Basic Pharmacology PDF
Document Details
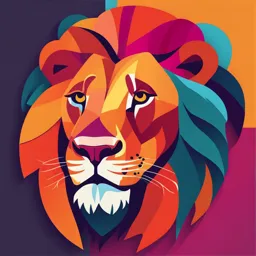
Uploaded by WellBeingMoose
Tags
Summary
This document is an introductory chapter on basic pharmacology, covering drug sources, classifications, and administration routes. It discusses the different sources of drugs and provides examples of plant-derived pharmacological agents.
Full Transcript
1 Basic Pharmacology OBJECTIVES After completing this chapter, you should be able to do the following: 1. Define terms and abbreviations related to pharmacology. 2. State sources of drugs and list examples of drugs from each source. 3. List and describe several classes of drugs relevant to s...
1 Basic Pharmacology OBJECTIVES After completing this chapter, you should be able to do the following: 1. Define terms and abbreviations related to pharmacology. 2. State sources of drugs and list examples of drugs from each source. 3. List and describe several classes of drugs relevant to surgical practice. 4. Explain medication orders used both in prescriptions and in surgery. 5. List the parts of a medication order used in surgery. 6. Identify drug distribution systems used in hospitals. 7. List and describe types of drug forms. 8. Compare and contrast medication administration routes used in surgery. 9. Explain the four processes of pharmacokinetics. 10. Identify and discuss aspects of pharmacodynamics. The science of pharmacology is a diverse study of the interactions between chemicals and biological systems. In the broadest sense, it includes toxicology, food science, agriculture, and medicine. When chemicals are used to treat diseases, we call them drugs or medications. Medical pharmacology is a rapidly expanding field of study because new drugs are being developed nearly every day. An understanding of basic principles in pharmacology can help the surgical technologist deal with such constant developments. Students should seek to build a framework of principles so they can incorporate new information more easily. When a new drug is introduced into surgical practice, the surgical technologist should be able to understand information about the drug by applying the principles of pharmacology. This chapter presents an introduction to the foundations of pharmacology that can be applied throughout a professional career in surgical technology. Drug Sources Drugs in use today come from three main sources: natural sources, chemical synthesis, and biotechnology. Natural sources include plants, animals, and minerals. The study of drugs derived from natural sources is called pharmacognosy. At one time, plants were nearly the only source of medicines available. Today, only a few prescription drugs relevant to surgical practice are still derived directly from plant sources. Examples of current drugs made from plants include atropine from the roots of the belladonna plant (Atropa belladonna; deadly nightshade; Fig. 1.1), digitalis from the leaves of the purple foxglove, and morphine from the seeds of the opium poppy (Table 1.1). The trend toward alternative medicines has initiated a closer look at plants as sources of important and helpful chemicals in the natural state (Insight 1.1). Chemicals produced by plants also hold great promise in the development of drugs to treat cancer. One such drug is paclitaxel (Taxol), which is derived from Taxus baccata and is used to treat breast cancer. Animals provide a source for some drugs, particularly hormones. Cattle and hog endocrine glands were the best available source of hormones before the advent of biotechnology. We describe drugs derived from hogs as porcine and those from cattle as bovine. Thus thyroglobulin (Proloid)—a purified extract of hog thyroid gland—is porcine in origin, whereas thrombin (Thrombogen)—a topical hemostatic—is bovine in origin. The early form of insulin is both bovine and porcine because it was obtained from the pancreas of cattle and hogs. Estrogen was another hormone obtained from an animal source. Conjugated estrogen (Premarin) was obtained from the urine of pregnant horses and so is referred to as equine in nature. FIG. 1.1 Atropa belladonna. Courtesy Martin Wall Botanical Services. (From Ulbrich C: Natural standard herbal pharmacotherapy, ed 1, St Louis, 2010, Elsevier. TABLE 1.1 Examples of Plant-Derived Drugs Relevant to Surgical Practice Drug Category Plant Atropine Anticholinergic Atropa belladonna Cocaine Local anesthetic Erythroxylum coca Digoxin Cardiac agent Digitalis purpurea Ephedrine Sympathomimetic Ephedra sinica Morphine Analgesic Papaver somniferum Papaverine Smooth muscle relaxant Papaver somniferum Pilocarpine Parasympathomimetic Pilocarpus jaborandi Minerals, such as calcium, magnesium, and silver salts in several forms, are used in some pharmacological agents. For example, Tums and Mylanta are antacids that contain calcium (Tums) and magnesium (Mylanta) hydroxides. Silver sulfadiazine (Silvadene cream) is an antimicrobial agent used in dressings for burn patients that contains silver salts (see Chapter 5). Even gold is used, as in aurothioglucose (Solganal), an antiarthritic agent. The second major source of drugs is chemical synthesis in the laboratory (Fig. 1.2). There are two ways for drugs to be synthesized, that is, put together. Synthetic drugs are drugs that are synthesized from laboratory chemicals. Semisynthetic drugs are drugs that start with a natural substance that is extracted, purified, and altered by chemical processes. The vast majority of modern drugs are either synthetic or semisynthetic. Meperidine (Demerol) is an example of a synthetic drug; it is made from chemicals, yet its pain-relieving effects are similar to those of opium. Many types of penicillin, such as amoxicillin, are semisynthetic drugs. The penicillin group of drugs was originally derived from a natural mold (Penicillium), the active substance of which is extracted and purified in the chemical laboratory. Another example of semisynthetic drugs is the aminoglycoside group of antibiotics, the active substance of which is obtained from the bacterial species Streptomyces. An increasing source of drugs has been provided by the science of biotechnology. The term biotechnology is used to refer to the concepts of genetic engineering and recombinant deoxyribonucleic acid (DNA) technology. The science of biotechnology has many applications in pharmacology and has provided significant improvements in the treatment of various conditions. Biotechnology is a process that allows scientists to produce proteins from bacteria— proteins that were previously available only from animals. Molecular biologists use bacteria as tiny factories to produce the proteins they need to make drugs. They do this by altering the DNA of bacteria, such as Escherichia coli (E. coli). How? By physically inserting a gene into the DNA of a single E. coli cell—a gene that codes for (tells the cell to make) a certain protein (Fig. 1.3). When the bacterial cell has this gene incorporated into its DNA, it becomes a miniature copying machine, producing daughter cells that have daughter cells that have daughter cells—each with the new gene and each producing the desired protein. Because this reproduction process occurs very rapidly, large volumes of the desired protein can be obtained quickly. The specific protein is extracted and purified in the laboratory and prepared for administration into a patient. Molecular biologists also use cultures of mammalian cell lines, such as genetically altered Chinese hamster ovary (CHO) cells, to produce various therapeutic proteins. Adalimumab (Humira) is an example of a drug developed with recombinant DNA technology