Chapter 2: 12 Principles of Green Chemistry PDF
Document Details
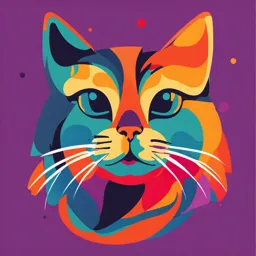
Uploaded by FreedSavannah2059
Oran 1 University - Ahmed Ben Bella
Tags
Summary
This chapter introduces the 12 principles of green chemistry, aiming to minimize the environmental impact of chemical processes. It provides examples, methodologies, and alternative approaches.
Full Transcript
2 CHAPTER 2 12 principles of green chemistry Introduction The term “Green Chemistry” was introduced for the first time by Anastas in 1991 in a special program created by the US Environmental Protection Agency (EPA) in order to stimulat...
2 CHAPTER 2 12 principles of green chemistry Introduction The term “Green Chemistry” was introduced for the first time by Anastas in 1991 in a special program created by the US Environmental Protection Agency (EPA) in order to stimulate a substantial development in chemistry and chemical technology. The program was also aimed at changing the outlook of chemists and was directed at protecting the environment by focusing on lower risks or their complete elimination as far as human health is concerned. 1. The concept of green chemistry The Green chemistry concept appeared in the USA as a general scientific program, originating from the interdisciplinary cooperation of research groups in universities, independent research groups, scientific societies and government agencies, with members of each of these bodies having their own program dedicated to lowering levels of environmental pollution. Green chemistry comprises a new approach to the synthesis, processing and application of chemical substances, thus diminishing the hazards for human health and environmental pollution. Green Chemistry can be comprehensively illustrated as a set of 12 principles which were proposed by Anastas and Warner. These principles include instructions for professional chemists concerning the creation of new substances, new syntheses and new technological processes. 43 2 CHAPTER 2 12 principles of green chemistry The first principle describes the basic idea of Green Chemistry environmental protection from pollution. The other principles focus on such problems as atom economy, toxicity, solvents, energy consumption, use of raw materials from renewable resources and decomposition of the chemical products to simple non-toxic substances that are compatible with the environment. 2. The 12 principles of green chemistry 2.1. Prevention It is better to prevent the formation of waste materials and/or by products than to process or clean them. The organic syntheses in the absence of solvents have stimulated so-called “grinding chemistry”, in which the reagents are mixed without solvent, sometimes by simply grinding them in a mortar. Researches described a good example of a three-component Friedel–Crafts reaction on indoles, leading to the functionalized indole. In a similar way, the synthesis of 4-quinazolinone was developed using a rapid method without solvent. “Grinding chemistry” has recently been reviewed. An expanding area of chemistry without solvents involves the use of microwaves to irradiate mixtures of neat reagents. One example of this approach is the synthesis of 4,4'-diaminotriphenyl-methanes using microwave irradiation (Scheme 2.1). 2.2. Atom economy Synthetic methods should be designed in such a way that all products participating in the reaction process are included in the final product. Chemists all over the world consider a reaction to be “perfect‟ when the yield is 90% or more. However, such a reaction could create considerable amounts of waste. The concept of atom economy was developed by Trost and is represented as follows: 44 2 CHAPTER 2 12 principles of green chemistry % = × 100 HN O Et O NH2 O Et + + H O Solvent free N N H H 76% O O OMe NH2 OH OMe La(NO 3)3,6H2O N + + H OMe 5 min NH2 N 98% R1 R3 R3 R2 N N R2 R1 R3 R5 R2 O PhN2HCl N + C MW R1 H R4 Solvent free R4 R5 R1-R5 are alkyls or substituted alkyls Scheme 2.1: Organic syntheses in the absence of solvent O OH 200 °C Scheme 2.2: Allylic rearrangement with 100% atom economy This reaction has an atom economy of 100% because all reactants are included in the final product (Scheme 2.2). + " → " + Scheme 2.3: Preparation of an amide with 65.4% atom economy 45 2 CHAPTER 2 12 principles of green chemistry In the precedent reaction (Scheme 2.3), the leaving group (OC2H5) and a proton from the methylamine are not used. The rest of the atoms are used and for this reason: 87.106 % = × 100 = 65.40% 133.189 The most important principle of green chemistry is to eliminate or at least to decrease the formation of hazardous products, which can be toxic or detrimental to the environment. 