Chapter 12a Muscle Physiology(1).pdf
Document Details
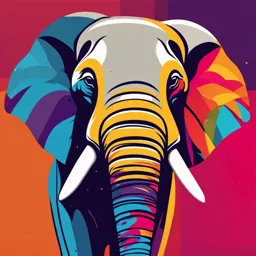
Uploaded by AmazedElectricOrgan
2017
Tags
Full Transcript
PowerPoint® Lecture Presentation CHAPTER 12a Muscle Physiology © 2017 Pearson Education, Inc. Chapter Outline 12.1 12.2 12.3 12.4 12.5 12.6 Skeletal Muscle Structure The Mechanism of Force Generation in Muscle The Mechanics of Skeletal Muscle Contraction Skeletal Muscle Metabolism Control of Skeleta...
PowerPoint® Lecture Presentation CHAPTER 12a Muscle Physiology © 2017 Pearson Education, Inc. Chapter Outline 12.1 12.2 12.3 12.4 12.5 12.6 Skeletal Muscle Structure The Mechanism of Force Generation in Muscle The Mechanics of Skeletal Muscle Contraction Skeletal Muscle Metabolism Control of Skeletal Muscle Activity Smooth and Cardiac Muscle © 2017 Pearson Education, Inc. 12.1 Skeletal Muscle Structure Skeletal muscles Connected to two or more bones by tendons The tissue surrounding muscle (epimysium) and tendon connective tissue are continuous Perimysium extends into the muscle body, dividing muscle into bundles (fascicles) of muscle cells Fascicles contain 100s-1000s of muscle cells—muscle fibers, which extend the length of the muscle © 2017 Pearson Education, Inc. Structure at the Cellular Level Components of a muscle fiber Muscle fibers surrounded by connective tissue (endomysium) Sarcolemma Plasma membrane Multinucleated Sarcoplasm Cytoplasm © 2017 Pearson Education, Inc. Figure 12.1 Structure of a skeletal muscle. Bone Tendon Muscle body Perimysium Epimysium Fascicle Muscle fiber (cell) Myofibril Endomysium Muscle fibers in fascicle © 2017 Pearson Education, Inc. Nucleus Sarcolemma Protein filaments Structure at the Cellular Level Components of a muscle fiber Contains many myofibrils Sarcoplasmic reticulum Smooth ER Contains many mitochondria—high energy Transverse tubules T tubules Lateral sacs (terminal cisternae) Ca2+ Triad: T tubule + two lateral sacs © 2017 Pearson Education, Inc. Figure 12.2 Structure of a skeletal muscle fiber. Axon of motor neuron Neuromuscular junction Axon terminal Muscle fiber Synaptic vesicles Synaptic cleft Motor end plate T tubule Nucleus Sarcolemma Sarcoplasmic reticulum Sarcoplasmic reticulum T tubule Myofibrils Triad T tubule Lateral sacs Lateral sacs Mitochondria © 2017 Pearson Education, Inc. Thick filament (myosin) Thin filament (actin) Structure at the Molecular Level Myofibrils Give skeletal and cardiac muscle striated appearance Orderly arrangement of thick and thin filaments Actin Myosin Striations due to thick and thin filaments that run parallel to the long axis Filaments form sarcomeres © 2017 Pearson Education, Inc. Structure at the Molecular Level Structure of a sarcomere A band I band Dark band Thick filaments H zone Thick filaments No overlap M line Links thick filaments © 2017 Pearson Education, Inc. Light band Thin filament No overlapping Z line Links thin filaments Sarcomere Functional unit Z line to Z line Figure 12.3 Sarcomere structure. M line I band Crossbridges H zone Z line Thick filaments Myofibril Z line A band Sarcomere Z line M line M line Z line H zone I band A band © 2017 Pearson Education, Inc. Z line Thin filaments Cross section of A band where thick and thin filaments overlap Figure 12.4 Structure of a thin filament. Myosin-binding site G actin G actin molecules F actin Double-helical actin strands Actin Tropomyosin Troponin complex Portion of thin filament © 2017 Pearson Education, Inc. Structure at the Molecular Level Actin Contractile protein Each G actin has a binding site for myosin Tropomyosin Regulatory protein Overlaps binding sites on actin for myosin © 2017 Pearson Education, Inc. Structure at the Molecular Level Troponin Regulatory protein Complex of three proteins Attaches to actin Attaches to