and mammalian cell lines. In general, CHO cells provide more stable gene expression and higher volumes of the desired proteins than bacterial cells and are becoming the primary choice of cell lines for pharmacological use. INSIGHT 1.1 In Search of New Drugs Sometimes the quest for new drugs involves some very old sources. It has long been said that a glass of wine is good for you, and archaeologists know the ancient Egyptians thought the same. When the 5100-year-old tomb of Pharaoh Scorpion I was excavated, more than 700 jars were discovered in one of the chambers. Some of the jars contained wine residue with medicinal additives. These additives might have come from a relative of the present-day wormwood (Artemisia sieberi), blue tansy, herbs, and tree resins. The jars were imported from several sites in the ancient world, in what we know today as Israel and Palestine. These jars demonstrate how humans from thousands of years ago had turned to their natural environments for effective plant remedies. It will take our modern technology using biomolecular analysis to isolate these active components. Scientists are working with physicians to see whether any of these compounds might be useful today—perhaps in the fight against cancer. FIG. 1.2 A pharmaceutical laboratory. iStock.com/LajosRepasi FIG. 1.3 Biotechnology.(A) Escherichia coli deoxyribonucleic acid (DNA). (B) Desired gene is inserted into bacterial DNA. (C) Bacterial DNA with recombinant gene. Among the drugs produced by biotechnology are human insulin (Humulin), human growth hormone (Nutropin), human thyroid- stimulating hormone (Thyrogen), and the thrombolytic agent alteplase (Activase). Drugs such as these are always administered by injection; they cannot be taken orally because they are proteins, which are digested when consumed. Genetically engineered proteins do not cause the adverse side effects—for example, immune or allergic reactions—often seen in the long-term use of drugs from animal sources. The hepatitis B vaccine (Engerix-B, Recombivax HB) is another product of biotechnology. This vaccine is of particular interest to surgical technologists because it is one of the required vaccinations for healthcare providers. Drug Classifications Drug classifications are used to group drugs that are used for similar purposes (Table 1.2). Learning the drug classification of common medications is particularly helpful to the surgical technologist in practice, because many classification titles will provide clues to the use or purpose of a medication. For example, a vasoconstrictor will cause contraction of the walls of blood vessels and restrict blood flow. An ophthalmic agent is specially formulated for use in the eye only, and an otic medication is specially formulated for use in the ear. Make It Simple Use of medical terminology word-building techniques to define the drug class will help in the understanding of a medication’s purpose —for example, anesthetic: “an-” means without and “-esthesia” means sensation. Thus an anesthetic agent causes a loss of sensation. Another example, thrombolytic: “thrombo-” means blood clot, “-lysis” is to break down, and “-tic” is a suffix meaning pertaining to. Thus a thrombolytic is a medication given to break down a blood clot. Some drugs can be listed in multiple classes. For example, ranitidine (Zantac) is categorized as an antacid, a histamine receptor antagonist, and a gastric agent. Aspirin relieves pain, fever, and inflammation, so it is classified as an analgesic, antipyretic, and antiinflammatory agent. We can also classify drugs in broader categories by what they do, what they affect, and what they are; thus classification categories may include the following: Therapeutic action: what they do for a patient; for example, analgesics relieve pain. Physiological action: what they do in the body; for example, histamine receptor antagonists block histamine production. Affected body system: what they affect; for example, cardiovascular agents affect the heart and circulatory system. Chemical type: what they are; for example, barbiturates are a class of chemical compounds derived from barbituric acid. Tech Tip Classes of drugs frequently used from the sterile back table include antibiotics, anticoagulants, antiinflammatory agents, and local anesthetics. Drugs are also classified by how they may be obtained. The distinction between prescription and nonprescription, or over-the- counter (OTC), drugs is a legal classification. In addition, some prescription medications are classified as controlled substances. Legal classifications are discussed in Chapter 2. Medication Orders Prescriptions When treatment requires a specific drug, a licensed physician or designee, such as a physician assistant or nurse practitioner, writes a prescription for the drug. State governments have the power to regulate which medical professionals write prescriptions, so there may be variations in practice from state to state. Fig. 1.4 shows a typical written prescription form. As shown, prescriptions must include the date, name of the patient, name of the drug, dosage, route of administration, and frequency or time of administration. It must also bear the prescriber’s signature. Notice that the printed form contains the name, address, telephone number, and Drug Enforcement Administration (DEA) number of the prescriber. The DEA requires that this number be listed on any prescription for a controlled substance (see Chapter 2). A written prescription usually designates the drug by trade name but may indicate that a generic substitution is permissible. When writing prescriptions, physicians (or their designees) use abbreviations and symbols (Table 1.3) for directions, dosages, frequency, and administration routes. Pharmacists interpret these symbols and give the drugs to the patient, along with instructions for proper use. Many pharmacies use computer database systems that provide specific, detailed printouts to the patient of such important drug information as side effects, precautions, normal usage, and storage. Prescriptions, such as shown, are not generally used during a patient’s hospitalization, so they have little or no use during surgery. TABLE 1.2 FIG. 1.4 A typical prescription form. TABLE 1.3 Written prescriptions occasionally present difficulties when handwriting is interpreted. The introduction of electronic prescribing, or e-prescribing, may significantly reduce medication errors that are due to misinterpretation of poorly handwritten prescriptions. E- prescribing is the process of generating, transmitting, and filing of prescriptions through a computer-based system (Fig. 1.5). These systems also provide ready access to important prescriber safety information, such as patient medication history and allergies. In the hospital setting, any medications to be administered to the patient must be ordered by a licensed physician or designee and written on a physician’s order sheet or entered into the electronic prescribing system. Medication Orders in Surgery In surgery, the medication order may be one of several types, such as standing orders, verbal orders, ‘immediately’ (STAT) orders, and ‘as needed’ (PRN) orders. A standing order, or protocol, is used for common situations requiring a standard treatment. For example, institutions participating in the Surgical Care Improvement Project may have a standing order in place stating that all patients undergoing specified surgical procedures are to receive a particular prophylactic antibiotic 1 hour before the incision. In the operating room, surgeons’ preference cards contain standing orders for specific surgical