2.3. Less hazardous chemical syntheses Wherever practicable, synthetic methods should be designed to use and generate substances that possess little or no toxicity to human health and the environment. An example of this principle is the oxidation of cyclohexene to adipic acid with 30% hydrogen peroxide (Scheme 2.4). Scheme 2.4: Oxidation of cyclohexene to adipic acid with 30% hydrogen peroxide 2.4. Designing safer chemicals The design of products should be safe in terms of human health and the environment. A typical example of a hazardous drug is thalidomide (Scheme 2.5), which was introduced in 1961 in West Germany. This drug was prescribed to 46 2 CHAPTER 2 12 principles of green chemistry pregnant women against nausea and vomiting. Pregnant women who had taken the drug gave birth to babies with a condition called phocomelia–abnormally short limbs with toes sprouting from the hips and flipper-like arms. Other infants had eye and ear defects or malformed internal organs such as unsegmented small or large intestines. This drug is now prescribed for treatment of patients with multiple myeloma and for the acute treatment of the cutaneous manifestations of erythema nodosum leprosum. O N O N O O H Scheme 2.5: Chemical structure of Thalidomide-2-(2,6-dioxopiperidin-3-yl)-1,3-dione Dow AgroSciences designed spinosad, a highly selective, environmentally friendly insecticide. Spinosad demonstrates both rapid contact and ingestion activity in insects, which is unusual for a biological product (Scheme 2.6). Spinosad has a favorable environmental profile. It does not leach, bioaccumulate, volatilize, or persist in the environment. Spinosad will degrade photo-chemically when exposed to light after application. Spinosad strongly adsorbs to soils and, as a result, it does not leach through soil to groundwater when used properly and buffer zones are not required. Spinosad has a relatively low toxicity to mammals and birds and, although it is moderately toxic to fish, this toxicity represents a reduced risk to fish when compared with many synthetic insecticides currently in use. In addition, 70–90% of beneficial insects and predatory wasps are left unharmed by spinosad. The unique mode of action of spinosad, coupled with the high degree of activity on targeted pests, low toxicity to non-target organisms (including many beneficial arthropods), and 47 2 CHAPTER 2 12 principles of green chemistry resistance management properties make spinosad an excellent new tool for integrated pest management. Spinosad is an example of a technological development that demonstrates how the creation and production of safer chemicals is possible. Changes in the chemical structure are the means to achieve this goal. H3 C H3 C N O CH3 CH3 O CH3 H H O O OCH3 OCH3 H3CO Et O O H H H R Spinosyn A: R = H; Spinosyn D: R = CH 3 Spinosad is a mixture of spinosyn A and spinosyn D Scheme 2.6: Chemical structure of Spinosad an environmental friendly insecticide 2.5. Safer solvents and auxiliaries The solvent chosen for a given reaction should not pollute the environment or be hazardous to human health. The use of ionic liquids or supercritical CO2 is recommended. If possible, the reaction should be carried out in an aqueous phase or in the absence of solvent. A better method is to conduct the reaction in the solid phase and one example of this approach is the preparation of styryl dyes. A series of styrylpyridinium, styrylquinolinium and styrylbenzothiazolium dyes have been synthesized by novel environmentally benign procedures. The condensation of 4- methylpyridinium methosulfate, 2- or 4-methylquinolinium methosulfate or 2- methylbenzothiazolium methosulfate with aromatic aldehydes was performed under 48 2 CHAPTER 2 12 principles of green chemistry solvent-free conditions and microwave irradiation in the presence of different basic or acidic reagents (Scheme 2.7). H3 C N CH3 O CH3 NaOH (catalyst), 1-3 drops H2O + C N H 3C N CH3 MW, 2 min z- H CH3 N CH3SO4- CH3 96% Scheme 2.7: Preparation of styryl dyes under solvent-free conditions and microwave irradiation Another example of this approach is the preparation of brominated anilines and phenols in the solid phase (Scheme 2.8). O OH NH 2 Br Br NH 2 Br Br Phase solide + + Br NO2 NO2 Br Br Br Scheme 2.8: Bromination of anilines in the solide phase The volatility of solvents is also a fundamental problem as these materials can be hazardous to human health and the environment. One possibility of overcoming this problem is the use of immobilized solvents or solvents with low volatility, e.g. ionic liquids, and the use of these systems is growing. 2.6. Energy efficiency The energy requirements involved in the chemical processes should be accounted for, in view of their influence on the environment and the economic balance, and the energy requirements should be diminished. If possible, the chemical processes should be carried out at room temperature and atmospheric pressure. The reaction energy could be photochemical, microwave or ultrasonic irradiation. A boom is currently occurring in the use of these green energy sources and this is also 49 2 CHAPTER 2 12 principles of green chemistry associated with a marked decrease in the reaction time, to higher yields and, very often, to higher product purity. A number of azaheterocycles (i.e. pyrrole, imidazole, indole and carbazole) react remarkably quickly with alkyl halides to give exclusively N-alkyl derivatives under microwave conditions (Scheme 2.9). N N K2CO3/KOH, Tetrabutylammonium bromide + R-X N MW, 30 s, 1 min N H R 73-89% R: different alkyls X: Cl, Br K2CO3, Tetrabutylammonium bromide + R-X MW, 4-10 min N N H R 79-95% Scheme 2.9: N-Alkylation azaheterocycles under microwave conditions A series of imines was synthesized by an ultrasound-assisted reaction of aldehydes and primary amines using silica as the promoter (Scheme 2.10). R1 O N + R1NH2 Promoter R R Scheme 2.10: Preparation of imines using alternative energy source 2.7. Use of renewable feedstocks The intermediates and materials should be renewable rather than depleting (which is the case with, e.g., crude oil) whenever this is technically and economically advantageous. Biodiesel is a diesel-equivalent biofuel that is usually produced from 50 2 