tropomyosin Binds Ca2+ reversibly Ca2+ binding to troponin regulates skeletal muscle contraction © 2017 Pearson Education, Inc. Figure 12.5a-c Structure of a thick filament. Heads Tail Actinbinding site ATPase site Myosin molecule Two myosin molecules bound at their tail ends Crossbridges Portion of thick filament © 2017 Pearson Education, Inc. Bare zone Figure 12.5d-e Structure of a thick filament. Sarcomere Titin Thick filament (myosin) Thin filament (actin) Crossbridge Z line Sarcomere Thin filament Thick filament Crossbridge Electron micrograph of portion of sarcomere © 2017 Pearson Education, Inc. Structure at the Molecular Level Thick myofilament Myosin tail is toward the M line Myosin head is toward the I band Myosin head binding sites Actin binding site Nucleotide binding site for ATP and ATPase © 2017 Pearson Education, Inc. Structure at the Molecular Level Titin Is a very elastic protein Supports protein in muscle Anchors thick filaments between the M line and the Z line Provides structural support and elasticity © 2017 Pearson Education, Inc. 12.2 The Mechanism of Force Generation in Muscle The sliding filament model The crossbridge cycle: how muscles generate force Excitation-contraction coupling: how muscle contractions are turned on and off Muscle cell metabolism: how muscle cells provide ATP to drive the crossbridge cycle © 2017 Pearson Education, Inc. Sliding-Filament Model Muscle contraction Shortening of muscle Thick and thin filaments overlap Neither thick nor thin filaments shorten Filaments slide past each other © 2017 Pearson Education, Inc. Sliding-Filament Model Within a sarcomere during contraction A band stays the same length I band shortens H zone shortens Sarcomere shortens Sliding is due to cyclical formation and breaking of cross bridges = crossbridge cycle © 2017 Pearson Education, Inc. Figure 12.6a How changes in striation pattern are explained by the sliding-filament model of muscle contraction. H zone I band I band shortens H zone shortens Z line I band shortens A band remains the same © 2017 Pearson Education, Inc. Figure 12.6b How changes in striation pattern are explained by the sliding-filament model of muscle contraction. Sarcromere (muscle relaxed) I band H zone I band A band Sarcromere (muscle contracted) © 2017 Pearson Education, Inc. The Crossbridge Cycle: How Muscles Generate Force Cyclical formation of links between actin and myosin, resulting in the sliding of thin filaments toward the M line of a sarcomere Myosin head undergoes conformation changes, swiveling back and forth High-energy form ADP and Pi bound to myosin High affinity for actin Low-energy form ATP bound to myosin Low affinity for actin Relies on hydrolysis of ATP © 2017 Pearson Education, Inc. The Crossbridge Cycle: How Muscles Generate Force Analogous to rowing a boat through water Oar paddle = crossbridge Linking of thick filament to thin filament = oar contact with water © 2017 Pearson Education, Inc. The Crossbridge Cycle: How Muscles Generate Force Power stroke: myosin head moves, propelling thin filament toward center of muscle = movement of oar propelling boat Thick and thin filaments detach = oar breaks contact with water Myosin head returns to the initial position = oar moved to new position, cycle starts again © 2017 Pearson Education, Inc. The Crossbridge Cycle © 2017 Pearson Education, Inc. Figure 12.7 The crossbridge cycle. Slide 1 ATP is hydrolyzed ATP Pi ADP Cocking of the myosin head (myosin in high-energy form) Unbinding of myosin and actin New ATP binds to myosin head ATP Pi ADP Binding of myosin to actin Rigor (myosin in low-energy form) ADP is released Power stroke Inorganic phosphate is released ADP Actin gets pulled toward middle of sarcomere © 2017 Pearson Education, Inc. ADP Pi Figure 12.7 The