procedures. For example, Dr. Vigil’s preference card for abdominal aortic aneurysm repair may include a standing order for 5000 units of heparin (a systemic anticoagulant, see Chapter 9) in 1000 mL of sodium chloride solution (saline, 0.9% NaCl) for topical irrigation. A standing order of this type informs the operating room team that the indicated medication should be ready on the sterile back table as a standard part of the setup for that procedure. Verbal orders are commonplace in surgery because a surgeon may request a particular drug to be administered either from the sterile field or by the anesthesia care provider. For example, during a carotid endarterectomy (approximately 3 minutes before the application of vascular clamps to the carotid artery), Dr. Vigil may give a verbal order to the anesthesia care provider to administer 5000 units of heparin intravenously (IV). Verbal orders in surgery are usually for a one-time single administration of a medication. The verbal order is documented in the patient’s record. Usually given verbally, STAT orders indicate that a drug is to be administered immediately and one time only. The most common use of STAT orders in surgery is during cardiac arrest resuscitation or other emergent situations. PRN stands for pro re nata, which means that the drug may be given as needed. For example, during septoplasty performed with the patient under local anesthesia, the analgesic meperidine (Demerol) may be administered PRN to reduce patient discomfort. In surgery, medications routinely needed during a procedure are listed on the surgeon’s preference card (see Fig. 10.4). The preference card should list all pertinent information, such as drug strength and quantity. Surgical medication orders on preference cards usually contain the drug name, strength, and volume. For example, when a radiopaque contrast medium (see Chapter 6) is needed, the preference card might state “Omnipaque 300—10 mL.” Unlike medication orders given in nursing care units, the surgeon administers all medications at the sterile field, so route of administration and frequency are not usually stated on surgeon’s preference cards. FIG. 1.5 An example screenshot of electronic prescribing. Courtesy SimChart for the Medical Office, Elsevier, Inc., 2016. INSIGHT 1.2 Practical Math—Calculating Drug Dosages at the Sterile Back Table Some drugs are available in various strengths, such as lidocaine. How much of the drug is given (dose) depends on the strength and the volume. The same dosage of lidocaine can be given in different ways. For example, 30 mL of 1% lidocaine delivers the same dosage as 15 mL of 2% lidocaine or 60 mL of 0.5% lidocaine. Many abbreviations are used to represent drug forms, dosages, routes, and timing of administration. Dosages are stated in a particular unit of measure, usually in the metric system, and abbreviated, such as 300 mg or 10 mL. The dosage of a medication is the medication strength (usually expressed as a percentage) multiplied by the volume administered (Insight 1.2). For example, lidocaine (Xylocaine) may be injected for local anesthesia (see Chapter 14) and therefore is listed on the preference card as “lidocaine plain 1%—30 mL.” This indicates that a 30-mL vial of a 1% solution of lidocaine without epinephrine should be delivered to the sterile back table and prepared for use (Fig. 1.6). It may not be necessary to use the entire 30 mL during the procedure, so the surgical technologist in the scrub role reports the final amount (volume) and strength used to the circulator and anesthesia care provider who record the dose. For regular hospital medication orders, the route of administration is usually abbreviated; for example, if a drug is to be given intravenously, it is designated as IV. The frequency or time of administration is also clearly stated and often abbreviated; for example, if the drug is to be taken twice a day, it is designated bid. Table 1.4 lists common abbreviations used to represent frequency of drug administration. Again, most surgeons’ preference cards do not list the route or frequency because the surgeon is administering all medications given at the surgical site. FIG. 1.6 Lidocaine label. Lidocaine 1% contains 1 g of medication per 100 mL solution and 10 mg per mL. From Morris DG: Calculate with confidence, ed 6, St Louis, 2014, Elsevier. Caution Some abbreviations have been associated with an increased risk of misinterpretation and possible medication errors. The Joint Commission, which accredits hospitals and other healthcare institutions, requires accredited facilities to publish a “do not use” list for potentially dangerous abbreviations, acronyms, and symbols (see Table 2.2). Before using what you may think are standard abbreviations, consult this list at your facility. Drug Distribution Systems Dispensing prescription drugs is the responsibility of a licensed pharmacist; that is, pharmacists must release drugs, either directly to patients or to the physician or surgeon who orders them. In hospitals, drugs are often released for secure storage in various patient care areas so they can be distributed as necessary. NOTE In all medical facilities, controlled substances (such as morphine) must be stored in a secure location once they have been released from the pharmacy. Individual institutional policies are put in place to account for and document the use of controlled substances. Distribution systems for drugs used in surgery vary among institutions. In large hospitals with many operating rooms, a satellite pharmacy within the surgical suite may dispense drugs as needed for each procedure. Other, often smaller, facilities may maintain a medication room or cabinet where they store frequently used drugs, such as antibiotics and local anesthetics. In addition, some hospitals use a system of mobile drug carts, which must be exchanged for restocking after the drugs are used. For example, emergency-response drug carts, known as crash carts, are used for cardiac arrest and other emergency situations (see Fig. 16.2). Such carts may be restocked on an exchange basis to ensure the immediate availability of all necessary drugs. TABLE 1.4 Abbreviations for Frequency of Medication Administration Abbreviation Meaning bid Twice a day h, hr Hour prn, PRN As necessary (pro re nata) q Every qh Every hour q2h Every 2 hours qid Four times a day tid Three times a day stat Immediately Computer-automated dispensing systems are frequently used for medication distribution in surgery (Fig. 1.7). These systems have been developed to minimize medication administration errors. A bar code- scanning system allows an approved user access to particular medications ordered for a particular patient. Drug Forms or Preparations Drugs are available in several different forms or preparations. Drugs may be in solid, semisolid, liquid, or gas form. The form of drug administered affects both the onset of drug actions and the intensity of the body’s response to the drug. Liquids, for example, tend to act more quickly than solids, and gases or vapors tend to act even faster. Drug form also dictates route of administration. For example, the antibiotic Neosporin comes in ointment (semisolid) form, which must be applied topically only. Some drugs are available in more than one form. For example, 2% lidocaine (Xylocaine), a local anesthetic agent, is available in jelly for topical application and in solutions of various strengths for injection. The names of drug forms are often abbreviated in drug orders. Table 1.5 lists several common abbreviations for drug forms. Solids Many drugs come prepared in solid form. These drugs may