CHAPTER 2 12 principles of green chemistry vegetable oil and/or animal fat by re-esterification with methanol or ethanol (Scheme 2.11) and this material can be used in cars and other motors. CH2OCOR1 CH2OH CHOCOR2 + 3 CH3OH CHOH + 3 RCOOCH3 CH2OCOR3 CH2OH Glycerol Biodiesel Scheme 2.11: Reesterification of vegetable or animal fat for biodiesel production Interest in biodiesel as an alternative fuel has increased tremendously as a result of recent regulations requiring a substantial decrease in the hazardous emissions from motor vehicles, as well as the high crude oil prices. Biodiesels are biodegradable in water and are not toxic. Upon combustion, much less hazardous emissions are formed (less sulfur is emitted, 80% less carbohydrates and 50% less solid particles) as compared to petro-diesel. Biodiesel can be used in modern diesel motors without the need for alteration of the motor. With a flash point of 160 ºC, biodiesel is classified as a non-flammable liquid. This property makes it far safer in accidents involving motor vehicles when compared to petro-diesel and gasolines. Biodiesel production is, and will continue to be, related to a new revival in agriculture in some regions that are at present in decline. 2.8. Decrease and/or elimination of chemical stages Derivatizations, such as protection/deprotection and various other modifications, should be decreased or avoided wherever possible since these stages require additional amounts of reagents and waste products could be formed. Bromination at the para- or ortho-position of anilines without protection of the amino 51 2 CHAPTER 2 12 principles of green chemistry group (Scheme 2.12) is a process in which the protection/deprotection steps have been removed. Br X X NaBO3.4H2O,KBr NH2 NH2 AcOH N X = O, S N Scheme 2.12: Bromination of anilines without protection of the amino group 2.9. Catalysis It is well known that catalysts increase substantially the chemical process rates, without their consumption or insertion into the final products. It follows that, wherever possible, a catalyst should be used in a chemical process. The advantages of using catalysts include: - Higher yield; - Shorter reaction time; - The reaction proceeds in the presence of a catalyst but does not take place in its absence; - Increase in selectivity. An example of this approach is the polyurethane from 1,4-butanediol and hexamethelene diisocyanate in the presence of Maghnite as a catalyst (Algerian modified clay) (Scheme 2.13). CTAB-Mag O H2 H2 H2 H H2 HO C OH + O C N C N C O * C O C N C * 4 6 90 °C 4 6 6h n 1,4-Butanediol Hexamethelene diisocyanate Polyurethane Scheme 2.13: Synthesis of polyurethane by treated Maghnite as a catalyst 52 2 CHAPTER 2 12 principles of green chemistry 2.10. Design for degradation The design of the final chemical products should be such that, after fulfilling their functions, these products should easily degrade to harmless substances that do not cause environmental pollution. This approach is exemplified by the creation of biodegradable “green polymers”. Conventional polymers such as polyethylene and polypropylene persist for many years after disposal. Built for the long haul, these polymers seem inappropriate for applications in which plastics are used for short time periods prior to disposal. In contrast, biodegradable polymers (BPs) can be disposed in bioactive environments and degrade by the enzymatic action of microorganisms such as bacteria, fungi, and algae. The worldwide consumption of biodegradable polymers has increased from 14 million kg in 1996 to an estimated 68 million kg in 2001. Target markets for BPs include packaging materials (trash bags, wrappings, loose-fill foam, food containers, film wrapping, laminated paper), disposable nonwovens (engineered fabrics) and hygiene products (diaper back sheets, cotton swabs), consumer goods (fast-food tableware, containers, egg cartons, razor handles, toys), and agricultural tools (mulch films, planters). For example, polycaprolactone (PCL) and polyalkylenesuccinate are biodegradable polymers. PCL is a thermoplastic biodegradable polyester that is synthesized by chemical conversion of crude oil, followed by ring-opening polymerization. PCL has good water, oil, solvent, and chlorine resistance, has a low melting point and low viscosity, and is easily processed thermally. To reduce manufacturing costs, PCL may be blended with starch for example, to make trash bags. The blending PCL with fiber forming polymers (such as cellulose) has been used to produce hydro-entangled nonwovens (in which bonding of a fiber web into a sheet is accomplished by entangling the fibers using water jets), scrub-suits, incontinence products, and bandage holders. The rate of hydrolysis and biodegradation of PCL depends on its 53 2 CHAPTER 2 12 principles of green chemistry molecular weight and degree of crystallinity. However, many microbes in nature produce enzymes that are capable of complete PCL biodegradation (Scheme 2.14). O O * O * * * O 4 O O n n Polycaprolactone Polybutylenesuccinate Scheme 2.14: Chemical structure of polycaprolactone and polybutylenesuccinate 2.11. Real time analysis for pollution prevention Analytical methodologies should be developed in such a way that the process can be monitored in real time. New analytical tools are needed for real time monitoring of industrial process and to prevent the formation of toxic materials. The growing field of process analytical chemistry is aimed