Reinforced Concrete I PDF 2024-2025 AQA
Document Details
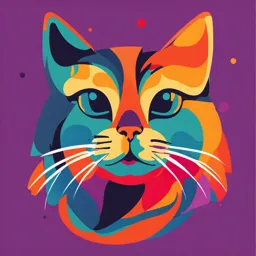
Uploaded by ArtisticMolybdenum
University of Halabja
2024
AQA
Dr. Nasih Habeeb Askandar
Tags
Related
- ED5001FP Reinforced Concrete Detailing and Design Training Notes 2015 PDF
- Fundamentals of Reinforced Concrete and Prestressed Concrete PDF
- Reinforced Concrete Design PDF
- 3A2 Structural Design Notes PDF
- CIVIL 3811 Lecture Slides - Week 3 - 2022 PDF
- Chapter 10: Bond, Development Length, Hooks, and Splicing of Reinforcement PDF
Summary
This document provides introduction and overview of reinforced concrete as a structural material. It discusses the historical background of reinforced concrete and its development. It also explains the advantages and disadvantages of reinforced concrete.
Full Transcript
University of Halabja/ Civil Eng. Dept. 3rd Stage Reinforced Concrete I Dr. Nasih H. Askandar Reinforced Concrete I 3rd YEAR CLASS (2024 – 2025) Chapter 1 Introduction and Overview...
University of Halabja/ Civil Eng. Dept. 3rd Stage Reinforced Concrete I Dr. Nasih H. Askandar Reinforced Concrete I 3rd YEAR CLASS (2024 – 2025) Chapter 1 Introduction and Overview Dr. Nasih Habeeb Askandar Ph.D. in Structural Engineering and Construction Materials 1 University of Halabja/ Civil Eng. Dept. 3rd Stage Reinforced Concrete I Dr. Nasih H. Askandar Ch. 1 / Introduction and Overview: 1-1 Concrete and Reinforced Concrete Concrete is a mixture of sand, gravel, crushed rock, or other aggregates held together in a rocklike mass with a paste of cement and water. Sometimes one or more admixtures are added to change certain characteristics of the concrete, such as its workability, durability, and time of hardening. As with most rocklike substances, concrete has high compressive strength and a very low tensile strength. Reinforced concrete is a combination of concrete and steel wherein the steel reinforcement provides the tensile strength lacking in the concrete. The steel reinforcing is also capable of resisting compression forces and is used in columns. 1-2 Historical Background: The real breakthrough for concrete occurred in 1824, when an English bricklayer named Joseph Aspdin, after long and laborious experiments, obtained a patent for cement that he called Portland cement because its color was quite similar to that of the stone quarried on the Isle of Portland off the English coast. He made his cement by taking certain quantities of clay and limestone, pulverizing them, burning them in his kitchen stove, and grinding the resulting clinker into a fine powder. During the early years after its development, his cement was used primarily in stuccos. The first uses of concrete are not very well known. Much of the early work was done by the Frenchmen Franc¸ois Le Brun, Joseph Lambot, and Joseph Monier. In 1832, Le Brun built a concrete house and followed it with the construction of a school and a church with the same material. In about 1850, Lambot built a concrete boat reinforced with a network of parallel wires or bars. Credit is usually given to Monier, however, for the invention of reinforced 2 University of Halabja/ Civil Eng. Dept. 3rd Stage Reinforced Concrete I Dr. Nasih H. Askandar concrete. In 1867, he received a patent for the construction of concrete basins or tubs and reservoirs reinforced with a mesh of iron wire. From 1867 to 1881, Monier received patents for reinforced concrete railroad ties, floor slabs, arches, footbridges, buildings. Another Frenchman, Franc¸ois Coignet, built simple reinforced concrete structures and developed basic methods of design. In 1861, he published a book in which he presented quite a few applications. He was the first person to realize that the addition of too much water to the mix greatly reduced concrete’s strength. William E. Ward built the first reinforced concrete building in the United States in Port Chester, New York, in 1875. In 1883, he presented a paper before the American Society of Mechanical Engineers in which he claimed that he got the idea of reinforced concrete by watching English laborers in 1867 trying to remove hardened cement from their iron tools. Thaddeus Hyatt, an American, was probably the first person to analyze the stresses in a reinforced concrete beam correctly, and in 1877, he published a 28- page book on the subject, entitled An Account of Some Experiments with Portland Cement Concrete, Combined with Iron as a Building Material. In this book, he praised the use of reinforced concrete and said that “rolled beams (steel) have to be taken largely on faith.” Hyatt put a great deal of emphasis on the high fire resistance of concrete. E. L. Ransome of San Francisco reportedly used reinforced concrete in the early 1870s and was the originator of deformed (or twisted) bars, for which he received a patent in 1884. These bars, which were square in cross section, were cold-twisted with one complete turn in a length of not more than 12 times the bar diameter. (The purpose of the twisting was to provide better bonding or adhesion of the concrete and the steel.) In 1890 in San Francisco, Ransome built the Leland Stanford Jr. Museum. It is a reinforced concrete building 95 m long, and 2 stories high in which 3 University of Halabja/ Civil Eng. Dept. 3rd Stage Reinforced Concrete I Dr. Nasih H. Askandar discarded wire rope from a cable-car system was used as tensile reinforcing. This building experienced little damage in the 1906 earthquake and the fire that ensued. The limited damage to this building and other concrete structures that withstood the great 1906 fire led to the widespread acceptance of this form of construction on the West Coast. Since the early 1900s, the development and use of reinforced concrete has been very rapid. 