be in capsule (cap) or tablet (tab) form and are administered orally. Capsules are gelatin cases containing a drug in powder or granule form; tablets are a compressed form of the drug, usually combined with inert ingredients. Capsules and tablets are rarely used in surgery because oral administration is required. In most cases surgical patients must be kept NPO (from the Latin nil per os, nothing by mouth), or they may be under a general anesthesia and unable to swallow. Some drugs come in powder form and are contained in glass vials. Such powders must be mixed with a liquid (reconstituted) to form a solution that can be administered by injection (Fig. 1.8). For example, several antibiotics administered in surgery are powders that must be reconstituted with sterile water or a sodium chloride solution (saline, 0.9% NaCl) to make an injectable solution. Other drugs, such as dantrolene (Dantrium), an infrequently used yet important skeletal muscle relaxant, also come in powder form and must be reconstituted before use (see Chapter 16). FIG. 1.7 A computer-automated drug dispensing system. Reproduced with permission from CareFusion. TABLE 1.5 Abbreviations for Drug Forms or Preparations Abbreviation Meaning cap Capsule gtts Drops soln Solution susp Suspension tab Tablet ung Ointment FIG. 1.8 Sterile powder must be reconstituted to form a solution that can be administered by injection. From Misch CE: Contemporary implant dentistry, ed 3, St Louis, 2008, Elsevier. Semisolids Semisolid preparations include creams, foams, gels, and ointments. Creams consist of active ingredients in a water base, whereas ointments contain active ingredients in an oil, lanolin, or petroleum base. Gels (such as K-Y Jelly) are thicker than creams but are still water based. Examples of semisolid drugs used in surgery include lidocaine (Xylocaine) jelly for topical anesthesia, Silvadene cream 1% for burns, estrogen cream for vaginal packing, and Neosporin ointment for wound dressing. Liquids Several types of liquid drug preparations are available. Many liquid medications are available in solution. A solution is a mixture of drug particles (called the solute), fully dissolved in a liquid medium (called the solvent, such as water or saline). Several common solutions are used from the sterile back table, including sodium chloride solution (saline, 0.9% NaCl) irrigation, antibiotic irrigation solutions, and heparin irrigation solution. Liquid nasal sprays, such as oxymetazoline (Afrin), may also be used from the sterile back table. Drugs may also be in suspension. A suspension is a form in which solid, undissolved particles float (are suspended) in a liquid. Suspensions should be shaken before administration to evenly distribute particles throughout the liquid. Cortisporin otic, used in ear surgery, is an example of a medication in suspension form used from the sterile back table. Tech Tip Just before handing a medication in suspension to the surgeon, simply roll the syringe or vial between your fingers or palms to mix the suspended particles evenly. Another type of liquid medication form is an emulsion, in which the medication is contained in a mixture of water and oil bound together with an emulsifier. An emulsifier is used to bind together two substances that do not normally mix. A household example of an emulsion is mayonnaise, in which lecithin (contained in egg yolks) binds egg yolks and oil together and keeps the substances from separating. Medication emulsions are usually either water in oil or oil in water, depending on the medication’s solubility. The most common emulsion used in surgery is propofol (Diprivan), an intravenous sedative-hypnotic agent used for induction of general anesthesia (see Chapter 15). Lecithin is also the emulsifier in propofol, thus it is contraindicated in patients with an allergy to eggs. Drugs in liquid form may also be administered orally, as elixirs (sweetened solutions of alcohol) or syrups (sweetened aqueous solutions); however, elixirs and syrups are rarely, if ever, used in surgery because of the NPO status for surgical patients. Gases The only common medication available in gas form is nitrous oxide, an inhalation anesthetic agent. Other inhalation anesthetic agents, such as desflurane (Suprane) (see Chapter 15), are known as volatile liquids. Volatile liquid agents are vaporized through an apparatus on the anesthesia machine into gas form for administration. Drug Administration Routes Medications are formulated for administration by a specific route. In addition to the drug form, the route by which a drug is given can affect onset time and body response. Drugs may be administered by several different routes, only a few of which are used commonly in surgery. There are two categories of medication administration routes: enteral and parenteral. The enteral route indicates that the medication is taken into the gastrointestinal (GI) tract, primarily by mouth (orally). The term parenteral indicates any route other than the digestive tract, the most common of which are topical, subcutaneous, intramuscular, and intravenous. Make It Simple Use medical terminology word-building techniques to help you understand the terms enteral and parenteral. The root word or combining form “enter/o-” means intestines, and the adjective ending “-al” means pertaining to. The combining form “/o” is not used when the suffix begins with a vowel, so the word enteral means pertaining to the intestines or intestinal tract. The prefix “para-” indicates beside or beyond (the “a” is dropped when the root word begins with a vowel), so the term parenteral means outside, or not within, the intestinal tract. The oral route (PO, from the Latin per os, “by mouth”) is the simplest and most common way to administer many drugs. Tablets or capsules are swallowed and readily absorbed through the lining (mucosa) of the GI tract. Certain drugs may irritate the GI mucosa and should therefore be given with food. Other drugs may be inactivated by increased amounts of digestive enzymes and so are best taken between meals. When drugs are administered orally, onset of action is usually slower and duration of effect is usually longer than with other routes. However, some drugs are completely inactivated by the digestive process and therefore must not be given orally. Insulin, a hormone, and heparin, an anticoagulant, are not effective when administered orally. The oral route is rarely used in surgery because the patient must be kept NPO before surgery and because many patients are under general anesthesia during surgery and so are unable to swallow. Standard medication orders, such as prescriptions and hospital orders, state the route of administration, usually in abbreviated form (Table 1.6, Fig. 1.9). The vast majority of medications used in surgery are administered parenterally. The most common parenteral routes are topical, subcutaneous, intramuscular, and intravenous. Some medications are available in topical preparations, which are intended for application to the skin or a mucous membrane–lined cavity. Some topical agents work at the site of application; this is called a local effect. Some topical medications exert a systemic effect, that is, throughout the entire body. Examples of topical medications with local effect include steroid creams for rashes and antibiotic ointment for cuts. Applied to the affected area, these agents work directly on the immediate site. Topical medications delivered through transdermal patches, such as those for hormone replacement therapy (estradiol), nicotine cessation (Nicoderm), and motion sickness (scopolamine), exert a systemic effect. Even