primarily at obtaining the analytical data close to the production operation. A real-time field measurement capability is desired for continuous environmental monitoring and this would replace the common approach of sample collection and transport to a central laboratory. The sensor in analytical chemistry will have a particularly important role to play, but in the future it is expected that traditional and instrumental analytical chemistry will also play a fundamental part in green technologies. The environmental and industrial interest in biosensor technology has been driven by the need for faster, simpler, cheaper, and better monitoring tools. Micro- fabricated microfluidic analytical devices, in which multiple sample-handling processes are integrated with the actual measurement step on a microchip platform, have been of considerable interest in recent times. For obvious reasons, such devices are referred to as “lab-on-a-chip” devices. In this respect complete assays, involving sample pretreatment (e.g., preconcentration/extraction), 54 2 CHAPTER 2 12 principles of green chemistry chemical/biochemical derivatization reactions, electrophoretic separations, and detection, have been carried out on single microchip platforms. The dramatic downscaling and integration of chemical assays make these analytical microsystems particularly attractive as “green analytical chemistry” screening tools and hold considerable promise for faster and simpler onsite monitoring of priority pollutants. A large number of environmental applications of capillary electrophoresis/electrochemistry (CE-EC) microchips have already appeared, including rapid separation and detection of chlorophenols, nitroaromatic explosives, hydrazines and organophosphate pesticides. 2.12. Inherently safer chemistry for accident prevention The reagents used to carry out chemical processes should be chosen with caution in order to avoid accidents, such as the release of poisonous substances into the atmosphere, explosions and fires. The oxidation of isatins to isatoic anhydrides has been achieved using a safe, cheap, stable and green oxidizing agent–urea/hydrogen peroxide complex and ultrasound irradiation at room temperature (Scheme 2.15). The oxidant is safer than liquid hydrogen peroxide. O O R R O H2NCONH2.H2O2 O R = H, F, Cl, Br, CH3, NO2 N N O H H Scheme 2.15: Oxidation of isatins with urea/hydrogen peroxide complex and ultrasound irradiation at room temperature 3. Green solvents The organic solvents used in numerous syntheses are quite hazardous to the environment. Volatile organic solvents are released into the environment by 55 2 CHAPTER 2 12 principles of green chemistry evaporation or flow in substantial amounts, since they are used in much higher proportions than the reagents themselves. A new approach to overcome this problem is to carry out the chemical reactions in the absence of such media, i.e., without solvents or by the use of non-volatile solvents that are harmless to humans and the environment. The ideal “green solvent” should have a high boiling point and it must be non-toxic, dissolve numerous organic compounds, cheap and, naturally, recyclable. Clearly such requirements strongly limit the choice of substance or class of compound as a green solvent. The substantial efforts of research groups throughout the world have led to the establishment of good alternatives to the common organic solvents, including: supercritical liquids, ionic liquids, low-melting polymers, perfluorinated (fluorous) solvents and water. 3.1. Water: Water is the basis and bearer of life. For millions of years, water has been at work to prepare the earth for the evolution of life. Water is the solvent in which numerous biochemical organic reactions (and inorganic reactions) take place. All of these reactions affect living systems and have inevitably occurred in an aqueous medium. On the other hand, modern organic chemistry has been developed almost on the basis that organic reactions often have to be carried out in organic solvents. It is only within the last two decades or so that people have again focused their attention on carrying out organic reactions in water. There are many potential advantages to use water in organic reactions as a green solvent: - Cost: Water is the cheapest solvent available on earth; using water as a solvent can make many chemical processes more economical. 56 2 CHAPTER 2 12 principles of green chemistry - Safety: Many organic solvents are flammable, potentially explosive, mutagenic, and/or carcinogenic. Water, on the other hand, has none of these adverse properties. - Synthetic efficiency: In many organic syntheses it may be possible to eliminate the need for the protection and deprotection of functional groups, thus saving numerous synthetic steps. Water soluble substrates can be used directly and this would be especially useful in carbohydrate and protein chemistry. - Environmental benefits: The use of water may alleviate the problem of pollution by organic solvents since water can be recycled readily and is benign when released into the environment (when harmful residues are not present). On the basis of the above characteristics water is probably the greenest solvent in view of its price, availability, safety and environmental effects. The drawbacks of using water, however, are that many organic compounds are insoluble or slightly soluble in water, and with some reagents (e.g., organometallic compounds) water is