1-3 Advantages of Reinforced Concrete as a Structural Material The tremendous success of this universal construction material can be understood quite easily if its numerous advantages are considered. These include the following: 1. It has considerable compressive strength per unit cost compared with most other materials. 2. Reinforced concrete has great resistance to the actions of fire and water. 3. Reinforced concrete structures are very rigid. 4. It is a low-maintenance material. 5. It is usually the only economical material available for footings, floor slabs, basement walls, piers, and similar applications. 6. A special feature of concrete is its ability to be cast into an extraordinary variety of shapes from simple slabs, beams, and columns to great arches and shells. 7. In most areas, concrete takes advantage of inexpensive local materials (sand, gravel, and water) and requires relatively small amounts of cement and reinforcing steel, which may have to be shipped from other parts of the country. 1-4 Disadvantages of Reinforced Concrete as a Structural Material To use concrete successfully, the designer must be completely familiar with its weak points as well as its strong ones. Among its disadvantages are the following: 1. Concrete has a very low tensile strength, requiring the use of tensile reinforcing. 4 University of Halabja/ Civil Eng. Dept. 3rd Stage Reinforced Concrete I Dr. Nasih H. Askandar 2. Forms are required to hold the concrete in place until it hardens sufficiently. In addition, falsework or shoring may be necessary to keep the forms in place for roofs, walls, floors, and similar structures until the concrete members gain sufficient strength to support themselves. Formwork is very expensive. Its costs run from one-third to two-thirds of the total cost of a reinforced concrete structure. 3. The low strength per unit of weight of concrete leads to heavy members. This becomes an increasingly important matter for long-span structures. 4. The properties of concrete vary widely because of variations in its proportioning and mixing. Furthermore, the placing and curing of concrete are not as carefully controlled. 5. Two other characteristics that can cause problems are concrete’s shrinkage and creep. 1-5 Types of Portland Cement Concretes made with normal portland cement require about 2 weeks to achieve sufficient strength to permit the removal of forms and the application of moderate loads. Such concretes reach their design strengths after about 28 days and continue to gain strength at a slower rate after that. Type I—The common, Normal Portland Cement (NPC) all-purpose cement used for general construction work. Type II—A modified cement that has a lower heat of hydration than does Type I cement and that can withstand some exposure to sulfate attack. Type III-A high-early-strength cement This cement does have a much higher heat of hydration, enable us to obtain desired strengths in 3 to 7 days rather than the normal 28 days. These cement are particularly useful for the fabrication of precast members and for emergency repairs of concrete. 5 University of Halabja/ Civil Eng. Dept. 3rd Stage Reinforced Concrete I Dr. Nasih H. Askandar Type IV—A low-heat cement that produces concrete that generates heat very slowly. It is used for very large concrete structures. Type V—A cement used for concretes that are to be exposed to high concentrations of sulfate. 1-6 Admixtures Materials added to concrete during or before mixing are referred to as admixtures. They are used to improve the performance of concrete in certain situations as well as to lower its cost. Several of the most common types of admixtures are listed and briefly described here: Air-entraining admixtures, are used primarily to increase concrete’s resistance to freezing and thawing and provide better resistance to the deteriorating action of deicing salts. The air-entraining agents cause the mixing water to foam, with the result that billions of closely spaced air bubbles are incorporated into the concrete. The addition of accelerating admixtures, such as calcium chloride, to concrete will accelerate its early strength development. The results of such additions (particularly useful in cold climates Retarding admixtures are used to slow the setting of the concrete and to retard temperature increases. They consist of various acids or sugars, or sugar derivatives. Some concrete truck drivers keep sacks of sugar on hand to throw into the concrete in case they get caught in traffic jams or are otherwise delayed. Retarding admixtures are particularly Superplasticizers are admixtures made from organic sulfonates. Their use enables engineers to reduce the water content in concretes substantially while at the same time increasing their slumps. Although superplasticizers can also be used to keep water-cement ratios constant while using less cement, they are more commonly used to produce workable concretes with considerably higher strengths while using the same amount of cement. 