Chapter 11 - Water - Groundwater PDF
Document Details
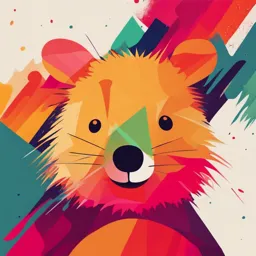
Uploaded by TruthfulArtInformel5102
Victoria College
Tags
Summary
This chapter explores groundwater, a significant source of freshwater. It details the processes of groundwater formation, movement, and extraction from aquifers. The summary covers porosity, permeability, and aquifer characteristics, including groundwater flow, mining, and contamination. The chapter also describes methods for understanding and managing groundwater resources, including water quality and remediation techniques
Full Transcript
WATER | 329 Take this quiz to check your comprehension of this section. If you are using an offline version of this text, access the quiz for section 11.5 via the QR code. An interactive H5P element has been excluded from this version of the text. You can view it onlin...
WATER | 329 Take this quiz to check your comprehension of this section. If you are using an offline version of this text, access the quiz for section 11.5 via the QR code. An interactive H5P element has been excluded from this version of the text. You can view it online here: https://pressbooks.lib.vt.edu/introearthscience/?p=765#h5p-80 11.6 Groundwater Groundwater is an important source of freshwater. It can be found at varying depths in all places under the ground, but is limited by extractable quantity and quality. One or more interactive elements has been excluded from this version of the text. You can view them online here: https://www.youtube.com/watch?v=g7R0yLX0V9E Video 11.7: What is an aquifer? If you are using an offline version of this text, access this YouTube video via the QR code. 11.6.1 Porosity and Permeability An aquifer is a rock unit that contains extractable ground water. A good aquifer must be both porous and permeable. Porosity is the space between grains that can hold water, expressed as the percentage of open space in the total volume of the rock. Permeabilitycomes from connectivity of the spaces that allows water to move in the aquifer. Porosity can occur as primary porosity, as space between sand grains or vesicles in volcanic rocks, or secondary porosity as fractures or dissolved spaces in rock). Compaction and cementation during lithification of sediments reduces porosity (see section 5.3). A combination of a place to contain water (porosity) and the ability to move water (permeability) makes a good aquifer—a rock unit or sediment that allows extraction of groundwater. Well-sorted sediments have higher porosity because there are not smaller sediment particles filling in the spaces between the larger particles. Shales made of clays generally have high porosity, but the pores are poorly connected, thereby causing low permeability. 330 | WATER One or more interactive elements has been excluded from this version of the text. You can view them online here: https://www.youtube.com/watch?v=8mfBomrw0rs Video 11.8: Porosity and permeability. If you are using an offline version of this text, access this YouTube video via the QR code. While permeability is an important measure of a porous material’s ability to transmit water, hydraulic conductivity is more commonly used by geologists to measure how easily a fluid is transmitted. Hydraulic conductivity measures both the permeability of the porous material and the properties of the water, or whatever fluid is being transmitted like oil or gas. Because hydraulic conductivity also measures the properties of the fluid, such as viscosity, it is used by both petro leum geologists and hydrogeologists to describe both the production capability of oil reservoirs and of aquifers. High hydraulic conductivity indicates that fluid transmits rapidly through an aquifer. 11.6.2 Aquifers Aquifers are rock layers with sufficient porosity and permeability to allow water to be both contained and move within them. For rock or sediment to be considered an aquifer, its pores must be at least partially filled with water and it must be permeable enough to transmit water. Drinking water aquifers must also contain potable water. Aquifers can vary dra- matically in scale, from spanning several formations covering large regions to being a local formation in a limited area. Aquifers adequate for water supply are both permeable, porous, and potable. 11.6.3 Groundwater Flow When surface water infiltrates or seeps into the ground, it usually enters the unsaturated zone also called the vadose zone, or zone of aeration. The vadose zone is the volume of geologic material between the land surface and the zone of saturation where the pore spaces are not completely filled with water. Plant roots inhabit the upper vadose zone and fluid pressure in the pores is less than atmospheric pressure. Below the vadose zone is the capillary fringe. Capillary fringe is the usually thin zone below the vadose zone where the pores are completely filled with water (saturation), but the fluid pressure is less than atmospheric pressure. The pores in the capillary fringe are filled because of capillary action, which occurs because of a combination of adhesion and cohesion. Below the capillary fringe is the saturated zone or phreatic zone, Figure 11.31: Zone of saturation. where the pores are completely saturated and the fluid in the pores is at or above atmospheric pressure. The interface between the capillary fringe and the saturated zone marks the location of the water table. Wells