highly reactive. The use of water is often restricted to hydrolysis reactions, but in the early 1980s it was shown that water has unique properties that can lead to surprising results. The use of co-solvents or surfactants helps to increase the solubility of non-polar reagents by disrupting the dense hydrogen bonding network of pure water. The Wittig reaction has been investigated in aqueous conditions. Wittig olefination reactions with stabilized ylides (known as the Wittig–Horner or Horner– Wadsworth–Emmons reaction) are sometimes performed in an organic/aqueous biphasic system. In many cases a phase-transfer catalyst is used. Recently, the use of water alone as the solvent has been investigated and the reaction proceeded smoothly with a much weaker base such as K2CO3 or KHCO3. In addition, a phase- transfer catalyst was not required. Recently, water-soluble phosphonium salts were 57 2 CHAPTER 2 12 principles of green chemistry synthesized and their Wittig reactions with substituted benzaldehydes were carried out in aqueous sodium hydroxide solution (Scheme 2.16). R CHO A- aq, NaOH, r.t., 12 h P+ + R = H, OCH3, Cl, NO2 R HOOC Scheme 2.16: Witting reaction in aqueous media The assessment of how green a solvent is requires the consideration of various aspects, such as environmental impacts arising from industrial productions, recycling and disposal processes, as well as EHS (environmental, health and safety) characteristics. 26 organic solvents have been studied and results show that simple alcohols (methanol, ethanol) or alkanes (heptane, hexane) are environmentally preferable solvents, whereas the use of dioxane, acetonitrile, acids, formaldehyde and tetrahydrofuran is not recommendable from an environmental perspective. 3.2. Ionic liquids Ionic liquids are the most widely explored alternatives to organic solvents, as confirmed by the incredible number of publications in the literature dedicated to this topic. In our opinion, this is a new branch in applied organic chemistry and organic chemical technology. The great interest in these compounds is due to the fact that they possess some quite attractive properties, such as negligible vapour pressure, good chemical and thermal stability, they are inflammable, have high ionic conductivity, wide electrochemical potential and, in addition, they can act as catalysts. In contrast to conventional solvents that consist of single molecules, ionic liquids consist of ions and are liquid at room temperature or have a low melting 58 2 CHAPTER 2 12 principles of green chemistry temperatures (usually below 100 ºC). Due to their ionic nature, these materials reveal different properties when used as solvents in comparison to conventional molecular liquids. A huge variety of ionic liquids can be envisaged by simple combination of different cations and anions. By changing the anion or the alkyl chain of the cation, physical properties, such as hydrophobicity, viscosity, density and solvating ability can be varied. The application of ionic liquids (Scheme 2.17) is not limited only to the replacement of organic solvents in the reaction media of organic reactions. In some cases, ionic liquids can act as reagents, catalysts or media for catalyst immobilization or to induce chirality. R N X- + N+ N R R R: Alkyl X-: PF4, BF4, Br, OTs etc. Scheme 2.17: Chemical structure of some widely used ionic liquids The presence of Lewis acid species in chloroaluminate ionic liquids has also been used to bring about various acid-catalysed transformations that do not require additional catalysts. For example, acidic ionic liquids are ideally suited to Friedel– Crafts acylation reactions. In a traditional Friedel–Crafts acylation an acylium ion is generated by reaction between an acyl chloride and AlCl3 or FeCl3. Acidic chloroaluminate ionic liquids are able to generate acylium ions and are therefore ideally suited to Friedel–Crafts reactions. Acylation of mono-substituted aromatic compounds in acidic chloroaluminate ionic liquids leads almost exclusively to substitution at the 4 position on the ring (Scheme 2.18). 59 2 CHAPTER 2 12 principles of green chemistry X X CH3COCl 89-99% + N C 2H 5 H 3C N - Cl-AlCl3 H 3C O X: CH3, Cl, OCH3 Scheme 2.18: Alkylation of aromatic compounds in acidic chloroaluminate Essentially, there is no limit to the number of different ionic liquids that can be engineered with specific properties for chemical applications. However, a number of problems still need to be over-come before their use becomes widespread. The current problems associated with ionic liquids include: 1. Many are difficult to prepare in a pure form, and the current methods that provide pure ionic liquids are generally very expensive. Scale-up could be a problem in certain cases. 2. The viscosity of ionic liquids is often quite high. In addition, impurities can have a marked influence and may increase the viscosity of the ionic liquid. In the worst case scenario the addition of a catalyst and substrate to an ionic liquid can increase the viscosity to such an extent that it becomes gel-like and therefore difficult to process. 3. Some ionic liquids (e.g. chloroaluminates) are highly sensitive to oxygen and water, which means that they can only be used in an inert environment and all substrates must be dried and de-gassed before use. 4. Catalysts immobilized in ionic liquids are sometimes leached into the product phase. It may therefore be necessary to design new catalysts for use in ionic liquids. 