though these types of agents are applied to an area of the skin, once absorbed the agents will have an effect on the entire body. Blood supply to the topical administration area impacts the speed of absorption. Topical medications are absorbed slowly through the skin but are absorbed rapidly when applied to blood supply–rich mucous membranes. NOTE Some medications, though taken orally, are actually topical in that the tablet (pill) is held in the mouth, either in the cheek (buccal) or under the tongue (sublingual), and allowed to dissolve. The medication is absorbed through the mucous membrane of the mouth and does not pass directly into the GI tract. TABLE 1.6 Abbreviations for Drug Administration Routes Abbreviation Meaning IM Intramuscularly IV Intravenously PO, po Orally (per os) Several topical medications are used in surgery. Antibiotic ointments, such as Neosporin, may be used on the surgical incision as part of the postoperative wound dressing. Topical hemostatic agents (see Chapter 9) are used almost exclusively in surgery. Agents, such as Avitene, may be applied to bleeding surfaces of the liver or spleen during trauma surgery to enhance coagulation. Topical antibiotic irrigation is common in surgery, in which case an antibiotic solution is poured or squirted into the surgical site (Fig. 1.10). In many vascular procedures, a solution of heparin (an anticoagulant; see Chapter 9) is used as a topical irrigation to help prevent the formation of blood clots in operative vessels during surgery. NOTE Vascular procedures may also involve using intravenous heparin for systemic effect. But when used as a topical irrigation, heparin exerts a more significant local effect because the operative vessel is usually clamped off, preventing systemic absorption of the agent. Instillation of a medication into a mucous membrane—lined cavity, such as the eye, nose, or urethra, may also be considered a topical route or application. A number of medications are instilled into body cavities intraoperatively, situations unique to surgery. For example, tetracaine (Pontocaine) drops may be instilled into the eye for local anesthesia for cataract extraction (see Chapter 10), oxymetazoline (Afrin) may be instilled into the nasal cavity for vasoconstriction during sinusoscopy, or lidocaine (Xylocaine) jelly may be instilled into the urethra as topical anesthesia for cystoscopy. During diagnostic gynecologic laparoscopy, a methylene blue solution may be instilled via a cervical cannula into the uterus. This procedure is known as a tubal dye study or chromotubation and is used to assess tubal patency in patients with infertility. Methylene blue solution fills the uterus and exits through the uterine tubes into the pelvic cavity when the tubes are patent (open) (Fig. 1.11). The laparoscope is used to observe the blue solution exiting the tubal fimbria. If the tubes are blocked by scarring, the solution cannot enter the pelvic cavity. Another example of medication instillation occurs during operative cholangiography. Although not as frequently performed currently because of the availability of endoscopic retrograde cholangiopancreatography, intraoperative cholangiography involves the instillation of radiopaque contrast media (ROCM) into the common bile duct to detect gallstones under x-ray. If present, common bile duct stones will be evident on radiograph as shadows in the ROCM. For additional information on diagnostic agents, see Chapter 6. FIG. 1.9 (A) Intramuscular injection (IM). (B) Intravenous injection (IV). From Workman ML: Understanding pharmacology, St Louis, 2011, Elsevier. FIG. 1.10 Antibiotic irrigation used during surgery is an example of a topical application of a medication. Inhalation is a means of medication administration that can be considered topical. Some asthmatic drugs are administered through a respiratory inhaler or through a nebulizer—a device that converts liquid drugs into an inhalable mist. The drug is absorbed in the bronchi of the lungs, providing local relief of bronchoconstriction. In surgery, anesthetic gases are administered via inhalation, but these drugs exert systemic rather than local effects. Subcutaneous injections are given beneath the skin into the subcutaneous tissue layer. Common sites for subcutaneous injections are the upper lateral aspect of the arm, the anterior thigh, and the abdomen. Depending on blood supply, absorption from subcutaneous tissue is fairly rapid. Only a few drugs are administered subcutaneously in surgery; for example, heparin may be injected subcutaneously, preoperatively, in cardiothoracic surgery, to help prevent pulmonary embolism when working near the great vessels. FIG. 1.11 Chromopertubation with solution of methylene blue dye indicates the patency of the proximal tubal portion. Note that in this photo, the uterine tube has been excised and the methylene blue solution is exiting the remaining tubal stump. From Falcone T: Clinical reproductive medicine and surgery, St Louis, 2007, Elsevier. Caution The Institute for Safe Medication Practices (ISMP) has identified that the abbreviations for subcutaneous administration, including “SC,” “SQ,” and “sub q” are frequently mistaken for other meanings. The ISMP recommends using “subcut” or “subcutaneously” to indicate this intended route of administration. A few drugs used in surgery are administered intramuscularly (IM). Intramuscular injections are usually given into a large muscle mass, such as the deltoid, gluteal, or vastus lateralis. Intramuscular absorption is usually rapid because of the large absorbing surface and good blood supply. An example of a drug given IM in surgery is ketorolac (Toradol), a nonsteroidal antiinflammatory drug used for postoperative pain relief. Probably the most common example of the parenteral route used at the surgical site is the administration (injection) of a local anesthetic agent for intraoperative pain control. Local anesthesia during a surgical procedure may be accomplished with an agent, such as lidocaine (Xylocaine), which is injected into multiple tissue layers as needed. Frequently, a longer-acting local anesthetic (such as bupivacaine) is used to inject the surgical site for postoperative pain control. For a more detailed discussion of local anesthetics, see Chapter 14. Most medications administered parenterally during surgery are given IV, that is, within a vein, as shown in Fig. 1.12. A small catheter is inserted into a vein and connected to tubing called an infusion set. The infusion set is attached to a bag of intravenous fluid for administration. Drugs may then be injected, as needed, into port sites located along the tubing. Drugs may be given all at once, as a bolus, or by slow infusion. Absorption is immediate for medications administered IV because the agent goes directly into the bloodstream. FIG. 1.12 A drug may be administered by intravenous injection. From Lilley LL: Pharmacology for Canadian health care practice, ed 2, St Louis, 2011, Elsevier. Other parenteral routes are used less frequently. Intradermal injections are given between layers of skin, as seen in tuberculin skin testing and allergy testing. A local anesthetic may be injected intradermally before placing an intravenous catheter, thereby reducing discomfort at the insertion site. Intraarticular injections (into the joint space) of antiinflammatory agents or local anesthetics may be given after arthroscopy. Intrathecal injection of an anesthetic agent is administered into the spinal subarachnoid space to diffuse into cerebral spinal fluid. During cardiac arrest resuscitation, a drug, such as epinephrine, may be