are conduits that extend into the ground with openings to the aquifers, to extract from, measure, and sometimes add water to the aquifer. Wells are generally the way that geologists and hydrologist measure the depth to groundwater from the land surface as well as withdraw water from aquifers. WATER | 331 Water is found throughout the pore spaces in sediments and bedrock. The water table is the area below which the pores are fully saturated with water. The simplest case of a water table is when the aquifer is unconfined, meaning it does not have a confining layer above it. Confining layers can pressurize aquifers by trapping water that is recharged at a higher elevation underneath the confining layer, allowing for a potentiometric surface higher than the top of the aquifer, and sometimes higher than the land surface. A confining layer is a low permeability layer above and/ or below an aquifer that restricts the water from moving in and out of the aquifer. Confining layers include aquicludes, which are so impermeable that no water trav- els through them, and aquitards, which significantly decrease the speed at which water travels through them. The potentiometric surface represents the height that water would rise in a well penetrating the pressurized aquifer system. Breaches in the pressurized aquifer sys tem, like faults or wells, can cause springs or flowing wells, also known as artesian wells. The water table will generally mirror surface topography, though more subdued, because hydrostatic pressure is equal to atmospheric pressure along the surface of the water table. If the water table intersects the ground sur- face the result will be water at the surface in the form of a gaining stream, spring, lake, or wetland. The water table intersects the channel for gaining streams which then gains water from the water table. The channels for losing Figure 11.32: An aquifer cross-section. This diagram shows two aquifers with one aquitard (a confining or impermeable layer) between them, streams lie below the water table, thus losing streams surrounded by the bedrock aquiclude, which is in contact with a gaining lose water to the water table. Losing streams may be sea- stream (typical in humid regions). sonal during a dry season or ephemeral in dry climates where they may normally be dry and carry water only after rain storms. Ephemeral streams pose a serious danger of flash flooding in dry climates. One or more interactive elements has been excluded from this version of the text. You can view them online here: https://www.youtube.com/watch?v=UfgyJkmZgK8 Video 11.9: Where is the water table? If you are using an offline version of this text, access this YouTube video via the QR code. Mentioned in the video is the USGS Groundwater Watch site. Using wells, geologists measure the water table’s height and the potentiometric surface. Graphs of the depth to groundwater over time, are known as hydrographs and show changes in the water table over time. Well–water level is controlled by many factors and can change very frequently, even every minute, seasonally, and over longer peri ods of time. 332 | WATER In 1856, French engineer Henry Darcy developed a hypothesis to show how discharge through a porous medium is con trolled by permeability, pressure, and cross–sectional area. To prove this relationship, Darcy experimented withtubes of packed sediment with water running through them. The results of his experiments empirically established a quantitative measure of hydraulic conductivity and discharge that is known as Darcy’s law. The relationships described by Darcy’s Law have close similarities to Fourier’s law in the field of heat conduction, Ohm’s law in the field of electrical networks, or Fick’s law in diffusion theory. Q=KA(Δh/L) Q = flow (volume/time) K = hydraulic conductivity (length/time) A = cross-sectional area of flow (area) Δh = change in pressure head (pressure difference) L = distance between pressure (h) measurements (length) Δh/L is commonly referred to as the hydraulic gradi ent Pumping water from an unconfined aquifer lowers Figure 11.33: Pipe showing apparatus that would demonstrate Darcy’s the water table. Pumping water from a confined aquifer Law. Δh would be measured across L from a to b. lowers the pressure and/or potentiometric sur face around the well. In an unconfined aquifer, the water table is lowered as water is removed from the aquifer near the well producing drawdown and a cone of depression (see figure 11.34). In a confined aquifer, pumping on an artesian well reduces the pressure or potentiometric surface around the well. When one cone of depression intersects another cone of depression or a barrier feature like an impermeable moun tain block, drawdown is intensified. When a cone of depression intersects a recharge zone, the cone of depression is lessened. 