crossbridge cycle. Slide 2 Pi ADP Binding of myosin to actin © 2017 Pearson Education, Inc. Figure 12.7 The crossbridge cycle. Slide 3 Pi ADP Binding of myosin to actin Inorganic phosphate is released Pi © 2017 Pearson Education, Inc. Figure 12.7 The crossbridge cycle. Slide 4 Pi ADP Binding of myosin to actin Power stroke Actin gets pulled toward middle of sarcomere © 2017 Pearson Education, Inc. ADP Inorganic phosphate is released Pi Figure 12.7 The crossbridge cycle. Slide 5 Pi ADP Binding of myosin to actin ADP is released Power stroke Inorganic phosphate is released ADP Actin gets pulled toward middle of sarcomere © 2017 Pearson Education, Inc. ADP Pi Figure 12.7 The crossbridge cycle. Slide 6 Pi ADP Binding of myosin to actin Rigor (myosin in low-energy form) ADP is released Power stroke Inorganic phosphate is released ADP Actin gets pulled toward middle of sarcomere © 2017 Pearson Education, Inc. ADP Pi Figure 12.7 The crossbridge cycle. Slide 7 New ATP binds to myosin head ATP Pi ADP Binding of myosin to actin Rigor (myosin in low-energy form) ADP is released Power stroke Inorganic phosphate is released ADP Actin gets pulled toward middle of sarcomere © 2017 Pearson Education, Inc. ADP Pi Figure 12.7 The crossbridge cycle. Slide 8 ATP Unbinding of myosin and actin New ATP binds to myosin head ATP Pi ADP Binding of myosin to actin Rigor (myosin in low-energy form) ADP is released Power stroke Inorganic phosphate is released ADP Actin gets pulled toward middle of sarcomere © 2017 Pearson Education, Inc. ADP Pi Figure 12.7 The crossbridge cycle. Slide 9 ATP is hydrolyzed ATP Unbinding of myosin and actin New ATP binds to myosin head ATP Pi ADP Binding of myosin to actin Rigor (myosin in low-energy form) ADP is released Power stroke Inorganic phosphate is released ADP Actin gets pulled toward middle of sarcomere © 2017 Pearson Education, Inc. ADP Pi Figure 12.7 The crossbridge cycle. Slide 10 ATP is hydrolyzed ATP Pi ADP Cocking of the myosin head (myosin in high-energy form) Unbinding of myosin and actin New ATP binds to myosin head ATP Pi ADP Binding of myosin to actin Rigor (myosin in low-energy form) ADP is released Power stroke Inorganic phosphate is released ADP Actin gets pulled toward middle of sarcomere © 2017 Pearson Education, Inc. ADP Pi Excitation-Contraction Coupling: How Muscle Contractions Are Turned On and Off Sequence of events whereby an action potential in the sarcolemma causes contraction Dependent on neural input from the motor neuron Requires Ca2+ release from the sarcoplasmic reticulum © 2017 Pearson Education, Inc. Excitation-Contraction Coupling: How Muscle Contractions Are Turned On and Off Role of the neuromuscular junction Each motor neuron innervates several muscle cells Each muscle fiber receives input from a single motor neuron Similar to ordinary synapse Acetylcholine released Motor end plate High density of acetylcholine receptors Highly folded End-plate potential Motor neuron AP always creates a muscle cell AP © 2017 Pearson Education, Inc. Excitation-Contraction Coupling: How Muscle Contractions Are Turned On and Off Roles of Ca2+, troponin, and tropomyosin If no Ca2+ → troponin holds tropomyosin over myosin binding sites on actin No crossbridges form between actin and myosin Muscle relaxed If Ca2+ present → binds to troponin, causing movement of troponin, causing movement of tropomyosin, exposing binding sites for myosin on actin Crossbridges form between actin and myosin Cycle occurs; muscle contracts © 2017 Pearson Education, Inc. Excitation-Contraction Coupling: How Muscle Contractions Are Turned On and Off Steps of excitation-contraction coupling 1. Action potential in sarcolemma 2. Action potential down T tubules 3. DHP receptors of T tubules open Ca2+ channels (ryanodine receptors) in