injected directly into the heart; this is called an intracardiac injection. Pharmacokinetics The study of pharmacokinetics focuses on how the body processes drugs, and the science of pharmacodynamics examines how the action of the drug affects the body (Fig. 1.13). The science of pharmacokinetics studies a medication from administration through four basic physiological processes: absorption, distribution, biotransformation, and excretion (Table 1.7). The patient’s general health has a significant effect on how the body processes drugs. For example, if the patient’s blood supply, liver function, or kidney function is compromised, the ability to process medications is also compromised. Absorption To be effective, a drug must first be absorbed into the body. Absorption is the process by which a drug is taken into the body and moves from the site of administration into the blood. Drugs are absorbed from the site of administration into the bloodstream and enter systemic circulation. Speed of absorption, or absorption rate, varies by administration route and by blood supply to the area. Solubility of the drug (its ability to be dissolved) also affects the absorption rate. If a drug is in solid form, it must dissolve before it can be absorbed. Drugs in suspensions absorb faster than solid drugs, and solutions absorb faster than suspensions. For example, a solution instilled in the conjunctiva will absorb more quickly than an ointment applied to the conjunctiva. Oral absorption varies depending on the drug’s chemical structure as well as the pH (acidity) and motility of the GI tract. If the digestive tract is highly motile, as in patients with diarrhea, ingested drugs may not be adequately absorbed. Conversely, if the patient is constipated, drugs may be fully absorbed, sometimes to toxic levels. Intramuscular absorption is rapid if water-based drug solutions are injected and is slower if the solution is an oil-based emulsion. The amount and vascularization of muscle mass also affect the rate of absorption of medications given IM. Intravenous absorption is immediate because drugs are injected directly into the bloodstream. The absorption rate of drugs given subcutaneously depends on blood supply to the area of injection. NOTE Rapid drug absorption can be undesirable in surgery when local anes¬thetics are used. The anesthetic agent must stay in the desired area to exert its desired effect. Thus a vasoconstrictor, usually epinephrine, may be added to the anesthetic agent to narrow the small blood vessels in the local area and delay absorption of the anesthetic agent into systemic circulation. Local vasoconstriction will prolong the anesthetic agent’s effect (see Chapter 14). Absorption of drugs given by inhalation, especially inhalation anesthetics, is rapid because of the huge numbers of capillaries in the alveoli of the lungs. Some drugs administered by inhalation (such as steroids for asthma) are specifically formulated for local effect and so do not absorb rapidly. Mucosal tissues provide excellent absorption for some drugs because of the number of capillaries just under the mucosal surface. Common mucosal administration sites include the respiratory tract, oral cavity, and the conjunctiva. FIG. 1.13 Relationship between pharmacokinetics and pharmacodynamics. From Brenner GM, Stevens CW: Pharmacology, ed 4, St Louis, 2012, Elsevier. TABLE 1.7 Four Processes of Pharmacokinetics Four Processes of Pharmacokinetics Process Primary Body System Absorption Body system varies by administration route (e.g., integumentary, gastrointestinal, respiratory) Distribution Circulatory system Biotransformation Biliary system (liver) Excretion Urinary system (kidney) Although most drugs are not absorbed easily through skin, some are specifically formulated to overcome the skin barrier. Such drugs are administered transdermally from patches. For example, scopolamine patches are used to treat motion sickness; they release the drug slowly so it may be absorbed through skin over a period of hours. Thus absorption of a medication depends on the formulation of the drug, the route of administration, and the extent of blood supply to the site of administration. Distribution Once a drug has been absorbed into the bloodstream, the process called distribution begins. The circulatory system transports the drug throughout the body and drug molecules eventually diffuse out of the bloodstream to the site of action. The term bioavailability indicates the degree to which the drug molecule reaches the site of action to exert its effects. Several factors affect a drug’s bioavailability, including the acid-base balance (Insight 1.3). Because drug molecules are carried to all parts of the body, their effects can be seen in locations other than the intended area. The amount of drug reaching the site of action depends on the general condition of the patient’s circulatory system, on the effective blood flow to the intended area (tissue perfusion), and on the extent of plasma protein binding. Areas with high blood flow include the heart, liver, and kidneys, and tissues with low blood flow include bone, fat, and skin. For example, because bone has very limited blood flow, intravenous antibiotics often have little effect in treating bone infections. In addition, two barriers exist that limit the distribution of certain drug molecules to particular sites. The placental barrier allows some molecules to pass to the fetus, while restricting others. The blood-brain barrier also limits passage of certain molecules into brain tissue. Plasma Protein Binding Not all drug molecules in the bloodstream are available to bind at the site of action. Some drug molecules bind to proteins (albumins and globulins) contained in plasma—the liquid portion of blood—through a process known as plasma protein binding. Both the amount of plasma protein in the blood and the binding characteristics of the drug determine the extent to which a drug is bound. Some drugs are highly bound (up to 99%), some are only minimally bound, and others are not bound at all. Highly bound drug molecules have a longer duration of action because they stay in the body longer and a lower distribution to the site of action. The only drug molecules available to exert effects on the body are unbound molecules. Unbound drug molecules are considered bioactive. INSIGHT 1.3 Bioavailability and Acid-Base Balance A number of factors affect the bioavailability of a drug, including the size of the drug molecule (smaller molecules pass more easily through plasma membranes), lipid solubility (lipophilic molecules pass easier than hydrophilic molecules), and ionization of the drug molecule. Nonionized molecules pass through plasma membranes easier than ionized molecules. Acid-base balance also plays a role in drug bioavailability. An example of this concept in surgery occurs when a local anesthetic (a weak base) is injected into an infected wound (an acidic environment). The local anesthetic agent becomes ionized (a basic pH in an acidic condition) and cannot easily enter the lipid membrane of nerves to reach the site of action. If the agent does not readily enter the membrane, its intended effect may be delayed or reduced. Plasma protein binding is usually nonspecific; that is, plasma proteins can bind with many different drug molecules. It is also competitive in that drug molecules also compete with other drug molecules for protein-binding sites, significantly changing the amount of available drug. Potential hazards arise if patients are taking different drugs that compete for the same binding sites. For instance, if a patient taking warfarin sodium (Coumadin), a highly bound anticoagulant, takes aspirin, the aspirin will bind with the same plasma protein sites, making more warfarin available than is needed (Fig. 1.14). If more than the expected amount of warfarin is available, overmedication and excessive anticoagulation may occur. Highly bound drugs used in surgery include propofol (Diprivan), fentanyl, and diazepam. Plasma protein binding is reversible. When the concentration of unbound drug in blood is lowered, either by metabolism or by excretion, bound molecules are released from binding sites. The extent of plasma protein binding can prolong a drug’s effects and contribute to drug-drug interactions. Biotransformation The circulatory system also distributes drugs to the liver, the major structure of the biliary system. In the liver, the chemical composition of a drug is changed by a process called metabolism or biotransformation. The goal of biotransformation is to change lipid- soluble drug molecules into water-soluble molecules that can be more easily excreted. The liver is the primary site for biotransformation, but for some drug molecules, this process may take place in other locations, such as plasma, lungs, GI tract, or kidneys. Biliary biotransformation takes place when liver cells (hepatocytes) containing enzymes break down some drug molecules into other molecules called metabolites. The effectiveness of liver enzymes depends on several factors, including patient age, concurrent drug therapy, organ disease (e.g., cirrhosis), and nutritional status. Only unbound (bioactive) drug molecules can be biotransformed. Whereas some drugs are completely broken down and some are not broken down at all, most drugs are at least partially biotransformed. FIG. 1.14 Plasma protein binding of aspirin and warfarin. (A) Warfarin binds to specific receptor sites on plasma proteins. (B) Aspirin binds to the same receptor sites, making more warfarin available. Some drugs are actually prodrugs; this means they are administered in an inactive form, which is biotransformed into an active drug to produce the needed effect. Examples of prodrugs include the antiglaucoma agent dipivefrin (Propine, see Chapter 10) and the proton-pump inhibitor (see Chapter 13) omeprazole (Prilosec). Dipivefrin is also an example of a drug that is not broken down by the liver but at the site of administration (the eye). Dipivefrin is a prodrug that is biotransformed into epinephrine in the eye by enzyme hydrolysis. Hydrolysis is the addition of water to break chemical bonds of a larger molecule to form two smaller molecules (in this case into epinephrine and pivalic acid). All drugs taken orally enter the liver through the hepatic portal circulation (Fig. 1.15) before entering systemic circulation. The hepatic portal circulatory system is the venous drainage of the upper GI tract, carrying molecules absorbed by the gut into veins leading to the liver. Many drugs undergo a first-pass effect, which means they may be altered or nearly inactivated when passing through the liver, potentially reducing the drug’s effectiveness. Once liver enzymes begin to transform drug molecules, though, the enzymes are less able to break down additional amounts of the drug; thus repeated dosing may be used to overcome the first-pass effect. Drugs administered parenterally or sublingually are not subject to the first-pass effect. FIG. 1.15 Drugs administered orally are distributed to the liver for metabolism by the hepatic portal circulation. From Huether SE, McCance KL: Understanding pathophysiology, ed 5, St Louis, 2011, Elsevier. Excretion Medications taken into the body are eliminated in the process called excretion. Some drug molecules are eliminated in the bile, feces, or skin, but most unchanged drugs and metabolites are excreted by the kidneys and eliminated in urine (Fig. 1.16). Notable exceptions to urinary excretion are volatile anesthetic agents, which are excreted by the lungs. The circulatory system carries blood to the kidneys, where it is filtered and returned to general circulation. Two renal processes remove drug molecules and metabolites from the body: glomerular filtration and tubular secretion. Only drug molecules not bound to plasma proteins (i.e., bioactive molecules) will be filtered out of blood plasma reaching the glomerulus. How much unbound drug is filtered out depends on the glomerular filtration rate, which depends on blood pressure and blood flow to the kidneys. Tubular secretion uses cellular energy to force drugs and their metabolites from the bloodstream for elimination. Some drugs, depending on their characteristics and the pH of urine, may be reabsorbed and returned to circulation by tubular reabsorption. Factors influencing renal elimination of drug molecules include presence of kidney disease, such as renal failure, the pH of urine, and the concentration of drug molecules in the plasma. Pharmacodynamics As stated previously, pharmacodynamics is the study of how drugs exert their effects on the body, at both the molecular and physiological levels. The human body responds to different drugs in varying degrees and at various rates. Drugs may also have more than one effect on the body and this is taken into consideration when prescribing medications. Make It Simple Pharmacokinetics is the study of what the body does to drugs (how the body processes drugs). Pharmacodynamics is the study of what drugs do to the body (how drugs affect the body). Drugs must be able to reach the site of action and interact with cells to produce therapeutic effects. In most cases, drugs bind to specialized proteins, or receptors, on cell membranes. Some drugs are specific in action and some are nonspecific. Interaction with the target site produces the intended effect, whereas interaction with other cells may produce what are called side effects. Drug molecules with specific mechanisms interact with specific receptors sites on cell membranes. This specificity has been described by the lock-and-key analogy; the lock is the receptor site on the cell membrane (usually a protein complex), and the drug molecule is the key that fits in that specific lock. Very few drugs are exactly specific to a certain receptor; instead, most drug molecules will react with several types of receptors. FIG. 1.16 Most drugs are excreted by the kidneys. Drugs are removed in the nephrons (A) and eliminated by the kidneys (B). Agonists are drugs that bind to or have an affinity (attraction) for a receptor and cause a particular response (Fig. 1.17). This can be compared with the analogy of a key opening a lock. Natural agonists include neurotransmitters, such as acetylcholine, and hormones. Some drugs, such as succinylcholine, act as chemical agonists. Drugs that bind to a receptor and prevent a response are called antagonists (see Fig. 1.17). Antagonists are also called receptor blockers. Following the lock-and-key analogy, an antagonist can be thought of as a key that fits the lock but cannot open it (cause a response). Antagonists may be competitive, that is, bind to sites and prevent the agonist from reaching the site, or noncompetitive in that the antagonist alters the target site, preventing the agonist from causing the intended effect. Drug antagonism is responsible for many drug interactions, some of which are not desired. Many patients receive multiple drug therapy, so potential exists for several drug-drug interactions. Multiple drugs may cancel each other out or reduce each other’s effects. Vitamin K is given as an antidote for warfarin if the patient has been overanticoagulated because additional amounts of vitamin K in the body can