11.6.4 Recharge The recharge area is where surface water enters an aquifer through the process of infiltration. Recharge areas are generally topographically high locations of an aquifer. They are characterized by losing streams and permeable rock that allows infiltration into the aquifer. Recharge areas mark the beginning of groundwater flow paths. Figure 11.34: Cones of depression. In the Basin and Range Province, recharge areas for the unconsolidated aquifers of the valleys are along mountain foothills. In the foothills of Salt Lake Val ley, losing streams contribute water to the gravel-rich deltaic deposits of ancient Lake Bonneville, in some cases feeding artesian wells in the Salt Lake Valley. An aquifer management practice is to induce rechargethrough storage and recovery. Geologists and hydrolo gists can increase the recharge rate into an aquifer system using injection wells and infiltration galleries or basins. Injec tion wells pump water into an aquifer where it can be stored. Injection wells are regulated by state and federal governments to ensure that the injected water is not negatively impacting the quality or supply of the existing groundwater in the aquifer. Some aquifers can store significant quantities of water, allowing water managers to use the aquifer system WATER | 333 like a surface reservoir. Water is stored in the aquifer during periods of low water demand and high water supply and later extracted during times of high water demand and low water supply. Figure 11.35: Different ways an aquifer can be recharged. 11.6.5 Discharge Discharge areas are where the water table or potentiometric surface intersects the land surface. Discharge areas mark the end of groundwater flow paths. These areas are characterized by springs, flowing (artesian) wells, gaining streams, and playas in the dry valley basins of the Basin and Range Province of the western United States. 11.6.6 Groundwater Mining and Subsidence Like other natural resources on our planet, the quantity of fresh and potable water is finite. The only natural source of water on land is from the sky in the form of precipitation. In many places, groundwater is being extracted faster than it is being replenished. When groundwater is extracted faster than it is recharged, groundwater levels and potentiometric surfaces decline, and discharge areas diminish or dry up completely. Regional pumping-induced groundwater decline is known as groundwater mining or groundwater overdraft. Groundwater mining is a serious situation and can lead to dry wells, reduced spring and stream flow, and subsidence. Groundwater mining is happeningis places where more water is extracted by pumping than is being replenished by precipitation, and the water table is continually lowered. In these situations, groundwater must be viewed as a ore body and in its depletion, the possibility of producing ghost towns. One or more interactive elements has been excluded from this version of the text. You can view them online here: https://www.youtube.com/watch?v=_DuwZjb3_DA Video 11.10: Groundwater. If you are using an offline version of this text, access this YouTube video via the QR code. 334 | WATER In many places, water actually helps hold up an aquifer’s skeleton by the water pressure exerted on the grains in an aquifer. This pressure is called pore pressure and comes from the weight of overly ing water. If pore pressure decreases because of groundwater mining, the aquifer can compact, causing the surface of the ground to sink. Areas especially susceptible to this effect are aquifers made of unconsolidated sediments. Unconsolidated sediments with multiple layers of clay and other fine-grained material are at higher risk because when water is drained, clay com pactsconsiderably. Subsidence from groundwater mining has been documented in southwestern Utah, notably Cedar Valley, Iron County, Utah. Groundwater levels have declined more than 100 feet in certain parts of Cedar Valley, causing earth fissures and measurable amounts of land subsidence. This photo shows documentation of subsidence from pumping of groundwater for irrigation in the Central Valley in California. The pole shows subsidence from groundwater pumping over a period of time. Figure 11.36: Evidence of land subsidence from pumping of groundwater shown by dates on a pole. Take this quiz to check your comprehension of this section. If you are using an offline version of this text, access the quiz for section 11.6 via the QR code. An interactive H5P element has been excluded from this version of the text. You can view it online here: https://pressbooks.lib.vt.edu/introearthscience/?p=765#h5p-81 11.7 Water Contamination and Remediation Water can be contaminated by natural features like mineral-rich geologic formations and by human activities such as agriculture, industrial operations, landfills, animal operations, and sewage treatment processes, among many other things. As water runs over the land or infiltrates into the ground, it dissolves material left behind by these potential con taminant sources. There are three major groups of contamination: organic and inorganic chemicals and biological agents. Small sediments that cloud water, causing turbidity, is also an issue with some wells, but it is not considered contamina tion. The risks and type of remediation for a contaminant depends on the type of chemicals present. Contamination occurs as point–source and nonpoint–source pollution. Point source pollution can be attributed to a single, definable source, while nonpoint source pollution is from multiple dispersed sources. Point sources include waste dis- posal sites, storage tanks, sewage treatment plants, and chemical spills. Nonpoint sources are dispersed and indiscreet, where the whole of the contribution of pollutants is harmful, but the individual components do not have harmful concen- WATER | 335 trations of pollutants. A good example of nonpoint pollution is residential areas, where lawn fertilizer on one person’s yard may not contribute