lateral sacs of SR 4. Ca2+ increases in cytosol 5. Ca2+ binds to troponin, shifting tropomyosin 6. Crossbridge cycling occurs © 2017 Pearson Education, Inc. Figure 12.8 Events in excitation-contraction coupling. Slide 1 Acetylcholine (ACh) is released from the axon terminal of a motor neuron and binds to receptors in the motor end plate. This binding elicits an end-plate potential, which triggers an action potential in the muscle cell. Axon terminal Neuromuscular junction Synaptic cleft Sarcolemma ACh Action potential propagates along the sarcolemma and down T tubules Motor end plate Ca2+ is actively transported back into lumen of SR following the action potential T tubule Sarcoplasmic reticulum (SR) ACh receptors Ca2+ Ca2+ Tropomyosin blocks myosin-binding sites (muscle fiber relaxes) The action potential triggers Ca2+ release from SR Ca2+ Ca2+ binds to troponin, exposing myosin-binding sites Crossbridge cycle begins (muscle fiber contracts) © 2017 Pearson Education, Inc. Figure 12.8 Events in excitation-contraction coupling. Acetylcholine (ACh) is released from the axon terminal of a motor neuron and binds to receptors in the motor end plate. This binding elicits an end-plate potential, which triggers an action potential in the muscle cell. Axon terminal Neuromuscular junction Synaptic cleft ACh Motor end plate ACh receptors © 2017 Pearson Education, Inc. Slide 2 Figure 12.8 Events in excitation-contraction coupling. Slide 3 Acetylcholine (ACh) is released from the axon terminal of a motor neuron and binds to receptors in the motor end plate. This binding elicits an end-plate potential, which triggers an action potential in the muscle cell. Axon terminal Neuromuscular junction Synaptic cleft Sarcolemma ACh Action potential propagates along the sarcolemma and down T tubules Motor end plate ACh receptors © 2017 Pearson Education, Inc. T tubule Sarcoplasmic reticulum (SR) Figure 12.8 Events in excitation-contraction coupling. Slide 4 Acetylcholine (ACh) is released from the axon terminal of a motor neuron and binds to receptors in the motor end plate. This binding elicits an end-plate potential, which triggers an action potential in the muscle cell. Axon terminal Neuromuscular junction Synaptic cleft Sarcolemma ACh Action potential propagates along the sarcolemma and down T tubules Motor end plate T tubule Sarcoplasmic reticulum (SR) ACh receptors Ca2+ The action potential triggers Ca2+ release from SR Ca2+ © 2017 Pearson Education, Inc. Figure 12.8 Events in excitation-contraction coupling. Slide 5 Acetylcholine (ACh) is released from the axon terminal of a motor neuron and binds to receptors in the motor end plate. This binding elicits an end-plate potential, which triggers an action potential in the muscle cell. Axon terminal Neuromuscular junction Synaptic cleft Sarcolemma ACh Action potential propagates along the sarcolemma and down T tubules Motor end plate T tubule Sarcoplasmic reticulum (SR) ACh receptors Ca2+ The action potential triggers Ca2+ release from SR Ca2+ Ca2+ binds to troponin, exposing myosin-binding sites © 2017 Pearson Education, Inc. Figure 12.8 Events in excitation-contraction coupling. Slide 6 Acetylcholine (ACh) is released from the axon terminal of a motor neuron and binds to receptors in the motor end plate. This binding elicits an end-plate potential, which triggers an action potential in the muscle cell. Axon terminal Neuromuscular junction Synaptic cleft Sarcolemma ACh Action potential propagates along the sarcolemma and down T tubules Motor end plate T tubule Sarcoplasmic reticulum (SR) ACh receptors Ca2+ The action potential triggers Ca2+ release from SR Ca2+ Ca2+ binds to troponin, exposing myosin-binding sites Crossbridge cycle begins (muscle fiber contracts) © 2017 Pearson Education, Inc. Figure 