6 University of Halabja/ Civil Eng. Dept. 3rd Stage Reinforced Concrete I Dr. Nasih H. Askandar Waterproofing materials usually are applied to hardened concrete surfaces, but they may be added to concrete mixes. These admixtures generally consist of some type of soap or petroleum products, as perhaps asphalt emulsions. 1-7 Properties of Concrete Thorough knowledge of the properties of concrete is necessary for the student before he or she begins to design reinforced concrete structures. An introduction to several of these properties is presented in this section. 1-7-1 Compressive Strength The compressive strength of concrete, f`c, is determined by testing to failure 28-day-old 150 mm in diameter by 300mm concrete cylinders at a specified loading rate. For the 28-day period, the cylinders are usually kept underwater or in a room with constant temperature and 100% humidity. Although concretes are available with 28-day ultimate strengths not less than 17.5MPa (ACI 19.2.1) up to as high as 70MPa to 140MPa, most of the concretes used fall into the 21MPa to 50MPa range. The values obtained for the compressive strength of concretes, as determined by testing, are to a considerable degree dependent on the sizes and shapes of the test units and the manner in which they are loaded. In many countries, the test specimens are cubes, 150 mm on each side. For the same batches of concrete, the testing of 150mm by 300mm cylinders provides compressive strengths only equal to about 80% of the values in MPa determined with the cubes. 7 University of Halabja/ Civil Eng. Dept. 3rd Stage Reinforced Concrete I Dr. Nasih H. Askandar Fig.1 Stress strain curve for different strength and failure cracks pattern. The stress-strain curves of Fig.1 - represent the results obtained from compression tests of sets of 28-day-old standard cylinders of varying strengths. You should carefully study these curves because they bring out several significant points: (a) The curves are roughly straight while the load is increased from zero to about one-third to one-half the concrete’s ultimate strength. (b) Beyond this range, the behavior of concrete is nonlinear. This lack of linearity of concrete stress-strain curves at higher stresses causes some problems in the structural analysis of concrete structures because their behavior is also nonlinear at higher stresses. (c) Of particular importance is the fact that regardless of strengths, all the concretes reach their ultimate strengths at strains of about 0.002. (d) Concrete does not have a definite yield strength; rather, the curves run smoothly on to the point of rupture at strains of from 0.003 to 0.004. It will be assumed for the purpose of future calculations in this text that concrete fails at 0.003 (ACI 22.2.2.1). (e) Many tests have clearly shown that stress-strain curves of concrete cylinders are almost identical to those for the compression sides of beams. 8 University of Halabja/ Civil Eng. Dept. 3rd Stage Reinforced Concrete I Dr. Nasih H. Askandar (f) It should be further noticed that the weaker grades of concrete are less brittle than the stronger ones—that is, they will take larger strains before breaking. 1-7-2 High-Strength Concretes Concretes with compression strengths exceeding 70MPa are referred to as high-strength concretes. Another name sometimes given to them is high- performance concretes because they have other excellent characteristics besides just high strengths. For instance, the low permeability of such concretes causes them to be quite durable as regards the various physical and chemical agents acting on them that may cause the material to deteriorate. For ordinary applications, 21MPa and 28MPa concrete are used, whereas, for prestressed construction, 35MPa and 60MPa strengths are common. For some applications, such as for the columns of the lower stories of high-rise buildings, concretes with strengths up to 63MPa or 70MPa have been used and can be furnished by ready-mix companies. As a result, the use of such high-strength concretes is becoming increasingly common. Furthermore, concretes have been produced with strengths higher than 140MPa and more. Perhaps these latter concretes should be called super-high-strength concretes or super-high- performance concretes. 1-7-3 Static Modulus of Elasticity Concrete has no clear-cut modulus of elasticity. Its value varies with different concrete strengths, concrete age, type of loading, and the characteristics and proportions of the cement and aggregates. Furthermore, there are several different definitions of the modulus: (a) The initial modulus is the slope of the stress-strain diagram at the origin of the curve. 