much pollution to the system, but the combined effect of many residents using fertilizer can lead to significant nonpoint pollution. Other nonpoint sources include nutrients (nitrate and phosphate), herbicides, pesticides con- tributed by farming, nitrate contributed by animal operations, and nitrate contributed by septic systems. Organic chemicals are common pollutants. They consist of strands and rings of carbon atoms, usually connected by cova lent bonds. Other types of atoms, like chlorine, and molecules, like hydroxide (OH–), are attached to the strands and rings. The number and arrangement of atoms will decide how the chemical behaves in the environment, its danger to humans or ecosystems, and where the chemical ends up in the environment. The different arrangements of carbon allow for tens of thousands of organic chemicals, many of which have never been studied for negative effects on human health or the environment. Common organic pollutants are herbicides and pesticides, pharmaceuticals, fuel, and industrial solvents and cleansers. Organic chemicals include surfactants such as cleaning agents and synthetic hormones associated with pharmaceuticals, which can act as endocrine disruptors. Endocrine disruptors mimic hormones, and can cause long-term effects in devel oping sexual reproduction systems in developing animals. Only very small quantities of endocrine disruptors are needed to cause significant changes in animal populations. An example of organic chemical contamination is the Love Canal, Niagara Falls, New York. From 1942 to 1952, the Hooker Chemical Company disposed of over 21,337 mt (21,000 t) of chemical waste, including chlorinated hydrocarbons, into a canal and covered it with a thin layer of clay. Chlorinated hydrocarbons are a large group of organic chemicals that have chlorine functional groups, most of which are toxic and carcinogenic to humans. The company sold the land to the New York School Board, who developed it into a neighborhood. After residents began to suffer from serious health ailments and pools of oily fluid started rising into residents’ basements, the neighborhood had to be evacuated. This site became a U.S. Environmental Protection Agency Superfund site, a site with federal funding and oversight to ensure its cleanup. Inorganic chemicals are another set of chemical pollutants. They can contain carbon atoms, but not in long strands or links. Inorganic contaminants include chloride, arsenic, and nitrate (NO3). Nutrients can be from geologic material, like phospho rous-rich rock, but are most often sourced from fertilizer and animal and human waste. Untreated sewage and agricultural runoff contain concentrates of nitrogen and phosphorus which are essential for the growth of microorganisms. Nutrients like nitrate and phosphate in surface water can promote growth of microbes, like blue-green algae (cyanobacteria), which in turn use oxygen and create toxins (microcystins and anatoxins) in lakes. This process is known as eutrophication. Metals are common inorganic contaminants. Lead, mercury, and arsenic are some of the more problematic inorganic groundwater contaminants. Bangladesh has a well documented case of arsenic contamination from natural geologic material dissolving into the groundwater. Acid–mine drainage can also cause significant inorganic contamination (see chapter 16). Salt, typically sodium chloride, is a common inorganic contaminant. It can be introduced into groundwater from natural sources, such as evaporite deposits like the Arapien Shale of Utah, or from anthropogenic sources like the salts applied to roads in the winter to keep ice from forming. Salt contamination can also occur near ocean coasts from saltwater intruding into the cones of depression around fresh groundwater pumping, inducing the encroachment of saltwater into the fresh water body. Biological agents are another common groundwater contaminant which includes harmful bacteria and viruses. A com mon bacteria contaminant is Escherichia coli (E. coli). Generally, harmful bacteria are not present in groundwater unless the groundwater source is closely connected with a contaminated surface source, such as a septic system. Karst, land forms created from dissolved limestone, is especially susceptible to this form of contamination, because water moves relatively quickly through theconduits of dissolved limestone. Bacteria can also be used for remediation. View USGS tables on contaminants found in groundwater. 336 | WATER Remediation is the act of cleaning contamination. Hydrologists use three types of remediation: biological, chemical, and physical. Biological remediation uses specific strains of bacteria to break down a contaminant into safer chemicals. This type of remediation is usually used on organic chemicals, but also works on reducing or oxidizing inorganic chemicals like nitrate. Phytoremediation is a type of bioremediation that uses plants to absorb the chemicals over time. Chemical remediation uses chemicals to remove the contaminant or make it less harmful. One example is to use a reactive barrier, a permeable wall in the ground or at a discharge point that chemically reacts with contaminants in the water. Reac tive barriers made of limestone can increase the pH of acid mine drainage, making the water less acidic and more basic, which removes dissolved contaminants by precipitation into solid form. Physical remediation consists of