12.8 Events in excitation-contraction coupling. Slide 7 Acetylcholine (ACh) is released from the axon terminal of a motor neuron and binds to receptors in the motor end plate. This binding elicits an end-plate potential, which triggers an action potential in the muscle cell. Axon terminal Neuromuscular junction Synaptic cleft Sarcolemma ACh Action potential propagates along the sarcolemma and down T tubules Motor end plate Ca2+ is actively transported back into lumen of SR following the action potential T tubule Sarcoplasmic reticulum (SR) ACh receptors Ca2+ Ca2+ The action potential triggers Ca2+ release from SR Ca2+ Ca2+ binds to troponin, exposing myosin-binding sites Crossbridge cycle begins (muscle fiber contracts) © 2017 Pearson Education, Inc. Figure 12.8 Events in excitation-contraction coupling. Slide 8 Acetylcholine (ACh) is released from the axon terminal of a motor neuron and binds to receptors in the motor end plate. This binding elicits an end-plate potential, which triggers an action potential in the muscle cell. Axon terminal Neuromuscular junction Synaptic cleft Sarcolemma ACh Action potential propagates along the sarcolemma and down T tubules Motor end plate Ca2+ is actively transported back into lumen of SR following the action potential T tubule Sarcoplasmic reticulum (SR) ACh receptors Ca2+ Ca2+ Tropomyosin blocks myosin-binding sites (muscle fiber relaxes) The action potential triggers Ca2+ release from SR Ca2+ Ca2+ binds to troponin, exposing myosin-binding sites Crossbridge cycle begins (muscle fiber contracts) © 2017 Pearson Education, Inc. Figure 12.9 Actions of troponin and tropomyosin in excitation-contraction coupling. Actin Tropomyosin Troponin complex Relaxed Contracting © 2017 Pearson Education, Inc. Ca2+ bound to troponin Myosin-binding site exposed Excitation-Contraction Coupling © 2017 Pearson Education, Inc. Excitation-Contraction Coupling: How Muscle Contractions Are Turned On and Off Gating of sarcoplasmic reticulum Ca2+ channels Voltage-gated opening Coupled to T tubules by ryanodine and DHP receptors Ca2+-induced opening Ca2+-induced closing © 2017 Pearson Education, Inc. Excitation-Contraction Coupling: How Muscle Contractions Are Turned On and Off Termination of contraction Ca2+ must leave troponin, allowing tropomyosin to cover myosin binding sites on actin To remove Ca2+ from cytosol Ca2+-ATPase in the sarcoplasmic reticulum Transports Ca2+ from cytosol into the sarcoplasmic reticulum © 2017 Pearson Education, Inc. 12.3 The Mechanics of Skeletal Muscle Contraction The twitch Factors affecting the force generated by individual muscle fibers Regulation of the force generated by the whole muscle Velocity of shortening © 2017 Pearson Education, Inc. The Twitch Contraction produced in a muscle fiber in response to a single action potential An all-or-nothing event for a muscle fiber at rest Can be defined for a muscle fiber, for a motor unit, or at the whole muscle level © 2017 Pearson Education, Inc. The Twitch Phases of a twitch Latent period Time from action potential in muscle cell to onset of contraction (few milliseconds) Excitation-contraction coupling Contraction phase Time tension is increasing (10–100 msec) Crossbridge cycling Relaxation phase Time tension is decreasing back to zero (longer than contraction phase) Ca2+ reuptake © 2017 Pearson Education, Inc. Tension (force of contraction) (g) Figure 12.11 Phases of a twitch. Latent period Stimulus © 2017 Pearson Education, Inc. Contraction phase Relaxation phase Time (msec) The Twitch Isometric and isotonic twitches Contractile elements = sarcomeres Series elastic elements = connective tissue, tendons Force exerted by contracting muscle = tension Force opposing contraction (such as weight to be moved) = load © 2017 Pearson