhydrase inhibitors—acetazolamide, dichlorphenamide, and methazolamide (Table 25–2). Table 25–2 Inhibitors of Carbonic Anhydrase DRUG RELATIVE POTENCY ORAL AVAILABILITY t 1 / 2 (hours) ROUTE OF ELIMINATION Acetazolamide 1 ~100% 6–9 R Dichlorphenamide 30 ID ID ID Methazolamide >1;