removing the contaminated water and either treating it with filtration, called pump–and–treat, or disposing of it. All of these options are technically complex, expensive, and difficult, with physi cal remediation typically being the most costly. Take this quiz to check your comprehension of this section. If you are using an offline version of this text, access the quiz for section 11.7 via the QR code. An interactive H5P element has been excluded from this version of the text. You can view it online here: https://pressbooks.lib.vt.edu/introearthscience/?p=765#h5p-82 11.8 Karst Karst refers to landscapes and hydrologic features cre ated by the dissolving of limestone. Karst can be found anywhere there is limestone and other soluble subter ranean substances like salt deposits. Dissolving of lime stone creates features like sinkholes, caverns, disappearing streams, and towers. Figure 11.37: Steep karst towers in China left as remnants as limestone is dissolved away by acidic rain and groundwater. WATER | 337 Figure 11.38: Sinkholes of the McCauley Sink in Northern Arizona, produced by collapse of Kaibab Limestone into caverns caused by solution of underlying salt deposits. Dissolving of underlying salt deposits has caused sinkholes to form in the Kaibab Limestone on the Colorado Plateau in Arizona. Figure 11.39: This sinkhole from collapse of surface into a underground cavern appeared in the front yard of this home in Florida. Collapse of the surface into an underground cavern caused this sinkhole in the front yard of a home in Florida. CO2 in the atmosphere dissolves readily in the water droplets that form clouds from which precipitation comes in the form of rain and snow. This precipitation is slightly acidic with carbonic acid. Karst forms when carbonic acid dis solves calcite (calcium carbonate) in limestone. H2O + CO2 = H2CO3 Water + Carbon Dioxide Gas equals Carbonic Acid in Water CaCO3 + H2CO3 = Ca2++ 2HCO3 -1 Solid Calcite + Carbonic Acid in Water Dissolved equals Calcium Ion + Dissolved Bicarbonate Ion 338 | WATER After the slightly acidic water dissolves the calcite, changes in temperature or gas content in the water can cause the water to redeposit the calcite in a different place as tufa (travertine), often deposited by a spring or in a cave. Speleothems are secondary deposits, typically made of travertine, deposited in a cave. Travertine speleothems form by water dripping through cracks and dissolved openings in caves and evaporating, leaving behind the travertine deposits. Speleothems commonly occur in the form of stalactites, when extending from the ceiling, and stalagmites, when standing up from the floor. Figure 11.40: Mammoth hot springs, Yellowstone National Park. Figure 11.41: Varieties of speleotherms. Surface water enters the karst system through sinkholes, losing streams, and disappearing streams. Changes in base level can cause rivers running over limestone to dis solve the limestone and sink into the ground. As the water continues to dissolve its way through the limestone, it can leave behind intricate networks of caves and narrow pas sages. Often dissolution will follow and expand fractures in the limestone. Water exits the karst system as springs and rises. In mountainous terrane, dissolution can extend all the way through the vertical profile of the mountain, with caverns dropping thousands of feet. Figure 11.42: This stream disappears into a subterranean cavern system to re-emerge a few hundred yards downstream. WATER | 339 Take this quiz to check your comprehension of this section. If you are using an offline version of this text, access the quiz for section 11.8 via the QR code. An interactive H5P element has been excluded from this version of the text. You can view it online here: https://pressbooks.lib.vt.edu/introearthscience/?p=765#h5p-83 Summary Water is essential for all living things. It continuously cycles through the atmosphere, over land, and through the ground. In much of the United States and other countries, water is managed through a system of regional laws and regulations and distributed on paper in a system collectively known as “water rights”. Surface water follows a watershed, which is sepa rate from other areas by its divides (highest ridges). Groundwater exists in the pores within rocks and sediment. It moves predominantly due to pressure and gravitational gradients through the rock. Human and natural causes can make water unsuitable for consumption. There are different ways to deal with this contamination. Karst is when limestone is dissolved by water, forming caves and sinkholes. Take this quiz to check your comprehension of this chapter. If you are using an offline version of this text, access the quiz for chapter 11 via the QR code. An interactive H5P element has been excluded from this version of the text. You can view it online here: https://pressbooks.lib.vt.edu/introearthscience/?p=765#h5p-84 URLs Linked Within This Chapter NPR story on Snake Valley: https://www.npr.org/2007/06/12/10953190/las-vegas-water-battle-crops-vs-craps SNWA History: https://www.snwa.com/about/mission/ index.html#:~:text=In%201991%2C%20seven%20local%20water,Big%20Bend%20Water%20District Dean Baker Story: The Consequences: Transporting Snake Valley water to satisfy a thirsty Las Vegas. [Video: 25:24] https://www.youtube.com/watch?v=eCZ8KLrmUXo NASA images: https://earthobservatory.nasa.gov/images/8103/mississippi-river-delta