9 University of Halabja/ Civil Eng. Dept. 3rd Stage Reinforced Concrete I Dr. Nasih H. Askandar (b) The tangent modulus is the slope of a tangent to the curve at some point along the curve—for instance, at 50% of the ultimate strength of the concrete. (c) The slope of a line drawn from the origin to a point on the curve somewhere between 25% and 50% of its ultimate compressive strength is referred to as a secant modulus. (d) Another modulus, called the apparent modulus or the long-term modulus, is determined by using the stresses and strains obtained after the load has been applied for a certain length of time. Section 19.2.2 of the ACI Code states that the following expression can be used for calculating the modulus of elasticity of concretes weighing from 1500kg/m3 to 2500kg/m3: 𝑬𝒄 = 𝒘𝟏.𝟓 𝒄 (𝟎. 𝟎𝟒𝟑)√𝒇`𝒄 In this expression, Ec is the modulus of elasticity in GPa, wc is the weight of the concrete in kg/m3, and f`c is its specified 28-day compressive strength in MPa. This is actually a secant modulus with the line (whose slope equals the modulus) drawn from the origin to a point on the stress–strain curve corresponding approximately to the stress (0.45f `c) that would occur under the estimated dead and live loads the structure must support. For normal-weight concrete weighing approximately 2400kg/m3, the ACI Code states that the following simplified version of the previous expression may be used to determine the modulus: 𝑬𝒄 = 𝟒𝟕𝟎𝟎√𝒇`𝒄 1-7-4 Poisson’s Ratio As a concrete cylinder is subjected to compressive loads, it not only shortens in length but also expands laterally. The ratio of this lateral expansion to the longitudinal shortening is referred to as Poisson’s ratio. Its value varies from about 0.11 for the higher-strength concretes to as high as 0.21 for the weaker-grade concretes, with average values of about 0.16. 10 University of Halabja/ Civil Eng. Dept. 3rd Stage Reinforced Concrete I Dr. Nasih H. Askandar 1-7-5 Shrinkage When the materials for concrete are mixed, the paste consisting of cement and water fills the voids between the aggregate and bonds the aggregate together. This mixture needs to be sufficiently workable or fluid so that it can be made to flow in between the reinforcing bars and all through the forms. To achieve this desired workability, considerably more water (perhaps twice as much) is used than is necessary for the cement and water to react (called hydration). After the concrete has been cured and begins to dry, the extra mixing water that was used begins to work its way out of the concrete to the surface, where it evaporates. As a result, the concrete shrinks and cracks. The resulting cracks may reduce the shear strength of the members and be detrimental to the appearance of the structure. In addition, the cracks may permit the reinforcing to be exposed to the atmosphere or chemicals, such as deicers, thereby increasing the possibility of corrosion. Shrinkage continues for many years, but under ordinary conditions, probably about 90% of it occurs during the first year. Furthermore, the larger the surface area of a member in proportion to its volume, the larger the rate of shrinkage; that is, members with small cross-sections shrink more proportionately than do those with large cross-sections. To minimize shrinkage it is desirable to: (1) keep the amount of mixing water to a minimum; (2) cure the concrete well; (3) place the concrete for walls, floors, and other large items in small sections (thus allowing some of the shrinkage to take place before the next section is placed); (4) use construction joints to control the position of cracks; (5) use shrinkage reinforcement; and (6) use appropriate dense and nonporous aggregates. 11 University of Halabja/ Civil Eng. Dept. 3rd Stage Reinforced Concrete I Dr. Nasih H. Askandar 1-7-6 Fatigue: Some reinforced concrete members, such as bridges, are subjected to a large number of loading cycles. In such case the member may fail in fatigue. Stress ratio (ratio of minimum applied stress to maximum applied stress) of 0.1. At 1 million cycles, the fatigue strength is generally between 50 and 70% of initial static strength and is relatively unaffected by realistic moisture and temperature exposures of concrete structures. 1-7-7 Creep Under sustained compressive loads, concrete will continue to deform for long periods of time. After the initial deformation occurs, the additional deformation is called creep, or plastic flow. If a compressive load is applied to a concrete member, an immediate or instantaneous elastic shortening occurs. If the load is left in place for a long time, the member will continue to shorten over a period of several years, and the final deformation will usually be two to three times the initial deformation. Perhaps 75% of the total creep will occur during the first year. Should the long- term load be removed, the member will recover most of its elastic strain and a little of its creep strain. If the load is replaced, both the elastic and creep strains will again develop. The amount of creep is largely dependent on the amount of stress. It is almost directly proportional to stress as long as the sustained stress is not greater than about one-half