Education, Inc. The Twitch Isometric twitch contraction Length constant Contractile elements contract, generating tension When load > tension Stretches series of elastic elements Muscle does not shorten, load not lifted © 2017 Pearson Education, Inc. Figure 12.12a Isometric and isotonic muscle contractions. Contracted muscle Tension (g) Relaxed muscle Rigid support Time (msec) Force transducer Stimulator Isometric muscle contraction © 2017 Pearson Education, Inc. Strip chart recorder The Twitch Isotonic twitch contraction Constant tension When tension > load Load is lifted as muscle shortens © 2017 Pearson Education, Inc. Contracted muscle Length transducer Movable lever Load Load Time (msec) Force transducer Stimulator Isotonic muscle contraction © 2017 Pearson Education, Inc. Tension (g) Relaxed muscle Degree of shortening (mm) Figure 12.12b Isometric and isotonic muscle contractions. Strip chart recorder Figure 12.13 Effect of load on peak tension in an isotonic twitch. Isotonic contraction Isotonic contraction Isotonic contraction Isometric contraction 10 g 15 g 20 g Muscle Load 5g 20 Isometric contraction Latent period to start of shortening Tension (g) 15 10 Shortening begins Ends 5 0 Stimuli Time (msec) © 2017 Pearson Education, Inc. 17 grams The Twitch Normal muscle activity Some purely isometric contractions occur Purely isotonic contractions are rare Even if the load is constant, isometric precedes isotonic phase of contraction Isometric continues (tension increases) until tension exceeds load Then isotonic contraction begins Tension remains constant as muscle shortens Load generally not constant Load changes as limb position changes © 2017 Pearson Education, Inc. Factors Affecting the Force Generated by Individual Muscle Fibers Graded muscle contractions depend on two factors: Tension produced by each fiber Number of active crossbridges that bind to actin More crossbridges that bind → more force Number of fibers contracting © 2017 Pearson Education, Inc. Factors Affecting the Force Generated by Individual Muscle Fibers Frequency of stimulation: increases in the frequency of action potentials in muscle fibers increase tension in two ways Treppe Summation © 2017 Pearson Education, Inc. Figure 12.14 Treppe. Tension (g) Plateau Stimuli Time (msec) © 2017 Pearson Education, Inc. Factors Affecting the Force Generated by Individual Muscle Fibers Basis of summation Action potential 2 msec Contraction 10–200 msec Contractions can overlap and sum © 2017 Pearson Education, Inc. Figure 12.15 Duration of an isometric twitch relative to an action potential. Tension (g) Action potential 0 © 2017 Pearson Education, Inc. 20 40 60 80 Time (msec) 100 120 140 Factors Affecting the Force Generated by Individual Muscle Fibers Fiber diameter Force-generating capacity = inherent ability of muscle to generate force Force-generating capacity depends on the number of crossbridges in each sarcomere and the geometric arrangement of sarcomeres More crossbridges/sarcomere → more force More sarcomeres in parallel → more force Number of thick and thin filaments/area = constant Fiber diameter varies Larger diameter → more filaments → more force © 2017 Pearson Education, Inc. Factors Affecting the Force Generated by Individual Muscle Fibers Fiber length Length of fiber at the onset of contraction affects force generated Optimal length Resting length of muscle at which the fiber can develop the greatest amount of tension Due to maximum overlap of thick filament crossbridges and thin filaments In situ, most muscles are at optimal length © 2017 Pearson Education, Inc. Factors Affecting the Force Generated by Individual Muscle Fibers Nonoptimal lengths Greater than optimum Decrease crossbridge overlap Less than optimum Thin filaments overlap each other Z lines