60 2 CHAPTER 2 12 principles of green chemistry Despite these problems, ionic liquids are currently attracting considerable attention as alternatives to volatile organic solvents in many different reactions, including oligomerization and polymerization, hydrogenation, hydroformylation and oxidation, C–C coupling and metathesis. In particular, ionic liquids containing BF41 or PF61 anions have been very widely used and several general proper ties have emerged: 1. These ionic liquids form separate phases with many organic materials and can therefore be used in biphasic catalysis. 2. These liquids are non-nucleophilic and present an inert environment that often increases the lifetime of the catalyst. 3. The rate of diffusion of gases is very high compared to many conventional solvents and this leads to increased reaction rates in catalysed reactions involving gaseous substrates such as hydrogenation, hydroformylation and oxidation. 3.3. Poly(ethylene glycol) Poly(ethylene glycol) (PEG) is a linear polymer obtained by polymerization of ethylene oxide. The term PEG is used to designate a polyether with a molecular mass lower than 20000. It is known that PEG is a cheap, thermally stable, biocompatible, non-toxic material that can be recycled. In addition, PEG and its monomethyl ethers have low vapor pressures, are inflammable and can be separated from the reaction medium by a simple procedure. For this reason, it is believed that PEG is a green alternative to volatile organic solvents and is a very convenient medium for organic reactions. PEG is used as an effective medium for phase transfer catalysis and, in some cases, as a polyether catalyst in the phase transfer catalysis reaction. Recently, PEG was used as a reaction medium for organic reactions; low molecular weight (< 20000) derivatives are usually applied since they have low 61 2 CHAPTER 2 12 principles of green chemistry melting points or are liquids at room temperature. Despite the fact that PEGs are less widely used, they are commercial products and are much cheaper than ionic liquids but, unlike the latter, their properties cannot be changed easily. One of the greatest disadvantages of PEGs (which also holds for ionic liquids) is that organic solvents must be used for the extraction of the reaction products – although supercritical carbon dioxide (scCO2) could also be used in both cases. The literature examples of the use of PEG are scarce but, in recent years, polyethylene glycol-promoted reactions have attracted the attention of organic chemists due to the solvating power of these compounds and their ability to act as a phase transfer catalyst, negligible vapor pressure, easy recyclability, reusability, ease of work-up, eco-friendly nature, and low cost. An efficient and facile method for the synthesis of 3-amino 1H-pyrazoles in the presence of p-toluenesulfonic acid using PEG-400 as an efficient and reusable reaction medium has been reported (Scheme 2.19). This method does not require expensive reagents or special care to exclude the moisture from the reaction medium. H3C CH3 O CH3 CN PEG 400, 80 °C, 1 h, p-TsOH H3C + H2NNH2.H2O 98% N H2N N H3C CH3 H Scheme 2.19: Synthesis of 3-amino 1H-pyrazoles using PEG-400 as an efficient and reusable reaction medium. 3.4. Perfuorinated (Fluorous) solvents The term “fluorous” was introduced for the first time by Horvath and Rabai by analogy with “aqueous” or “aqueous medium”. Fluorous compounds have recently been defined as substances that are fluorinated to a high degree and are based on 62 2 CHAPTER 2 12 principles of green chemistry sp3-hybridised carbon atoms. Perfluorous solvents, such as perfluoroalkanes, perfluoroalkyl ethers and per-fluoroalkylamines, are chemically stable and are harmless to the environment since they are non-toxic (unlike the freons), inflammable, thermally stable and could be recycled. These compounds have a high ability to dissolve oxygen, which is an advantage used in medical technology. In fluorous solvents or liquids, the fluorine atoms are substituents on the carbon atoms (C–F bond). Fluorous liquids have quite unusual properties and these include high density, high stability (mainly due to the stability of the C–F bond), low dissolving ability and extremely low solubility in water and organic solvents, although they are miscible with the latter at higher temperatures. The low solubility of the perfluorinated solvents can be explained in terms of their low surface tension, the weak intermolecular interactions, high densities and low dielectric constants. The reactions that take place in perfluorous solvents show a somewhat different trend in comparison to the other alternative green solvents. Although they are solvents, they cannot be considered as substitutes for solvents. Due to the fact that they are extremely non-polar, they are inappropriate to perform most chemical reactions and are used together with conventional organic solvents to give biphasic mixtures. In such a biphasic mixture, the soluble reagent or catalyst is in the fluorous phase while the starting materials are dissolved in the immiscible solvent phase, which could be an organic solvent, water or non-organic solvent. These two distinct layers are homogenized upon heating, the reactants come into contact with one another and the reaction takes place. The layers separate again upon cooling, with the reaction products remaining in the organic phase while the unreacted substances and