of f `c. Beyond this level, creep will increase rapidly. Long- term loads not only cause creep but also can adversely affect the strength of the concrete. Higher-strength concretes have less creep than do lower-strength concretes stressed at the same values. Creep increases with higher temperatures 12 University of Halabja/ Civil Eng. Dept. 3rd Stage Reinforced Concrete I Dr. Nasih H. Askandar The higher the humidity, the smaller will be the free pore water that can escape from the concrete. Creep is almost twice as large at 50% humidity than at 100% humidity Concretes with the highest percentage of cement–water paste has the highest creep because the paste, not the aggregate, does the creeping. Obviously, the addition of reinforcing to the compression areas of concrete will greatly reduce creep because steel exhibits very little creep at ordinary stresses. Large concrete members (i.e., those with large volume-to-surface area ratios) will creep proportionately less than smaller thin members where the free water has smaller distances to travel to escape. 1-7-8 Tensile Strength The tensile strength of concrete varies from about 8% to 15% of its compressive strength. A major reason for this small strength is the fact that concrete is filled with fine cracks. The cracks have little effect when concrete is subjected to compression loads because the loads cause the cracks to close and permit compression transfer. Obviously, this is not the case for tensile loads. Although tensile strength is normally neglected in design calculations, it is nevertheless an important property that affects the sizes and extent of the cracks that occur. Furthermore, the tensile strength of concrete members has a definite reduction effect on their deflections. (Because of the small tensile strength of concrete, little effort has been made to determine its tensile modulus of elasticity. Based on this limited information, however, it seems that its value is equal to its compression modulus.) This strength is quite difficult to measure with direct axial tension loads. As a result, two indirect tests have been developed to measure concrete’s tensile strength. These are the modulus of rupture and the split-cylinder tests. The tensile strength of concrete in flexure is quite important when considering beam cracks and deflections. For these considerations, the tensile 13 University of Halabja/ Civil Eng. Dept. 3rd Stage Reinforced Concrete I Dr. Nasih H. Askandar strengths obtained with the modulus of rupture test have long been used. The modulus of rupture (which is defined as the flexural tensile strength of concrete) is usually measured by loading a 150×150×750mm. plain (i.e., unreinforced) rectangular beam to failure with equal concentrated loads at its one-third points as per ASTM C78-2002.17 The load is increased until failure occurs by cracking on the tensile face of the beam. The modulus of rupture, fr, is then determined from the flexure formula. In the following expressions, b is the beam width, h is its depth, and M is PL/6, which is the maximum computed moment: 𝑴𝒄 𝟔𝑴 𝑷𝑳 𝒇𝒓 = = = 𝑰 𝒃𝒉𝟐 𝒃𝒉𝟐 The stress determined in this manner is not very accurate because, in using the flexure formula, we are assuming the concrete stresses vary in direct proportion to distances from the neutral axis. This assumption is not very good. Based on hundreds of tests, the code (Section 19.2.3) provides a modulus of rupture fr equal to 𝟎. 𝟔𝟐 𝝀√𝒇`𝒄. The λ term reduces the modulus of rupture when lightweight aggregates are used. The tensile strength of concrete may also be measured with the split- cylinder test. A cylinder is placed on its side in the testing machine, and a compressive load is applied uniformly along the length of the cylinder, with support supplied along the bottom for the cylinder’s full length (see Fig. -2). The cylinder will split in half from end to end when its tensile strength is reached. The tensile strength at which splitting occurs is referred to as the split-cylinder strength and can be calculated with the following expression, in which P is the maximum compressive force, L is the length, and D is the diameter of the cylinder: 14 University of Halabja/ Civil Eng. Dept. 3rd Stage Reinforced Concrete I Dr. Nasih H. Askandar 𝟐𝑷 𝒇𝒕 = 𝝅𝑳𝑫 Fig.2 In-direct test of tensile stress of concrete. 1-8 Reinforcing Steel The reinforcing used for concrete structures may be in the form of bars, welded wire fabric or strands. Reinforcing bars are referred to as plain or deformed. The deformed bars, which have ribbed projections rolled onto their surfaces to provide better bonding between the concrete and the steel, are used for almost all applications see Fig. 3. Reinforcing bars may be purchased in lengths 6m, 12m, and 18m. Longer bars have to be specially ordered. In general, longer bars are too flexible and difficult to handle. The ACI Table 20.2.2.4(a) has established an upper limit of 690MPa (100ksi) on yield strengths permitted for design calculations for reinforced concrete. Welded wire fabric is also frequently used for reinforcing slabs, pavements, and shells, and places where there is normally not sufficient room