contact thick filaments © 2017 Pearson Education, Inc. Tension (g) Figure 12.16 Effects of high stimulus frequency: summation and tetanus. Stimuli Average tension (constant) Twitch Summation Incomplete tetanus (unfused) Time (msec) © 2017 Pearson Education, Inc. Complete tetanus (fused) Factors Affecting the Force Generated by Individual Muscle Fibers Cause of summation and tetanus Amount of tension developed depends on amount of Ca2+ bound to troponin At high frequencies, release exceeds reuptake Ca2+ increases in cytosol Eventually saturates system All troponin has Ca2+ bound to it Crossbridge cycling maxed out Maximum tetanic contraction © 2017 Pearson Education, Inc. Tension (% of maximum) Figure 12.17 A length-tension curve. 100 50 60 80 100 120 Decreased Normal length 140 160 Increased length Resting length of muscle (%) © 2017 Pearson Education, Inc. Regulation of the Force Generated by Whole Muscles More muscle fibers contracting → greater force Recruitment Stimulating more muscle fibers to contract Muscle = bundle of muscle fibers More muscle fibers contracting → greater overall muscle tension Recruitment occurs at the level of the motor unit © 2017 Pearson Education, Inc. Figure 12.18 Increases in force generation with recruitment of motor units. Motor unit X Motor unit Y Tension (g) 12 fibers 7 fibers 5 fibers Stimuli X © 2017 Pearson Education, Inc. Y X+Y Regulation of the Force Generated by Whole Muscles Motor unit recruitment More fibers contracting → greater tension Recruit motor units Activation of the motor neuron activates all muscle fibers in the motor unit Increases in tension occur in steps proportional to the size of the motor unit Muscles for delicate movements Small motor units Muscles for strength Large motor units © 2017 Pearson Education, Inc. Regulation of the Force Generated by Whole Muscles Motor unit sizes Number of motor units varies in different muscles Size of motor units varies Small: for delicate movements Large: for strength movements Fiber diameter (and thus strength) varies in motor unit Small: for weaker movements Large: for stronger movements © 2017 Pearson Education, Inc. Regulation of the Force Generated by Whole Muscles Motor unit sizes Size of the motor unit and fiber diameter are often related Small motor units → small fibers Small motor neuron cell bodies Small axon diameters Large motor units → large fibers Large motor neuron cell bodies Large axon diameters © 2017 Pearson Education, Inc. Regulation of the Force Generated by Whole Muscles The size principle Order of motor unit recruitment is related to size of motor units Small units recruited first Large units recruited last Larger neurons are more difficult to depolarize to threshold Requires greater synaptic input Small neurons excited first (low input), then large neurons (high input) © 2017 Pearson Education, Inc. Figure 12.19 The size principle. Brain W X Y Z Spinal cord Tension (g) Z Y X Excitatory input (action potential frequency in W) © 2017 Pearson Education, Inc. Velocity of Shortening When muscle contracts isotonically Latent period of shortening increases with increasing load Duration of shortening decreases with increasing load Velocity of shortening decreases with increasing load Rate of change of the distance is shortened Reaches 0 when load ≥ maximum tension Greatest when no load on muscle © 2017 Pearson Education, Inc. Figure 12.20 The effect of load on muscle shortening. Distance shortened Slope = velocity of shortening Zero load Intermediate load Heavy load 0 20 40 60 80 Time (msec) © 2017 Pearson Education, Inc. 100 120 Figure 12.21 A load-velocity curve. Shortening velocity Maximum velocity (zero load) 0 © 2017 Pearson Education, Inc. Load Load is greater than or equal to maximum tension (force) of muscle