the catalyst remain in the perfluorous phase. This situation allows an easy 63 2 CHAPTER 2 12 principles of green chemistry separation of the reaction products and catalyst recycling without the use of an organic solvent for extraction. Such a system combines the advantages of a monopha-sic system with the ease of product separation in the biphasic system; this system is non-toxic, can be used several times, and allows the easy separation of the catalyst from the reagents and products (Figure 2.1). Reactants Warm Warm Cool Products Organic Solvent Fluorous solvent (Reactants) Products Rfr-catalyst-Rfr Rfr-catalyst-Rfr Lower temperature Higher temperature Lower temperature (Biphasic) (Monophasic) (Biphasic) Figure 2.1: Schematic representation of organic syntheses in fluorous solvents One example of this approach is the Sonogashira coupling in a liquid/liquid fluorous biphasic system for the preparation of 1-(4-nitrophenyl)-2-phenylacetylene (Scheme 2.20). In some cases the reaction takes place very rapidly at lower temperatures in a two-phase system. A disadvantage of the fluorous solvents is that they are expensive and toxic gaseous fluorine or hydrogen fluoride is required for their production. NO2 C8F17 H P PdCl2 3 2 + NO2 FC-72, Copper(I) Iiodide, n-Bu2NH, DMF, 55 °C, 4 h Br FC-72: is a mixure of perfluorohexane and is commercial product Scheme 2.20: Sonogashira coupling in a liquid/liquid fluorous biphasic system 64 2 CHAPTER 2 12 principles of green chemistry 3.5. Supercritical liquids A supercritical liquid (SCL) is defined as a substance above its critical temperature (Tc) and critical pressure (Pc). The properties of an SCL are between those of its liquid and gaseous phases. These properties can be specifically changed by varying the temperature and pressure. The most widely used SCL is carbon dioxide (scCO2). The critical point of CO2 is at 73 atm and 31.1 ºC; conditions that can easily be achieved in the laboratory. Other supercritical solvents are not as useful because of the extreme conditions required to achieve the critical point. For instance, the critical point of water is at 218 atm and 374 ºC. Recently, examples have appeared in the literature of reactions in scH2O. The advantages of using scCO2 are as follows: CO2 is inflammable and is less toxic than most organic solvents, it is relatively inert toward reactive substances, it is a natural gas found in the atmosphere and there are no regulations concerning its use, it can be easily removed by decreasing the pressure, which provides the possibility of its easy removal from the reaction products, it has a high gas-dissolving ability, a low solvating ability, a high diffusion rate and good mass transfer properties. The selectivity of a reaction can be dramatically changed when conducted in sc liquids when compared to the use of traditional organic solvents. In 1992 it was demonstrated that scCO2 works as an alternative solvent to chlorofluorocarbons (CFCs) for the homogeneous free-radical polymerization of highly fluorinated monomers. The homogeneous polymerization of 1,1- dihydroperfluorooctyl acrylate using azobisisobutyronitrile (AIBN) in scCO2 (59.4 ºC, 207 bar) gave perfluoropolymer in 65% yield with a molecular weight of 270000 (Scheme 2.21). 65 2 CHAPTER 2 12 principles of green chemistry * AIBN * O C7F15 n ScCO2 (207 bar) O 59.4 °C, 48 h, 65% O O C7F15 Scheme 2.21: Polymerization of an acrylate perfluoromonomer to perfluoropolymer in scCO2 In 1994, the first example of free-radical dispersion polymerization using amphiphilic polymers as stabilizers in scCO2 was reported. ScCO2 has also been successfully employed in cationic polymerizations. One example is the polymerization of isobutyl vinyl ether (IBVE) using an adduct of acetic acid and IBVE as the initiator, ethylaluminum dichloride as a Lewis acid and ethyl acetate as a Lewis base deactivator. The reaction proceeded via a heterogeneous precipitation process in scCO2 (40 °C, 345 bar) to form poly(IBVE) in 91% yield with a molecular weight distribution of 1.8 (Scheme 2.22). Several reviews have been published covering the history and recent developments of homogeneous and heterogeneous polymerizations in scCO2. OAc OBu-i EtAlCl2, AcOEt O ScCO2 (345 bar) O 40 °C, 12 h, 91% n Scheme 2.22: Dispersion polymerization in scCO2 Stoichiometric and catalytic Diels–Alder reactions in scCO2 have been studied extensively and the first report appeared in 1987. The reaction of maleic anhydride 66 2 CHAPTER 2 12 principles of green chemistry and isoprene was conducted in scCO2 and the effect of CO2 pressure (80–430 bar) on the reaction rate was investigated (Scheme 2.23). O O 35-60 °C + O ScCO2 (80-430 bar) O O 95% Scheme 2.23: Diels–Alder reaction in scCO2 The disadvantages of supercritical fluids should also be mentioned and these include the following: reactivity towards strong nucleophiles, specialized and expensive equipment is required to achieve the critical conditions, low dielectric constant and hence low dissolving ability, and the fluid behaves as a hydrocarbon solvent, for this reason it dissolves catalysts and/or reagents with some difficulty. 67 2 CHAPTER 2 12 principles of green chemistry Second tutorial file Questions and problems 1. Which of the following is a greener route to produce ethanal commercially? a) Catalytic cracking of ethanol; b) Oxidation of ethene with an ionic catalyst; c) Steam reforming of methanol; d) Dehydrogenation of ethylene. 