for providing 15 University of Halabja/ Civil Eng. Dept. 3rd Stage Reinforced Concrete I Dr. Nasih H. Askandar the necessary concrete cover required for regular reinforcing bars. The mesh is made of cold-drawn wires running in both directions and welded together at the points of intersection. The modulus of elasticity for nonprestressed steels is considered to be equal to200GPa ( 29 × 106 Psi). Fig.3 -Types of deformed reinforced bars. 1-8-1 Grades of Reinforcing Steel The SI concrete strengths (f `c ) and the minimum steel yield strengths (fy) are converted from the customary values into metric units and rounded off a bit. These values are used for the SI : 1. The bar sizes used in the metric version are numbered 10, 12, 16, 20, 22, 25, 30, 32, 35, 43,and 57 mm. For instance, the #3 bar has a diameter equal to 9.5 mm(to get the number take: 3 /8 inches times 25,4 mm), the #4 bar has a diameter equal to 12.7mm, and so on. 2. The steel reinforcing grades, or minimum steel yield strengths, referred to in the code are 280, 350, 420, 520, and 690MPa. These correspond, respectively, to Grade 40, 50, 60, 75, and 100ksi bars. See ACI Table 20.2.1.3(a) , (b) and (c) 3. The concrete strengths in metric units referred to in the code are 17, 21, 24, 28, 35, 42, …. MPa. These correspond respectively to 2500, 3000, 3500, 4000, 5000, 6000……..psi concretes. 16 University of Halabja/ Civil Eng. Dept. 3rd Stage Reinforced Concrete I Dr. Nasih H. Askandar 1-8-2 Corrosive Environments When reinforced concrete is subjected to deicing salts, seawater, or spray from these substances, it is necessary to provide special corrosion protection for the reinforcing. The structures usually involved are bridge decks, parking garages, wastewater treatment plants, and various coastal structures. Should the reinforcement be insufficiently protected, it will corrode; as it corrodes, the resulting oxides occupy a volume far greater than that of the original metal. The results are large outward pressures that can lead to severe cracking and spalling of the concrete. This reduces the concrete protection, or cover, for the steel, and corrosion accelerates. Also, the bond, or sticking of the concrete to the steel, is reduced. The result of all of these factors is a decided reduction in the life of the structure. 1-8-3 Compatibility of Concrete and Steel Concrete and steel reinforcing work together beautifully in reinforced concrete structures. The advantages of each material seem to compensate for the disadvantages of the other. 1. The most important point that used steel instead of other metals is that it have the same modulus of elasticity of concrete see Fig.4. The composite material concrete and steel work well together in relation to temperature changes because their coefficients of thermal expansion are quite close. For steel, the coefficient is 11.1x10-6mmper unit length per degree centigrade, and close to the concrete. 2. The two materials bond together very well, so there is little chance of slippage between the two; thus, they will act together as a unit in resisting forces. The excellent bond obtained is the result of the chemical adhesion between the two materials, the natural roughness of the bars, and the closely spaced rib-shaped deformations rolled onto the bars’ surfaces. 3. Steel has a high modulus of elasticity. 4. Low cost. 17 University of Halabja/ Civil Eng. Dept. 3rd Stage Reinforced Concrete I Dr. Nasih H. Askandar 1-9 Introduction to Loads Perhaps the most important and most difficult task faced by the structural designer is the accurate estimation of the loads that may be applied to a structure during its life. No loads that may reasonably be expected to occur may be overlooked. After loads are estimated, the next problem is to decide the worst possible combinations of these loads that might occur at one time. As will be seen, loads are classed as being dead, live, or environmental. To design a structure, it is necessary for the weights or dead loads of the various parts to be estimated for use in the analysis. 1-9-1 Dead Loads Dead loads are loads of constant magnitude that remain in one position. They include the weight of the structure under consideration as well as any fixtures that are permanently attached to it. For a reinforced concrete building, some dead loads are the frames, walls, floors, ceilings, stairways, roofs, and plumbing. The exact sizes and weights of the parts are not known until the structural analysis is made and the members of the structure are selected. The weights, as determined from the actual design, must be compared with the estimated weights. If large discrepancies are present, it will be necessary to repeat the analysis and design using better estimated weights. The approximate weights of some common materials used for floors, walls, roofs, and the like are given in Table 1. 