overcome the effect of warfarin. An example of a drug that reduces the effect of another drug is the antibiotic amoxicillin. When it is given to a patient taking oral contraceptives, the effectiveness of the contraceptive may be reduced. A drug that enhances the effect of another drug is called a synergist. Some drug-drug interactions may cause a dramatic increase in the intended effect of the primary drug, as seen in aspirin-warfarin interactions. FIG. 1.17 Receptor site agonist and antagonist action. (A) Acetylcholine is a natural agonist. (B) Succinylcholine is a chemical agonist. (C) Nondepolarizing muscle relaxants act as antagonists. In addition to interacting with another drug, some drugs may also interact with vitamins, minerals, and/or herbal supplements. For example, the effect of tetracycline is compromised when it is taken with dairy products containing calcium. However, it is important to keep in mind that most drugs do not interact with foods or other drugs. Not all drug actions are receptor-type interactions. For example, antibiotics may interfere quite specifically with certain aspects of bacterial cell metabolism, such as penicillin inhibiting the formation of bacterial cell wall (see Chapter 5). Some drugs interact specifically with certain enzymes rather than receptor sites on a cell. A drug molecule may bind with a particular enzyme and either inhibit or stimulate the enzyme’s activity to produce the desired effect. An example of a nonspecific (i.e., not a drug-receptor complex) interaction is a drug molecule’s ability to change the chemical environment surrounding the target cell. For example, mannitol—an osmotic diuretic—increases the osmotic pressure of urine; this means it reduces the reabsorption of water and produces large amounts of dilute urine. Antacids, such as sodium bicarbonate, chemically neutralize acid in the stomach. Several terms are used to clarify aspects of pharmacodynamics, and the surgical technologist should become familiar with the common terms as applied to the surgical patient. The reason or purpose for giving a medication is called the indication, whereas reasons against giving a particular drug are called contraindications. For example, a particular antibiotic may be indicated for a bacterial infection but is contraindicated when the patient has a known hypersensitivity to that antibiotic. Some important terms should be understood regarding the timing of expected drug effects. The time between administration of a drug and the first appearance of effects is called the onset. A drug’s onset can be affected by the administration route, absorption rate, and efficiency of distribution to the site of action. The time between administration and maximum effect is called the time to peak effect. Steady state, or equilibrium, is achieved when the amount of drug entering the body is equal to the amount of drug being eliminated. The time between onset and disappearance of drug effects is called the duration. A drug’s duration of action, or drug half-life, refers to the length of time the drug concentration is in the therapeutic range. Some drugs have a very short duration of action, such as propofol (6–8 minutes), whereas other drugs have a long duration of action, such as warfarin (a single dose is active for 2–5 days). A number of terms are used to describe the body’s response or reaction to medications. A side effect is a predictable but unintended effect of a drug. Side effects are rarely serious but usually unavoidable. An example of a side effect is the drowsiness that often occurs when antihistamines are used. Adverse effects are undesired, potentially harmful side effects of drugs. Adverse effects include nausea and vomiting, drug toxicity, hypersensitivity, and idiosyncratic (unusual) reactions. Drug toxicity may be the result of accidental overdosing or failure of the body to process the drug properly, as seen in patients with kidney or liver dysfunction. In cases of drug toxicity, the primary effect of the drug may be exaggerated, such as excessive anticoagulation when taking warfarin (Coumadin). Some drugs may be particularly toxic (usually in high doses) to a specific organ, such as the liver, kidney, or even the ear. For example, some antibiotics are known to be ototoxic in high doses; that is, they have the potential to damage the hearing mechanism (see Chapter 5). Acetaminophen can cause hepatotoxicity, and ibuprofen can cause nephrotoxicity. Make It Simple Use medical terminology word-building techniques to help you learn the meaning of terms used in pharmacodynamics. The suffix “-toxic” means poisonous or damaging; the root word for the liver is “hepat/o”; a root word for kidney is “nephr/o”; and a root word for ear is “oto.” Thus hepatotoxic means damaging to the liver, nephrotoxic means damaging to the kidney, and ototoxic means damaging to the (inner) ear. In addition, the root word “idi/o” means “unknown,” indicating that the cause of idiosyncratic drug reactions (IDRs) is not known at this time. Drug hypersensitivity is an adverse effect resulting from previous exposure to the drug or a similar drug. A patient may become sensitized to a drug after one or more doses, then exhibit an allergic response on subsequent administration of the drug. An allergic response may be immediate, with symptoms ranging from mild to severe. Mild allergy symptoms include the appearance of raised patches on the skin (wheals) with itching, commonly known as hives (Fig. 1.18). A severe allergic reaction, called anaphylaxis, can result in swollen bronchial passages and possible circulatory collapse (see Chapter 16). Delayed allergic reactions can occur days or weeks after a drug is taken and can include fever and joint swelling. FIG. 1.18 Urticaria (hives). From Amar SM, Dreskin SC: Urticaria, Primary Care 35(1):141–157, 2008. When the exact mechanism of an adverse drug effect is not known, the term idiosyncratic drug reaction, (IDR) is used. IDRs are rare and unpredictable adverse effects of some drugs on individuals. Some IDRs are thought to be related to a type of allergic response, but many of these reactions are unique to specific patients and particular drugs. One type of IDR that may occur in surgery is malignant hyperthermia (see Chapter 16), which is a life-threatening response to certain drugs attributable to a genetic defect. The emerging science of pharmacogenetics (see Chapter 2) may someday help identify and prevent some idiosyncratic drug effects. In the fascinating science of pharmacodynamics, some specific mechanisms of drug actions are known, but much remains to be discovered regarding many physiological interactions involved in drug therapy. Key Concepts Knowledge of basic principles of pharmacology can provide the surgical technologist with a solid foundation on which to build an understanding of the many drugs used in surgery. Drug sources include natural sources, such as plants, animals, and minerals, as well as drugs developed in chemistry and molecular biology laboratories. Drug classifications are often helpful in identifying the purpose or use of a drug. Medication orders may be in various forms in surgery and must be interpreted precisely. Drugs come in several forms or preparations, and surgical technologists must be able to recognize the form needed for the intended purpose. Pharmacokinetics is the study of the four basic steps the body uses to process drugs: absorption, distribution, metabolism, and excretion. The study of how drugs exert their effects is called pharmacodynamics. Most drugs interact with receptors on target cell membranes to produce the desired effect.