2. Name the conventional solvent that was used for dry cleaning purposes which later confirmed to be a suspected carcinogen. a) Supercritical CO2; b) Phenanthrene; c) Tetrachloroethene; d) Benzene aldehyde. 3. Dr. Paul Anastas & Dr. John Warner created 10 Principles of Green Chemistry to reduce or eliminate the use and generation of hazardous substances? a) True; b) False. 4. Which of the following is not a principle of Green Chemistry? a) Green solvents and auxiliaries; b) Use of renewable feedstock; c) Hazardous chemical synthesis; d) Design for energy efficiency. 5. Green chemistry is the design of chemical products and processes that reduce or eliminate the use and generation of hazardous substances. Identify the technique used in this area. a) Bioamplification; 68 2 CHAPTER 2 12 principles of green chemistry b) Polymer manufacturing; c) Pesticide synthesis; d) Use of sunlight and Microwave-assisted reaction. 6. Which of the following gas was traditionally used to bleach paper? a) Sulfur ; b) Carbon dioxide ; c) Chlorine ; d) Fluorine. 7. Replacement of liquid CO2 by halogenated solvent will result in less harm to groundwater. a) True; b) False. 8. Which of the following is a green solvent used for bleaching clothes? a) Hydrogen peroxide; b) Tetrachloroethene; c) Benzene; d) Toluene. 9. Identify the non-toxic and green solvent. a) Liquified carbondioxide; b) Benzene; c) Carbon tetrachloride; d) Toluene. 10. Which of the following are among the 12 Principles of Green Chemistry? a) Design commercially viable products; b) Use only new solvents; c) Use catalysts, not stoichiometric; reagents; 69 2 CHAPTER 2 12 principles of green chemistry d) Re-use waste. 11. Green chemistry can reduce all but which of the following? a) Cost; b) Risk & Hazard; c) Awareness; d) Waste. 12. Green chemistry synthesis could also involve which of the following? a) High temperature; b) Dichloromethane; c) Fossil fuels; d) Microwave. 13. Biodiesel is an example of which of the 12 Principles of Green Chemistry? a) Waste prevention; b) Use of renewable feedstocks; c) Use of catalysis; d) Safer solvents. 14. The use of solar power is covered within Green Chemistry Principle which is? a) Atom economy; b) Design for energy efficiency; c) Design benign chemicals; d) Less hazardous synthesis. 15. Bio-polymers exemplify green chemistry which is? a) Catalysis; b) Prevent waste; c) Benign solvents & auxiliaries; d) Design for degradation. 70 2 CHAPTER 2 12 principles of green chemistry 16........................was a co-founder of the worldwide green chemistry movement and the first director of the Green Chemistry Institute, now part of ACS? a) Joseph Breen; b) Albert Einstein; c) John Warner; d) Paul Anastas. 17. Green chemistry can provide green technology solutions for a sustainable future? a) True; b) False. 18. The following is often referred to as the universal solvent and is a preferred green solvent? a) Water; b) Methanol; c) Ethyl Acetate; d) Benzene. 19. This ‘green’ chemical is used in household cleaners to remove stains and is also a favorite dressing on salads!? a) Vinegar (acetic acid); b) Citric acid; c) Hydrochloric acid (HCl); d) Water; 20. The following legislation gave birth to today's green chemistry initiatives? a) Clean Water Act of 1972; b) Montreal Protocol of 1989; c) Pollution Prevention Act of 1991; d) Superfund Act of 1980. 71 2 CHAPTER 2 12 principles of green chemistry 21. Lignin, switch grass, and cellulose are all types of UUUUUUU.? a) Enzymes; b) Catalysts; c) Bio-based feedstock’s; d) Anti-cancer compounds. 22. UUUUUUUUUU or VOCs, have been replaced and were banned in some paints? a) Versatile Organic Chemicals; b) Volatile Organic Compounds; c) Volatile Organic Components; d) Versatile Odorless Components. 23.................................. is fulfilling the needs of the present generation without compromising the ability of future generations to meet their needs? a) Sustainability; b) Green chemistry; c) Life Cycle Assessment; d) Recycling. 24. Shortly after mid-night in 1984, a reaction caused poisonous methyl isocyanate gas to leak from a factory in this city, UUUUU..causing 3,700 deaths? a) Bhopal; b) Hinkley; c) Calcutta; d) Siberia. 25. Benzene, a..........................substance, is an important industrial solvent used in the production of pharmaceuticals, plastics, and dyes? a) Odorless; 72 2 CHAPTER 2 12 principles of green chemistry b) Non-flammable; c) Biodegradable; d) Carcinogenic. 26. Environmental benefits of green chemistry include: a) Fewer raw materials and natural resources used; b) Cleaner production technologies & reduced emissions; c) Smaller quantities of hazardous waste to be treated and disposed of; d) All of the above. 27. Used to indicate the level of contaminants present, the term ‘PPM’ means? a) Parts-per-micron; b) Parts-per-million; c) Parts-per-mass; d) Parts-per-molecule. 28. The term which refers to the breakup within a compound due to microbial activity is? a) Microbial degradation; b) Agro-degradation; c) Photo-degradation; d) Decomposition. 29. The first listed of the 12 Principles of Green Chemistry is? a) Prevent waste; b) Catalysis; c) Atom economy; d) Benign solvents. 30. Business benefits of green chemistry include: a) Reduced costs associated with waste treatment and disposal; 73 2 CHAPTER 2 12 principles of green chemistry b) Innovating 'greener' products to entice customers; c) Greater compliance with environmental legislation; d) All of the above. 74