18 University of Halabja/ Civil Eng. Dept. 3rd Stage Reinforced Concrete I Dr. Nasih H. Askandar 19 University of Halabja/ Civil Eng. Dept. 3rd Stage Reinforced Concrete I Dr. Nasih H. Askandar Fig. 4– Typical stress-strain curves for reinforced bars. Table- 1 Dead Load: Type of Dead Loads Unit weight (γ )kN/m3 - Brick walls 19 - Cement 14 - Gypsum 12 - Plain concrete 23 - Reinforced concrete 24 - Dry earth 16 - Hollow Block walls 14 - Cellular concrete block wall 8.0 - Perforated brick walls 13 - Sand 16-17 - Gravel 17-17 - Steel 78.5 - Thermostone 9.0 20 University of Halabja/ Civil Eng. Dept. 3rd Stage Reinforced Concrete I Dr. Nasih H. Askandar 1-9-2 Live Loads Live loads are loads that can change in magnitude and position. They include occupancy loads, warehouse materials, construction loads, overhead service cranes, equipment operating loads, and many others. In general, they are induced by gravity, act downward, and are distributed uniformly over an entire floor. Some typical floor live loads that act on building structures are presented in Table 1.2. Live load reductions are permitted, according to Section 4.8 of ASCE 7, because is it unlikely that the entire structure will be subjected to its full design live load over its entire floor area all at one time. Table1-2 , Minimum uniformly distributed live loads.(3) L.L. L.L. Occupancy or Use Occupancy or Use kN/m2 kN/m2 1.Residential : 4. Libraries: a. Multi-family house: -Private apartments 2.0 -Reading rooms 3.0 -Public rooms 5.0 -Stake rooms 7.5 -Corridors 3.0 5. Storage Warehouse: b. Dwellings: -Light 6.25 -First Floor 2.0 -Heavy 12.5 -Second floor and habitable attics 1.5 6. Stores: -Uninhabitable attics 1.0 Retail C. Hotels: -first floor , rooms 5.0 -Gust rooms 2.0 -Upper floors 3.75 -Public rooms( Dinner and 5.0 -Wholesale 6.25 restaurant) -Corridors serving public rooms 5.0 7. Side Walks, Vehicular drive 12.5 ways and yards subjecting to trucking -Public corridors 3.0 8. Stair , fire escape, exit ways. 5.0 -Private Corridors 2.0 9. Yards and Terraces, and 5.0 Pedestrians. 2. Schools: 10. Garage ( Passenger Cars ) 5.0 -Class rooms 2.0 11. Apartments –See Residential. -Corridors 5.0 12. Office building: 3. Hospital : -Office 2.5 -Operating rooms 3.0 -Lobbies 5.0 -Private rooms 2.0 13.Assembly Halls and others Places of Assembly: -Wards 2.0 -Fixed Seats 3.0 -Movable Seats 5.0 -Balcony( Exterior ) 5.0 21 University of Halabja/ Civil Eng. Dept. 3rd Stage Reinforced Concrete I Dr. Nasih H. Askandar Among the many other types of live loads are: Traffic loads for bridges: Bridges are subjected to series of concentrated loads of varying magnitude caused by groups of a truck or train wheels. Soil pressures: such as the exertion of lateral earth pressures on walls or upward pressures on foundations. Hydrostatic pressures: such as water pressure on dams, inertia forces of large bodies of water during earthquakes, and uplift pressures on tanks and basement structures. Blast loads: caused by explosions, sonic booms, and military weapons. 1-9-3 Environmental Loads Environmental loads are loads caused by the environment in which the structure is located. For buildings, they are caused by rain, snow, wind, temperature change, and earthquake. 1. Snow and ice. In the colder states, snow and ice loads are often quite important. 1m of snow is equivalent to approximately 960N/mm2. 2. Rain. Although snow loads are a more severe problem than rain loads for the usual roof, the situation may be reversed for flat roofs—particularly those in warmer climates. If water on a flat roof accumulates faster than it runs off, the result is called ponding because the increased load causes the roof to deflect into a dish shape that can hold more water, which causes greater deflections, and so on. This process continues until equilibrium is reached or until collapse occurs. 3. Wind. A survey of engineering literature for the past 150 years reveals many references to structural failures caused by wind. Perhaps the most infamous of these have been bridge failures such as those of the Tay Bridge in Scotland in 1879 (which caused the deaths of 75 persons) and the Tacoma Narrows Bridge (Tacoma, Washington) in 1940. There have also been some disastrous building 22 University of Halabja/ Civil Eng. Dept. 3rd Stage Reinforced Concrete I Dr. Nasih H. Askandar failures from wind during the same period, such as that of the Union Carbide Building in Toronto in 1958. 4. Seismic loads. Many areas of the world are in earthquake territory, and in those areas, it is necessary to consider seismic forces in design for all types of structures. Through the centuries, there have been catastrophic failures of buildings, bridges, and other structures during earthquakes. It has been estimated that as many as 50,000 people lost their lives in the 1988 earthquake in Armenia. The 2008 earthquake in Sichuan Province, China, caused 69,000 fatalities and another 18,000 missings. 1-9-4 Design Codes The code is a legally enforceable document. It is merely a statement of current good practice in reinforced concrete design. It is, however, written in the form of law so that various public bodies, such as city councils, can easily vote it into their local building codes, and then it becomes legally enforceable in that area. In this manner, the ACI Code has been incorporated into law by countless government organizations throughout the United States. The International Building Code (IBC), which was first published in 2000 by the International Code Council, has consolidated the three regional building codes (Building Officials and Code Administrators, International Conference of Building Officials, and Southern Building Code Congress International) into one national document. The IBC Code is updated every three years and refers to the most recent edition of ACI 318 for most of its provisions related to reinforced concrete design, with only a few modifications. The ACI 318 Code is also widely accepted in Canada and Mexico and has had a tremendous influence on the concrete codes of all countries throughout the world. As more knowledge is obtained pertaining to the behavior of reinforced concrete, the ACI revises its code. Other well-known reinforced concrete specifications are those of the American Association of State Highway and 23 University of Halabja/ Civil Eng. Dept. 3rd Stage Reinforced Concrete I Dr. Nasih H. Askandar Transportation Officials (AASHTO) and the American Railway Engineering Association (AREA). 1-9-5 Load Factors Load factors are numbers, almost always larger than 1.0, that are used to increase the estimated loads applied to structures. They are used for loads applied to all types of members, not just beams and slabs. The loads are increased to attempt to account for the uncertainties involved in estimating their magnitudes. How close can you estimate the largest wind or seismic loads that will ever be applied to the building that you are now occupying? How much uncertainty is present in your answer? You should note that the load factors for dead loads are much smaller than the ones used for live and environmental loads. Obviously, the reason is that we can estimate the magnitudes of dead loads much more accurately than we can the magnitudes of those other loads. Section 5.3 of the code presents the load factors and combinations that are to be used for reinforced concrete design. The required strength, U, or the load- carrying ability of a particular reinforced concrete member, must at least equal the largest value obtained by substituting into ACI Equations 5.3. The following equations conform to the requirements of the International Building Code (IBC) as well as to the values required by ASCE/SEI 7-10.2 In the preceding expressions, the following values are used: 24 University of Halabja/ Civil Eng. Dept. 3rd Stage Reinforced Concrete I Dr. Nasih H. Askandar U = the design or ultimate load the structure needs to be able to resist D = dead load L = live load Lr = roof live load S = snow load R = rain load W = wind load E = seismic or earthquake load effects The reader must realize that the sizes of the load factors do not vary in relation to the seriousness of failure. You may think that larger load factors should be used for hospitals or high-rise buildings than for cattle barns. Furthermore, the ACI load factors are minimum values, and designers are perfectly free to use larger factors as they desire. The magnitude of wind loads and seismic loads, however, reflects the importance of the structure. For example, in ASCE-7, a hospital must be designed for an earthquake load 50% larger than a comparable building with less serious consequences of failure. 1-10 Problems: 1. How the engineer test the tensile strength of concrete. 2. Explain the shrinkage in the concrete, and how the engineer can minimize the shrinkage of it. 3. Define high strength concrete, what is the benefit of high strength concrete. Draw relationship between stress and strain for different strength of concrete. 4. What is the compatibility between steel and concrete that make steel used instead of another metals (materials) to reinforced concrete. 5. What is modulus of elasticity, how many methods used to find it, which of them used to find modulus of elasticity of concrete and which of them for Steel. Clear your answer with figures. 6. What s the main points that make steel used in RC instead of other materials. 25 University of Halabja/ Civil Eng. Dept. 3rd Stage Reinforced Concrete I Dr. Nasih H. Askandar 7. What are the advantages of RC as a structural material. 8. What are the disadvantages of RC as a structural material. 9. What is creep, what factors influences the creep of concrete. 10. Explain the shrinkage in the concrete, and how the engineer can minimize the shrinkage of it. 11. Who take patent on inventing cement and When ? How make it? 12. Draw stress-strain curve for different strength of concrete starting from 31MPa to 120MPa, and write the main similar and difference between them. 13. Who got patent on inventing cement and When ? How make it? 14. Draw stress-strain curve for different strength of steel bars (for reinforcement) starting from 420MPa (60ksi) to 1860MPa (270ksi), and write the main similar and difference between them. 15. How invented cement (found), at about which year, how he made it (produced) and what is the event that leads to popularized the use (widespread). 16. What is the difference between normal strength concrete and high strength concrete. 17. How many methods to test tensile strength of concrete, explain briefly, then draw each one. 26