Cell Signaling - Chapter 16 PDF
Document Details
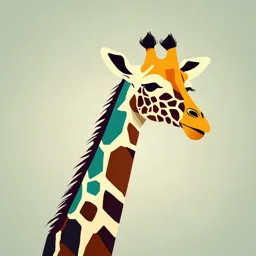
Uploaded by WellBalancedScandium1823
Tags
Summary
This document is a chapter on cell signaling, providing an overview of the general principles and some examples of signal molecules. The content explains how extracellular signals affect cellular behavior, and the different classes of cell-surface receptors.
Full Transcript
CHAPTER 16 Lecture Slides Cell Signaling Copyright © 2023 by W. W. Norton & Company, Inc. CHAPTER CONTENTS GENERAL PRINCIPLES OF CELL SIGNALING G-PROTEIN-COUPLED RECEPTORS ENZYME-COUPLED RECEPTORS Yeast cells respond to mating factor. Budding yeast (Saccharomyces cerevisiae) cells ar...
CHAPTER 16 Lecture Slides Cell Signaling Copyright © 2023 by W. W. Norton & Company, Inc. CHAPTER CONTENTS GENERAL PRINCIPLES OF CELL SIGNALING G-PROTEIN-COUPLED RECEPTORS ENZYME-COUPLED RECEPTORS Yeast cells respond to mating factor. Budding yeast (Saccharomyces cerevisiae) cells are (A) normally spherical, but (B) when they are exposed to an appropriate mating factor produced by neighboring yeast cells, they extend a protrusion toward the source of the factor. GENERAL PRINCIPLES OF CELL SIGNALING Signals Can Act over a Long or Short Range Extracellular Signals Act Through Specific Receptors to Change Cell Behaviors A Cell’s Response to a Signal Can Be Fast or Slow Cell-Surface Receptors Relay Extracellular Signals via Intracellular Signaling Pathways Some Intracellular Signaling Proteins Act as Molecular Switches Cell-Surface Receptors Fall into Three Main Classes Ion-Channel-coupled Receptors Convert Chemical Signals into Electrical Ones Signal transduction is the process whereby one type of signal is converted into another. (A) When a mobile telephone receives a radio signal, it converts it into a sound signal; when transmitting a signal, it does the reverse. (B) A target cell converts an extracellular signal into an intracellular signal. GENERAL PRINCIPLES OF CELL SIGNALING Signals Can Act over a Long or Short Range Animal cells use extracellular signal molecules to communicate with one another in various ways. (A) Hormones produced in endocrine glands are secreted into the bloodstream and are distributed widely throughout the body. (B) Paracrine signals are released by cells into the extracellular fluid in their neighborhood and act locally. (C) Neuronal signals are transmitted electrically along a nerve cell axon. When this electrical signal reaches the nerve terminal, it causes the release of neurotransmitters onto adjacent target cells. (D) In contact-dependent signaling, a cell-surface-bound signal molecule binds to a receptor protein on an adjacent cell. Many of the same types of signal TABLE 16–1 SOME EXAMPLES OF SIGNAL MOLECULES Signal Molecule Site of Origin Chemical Nature Some Actions Hormones Epinephrine (adrenaline) adrenal gland derivative of the amino acid tyrosine increases blood pressure, heart rate, an d metabolism Cortisol adrenal gland steroid (derivative of cholesterol) affects metabolism of proteins, carbohy drates, and lipids in most tissues Estradiol ovary steroid (derivative of cholesterol) induces and maintains secondary femal e sexual characteristics Insulin β cells of pancreas protein stimulates glucose uptake, protein synt hesis, and lipid synthesis in various cell types Testosterone testis steroid (derivative of cholesterol) induces and maintains secondary male sexual characteristics Thyroid hormone (thyroxine) thyroid gland derivative of the amino acid tyrosine stimulates metabolism in many cell typ es Ethylene Plant tissues Gaseous hydrocarbon Regulates developmental processes, including seed germination and fruit ripening Local Mediators Epidermal growth factor (EGF) various cells protein stimulates epidermal and many other c ell types to proliferate Platelet-derived growth factor (PDGF) various cells, including blood platelets protein stimulates many cell types to proliferat e Nerve growth factor (NGF) various innervated tissues protein promotes survival and axonal growth of certain classes of neurons Histamine mast cells derivative of the amino acid histidine causes blood vessels to dilate and beco me leaky, helping to cause inflammatio n Nitric oxide (NO) nerve cells; endothelial cells lining bloo dissolved gas causes smooth muscle cells to relax; re d vessels gulates nerve-cell activity Neurotransmitters Acetylcholine nerve terminals derivative of choline excitatory neurotransmitter at many ne rve– muscle synapses and in central nervous system γ-Aminobutyric acid (GABA) nerve terminals derivative of the amino acid glutamic a inhibitory neurotransmitter in central ne cid rvous system GENERAL PRINCIPLES OF CELL SIGNALING Extracellular Signals Act Through Specific Receptors to Change Cell Behaviors Extracellular signal molecules bind either to cell-surface receptors or to intracellular receptors. (A) Most extracellular signal molecules are large and hydrophilic and are therefore unable to cross the plasma membrane directly; instead, they bind to cell-surface receptors, which in turn generate one or more intracellular signaling molecules in the target cell. (B) Some small, hydrophobic, extracellular signal molecules pass through the target cell’s plasma The same signal molecule can induce different responses in different target cells. Different cell types are configured to respond to the neurotransmitter acetylcholine in different ways. Acetylcholine binds to similar receptor proteins on (A) heart pacemaker cells and (B) salivary gland cells, but it evokes different responses in each cell type. (C) Skeletal muscle cells produce a different type of receptor protein for the same signal molecule. (D) For such a versatile molecule, acetylcholine has a fairly simple chemical structure. An animal cell depends on multiple extracellular signals. Every cell type displays a set of receptor proteins that enables it to respond to a specific set of extracellular signal molecules produced by other cells. These signal molecules work in combinations to regulate the behavior of the cell. As shown here, cells may require multiple signals (blue arrows) to survive, additional signals (red arrows) to grow and divide, and still other signals (green arrows) to differentiate. If deprived of the necessary survival signals, most cells undergo a form of cell suicide known as apoptosis GENERAL PRINCIPLES OF CELL SIGNALING A Cell’s Response to a Signal Can Be Fast or Slow Extracellular signals can act slowly or rapidly. Certain types of cell responses—such as cell differentiation or increased cell growth and division involve changes in gene expression and the synthesis of new proteins; they therefore occur relatively slowly. Other responses—such as changes in cell movement, secretion, or metabolism—need not involve changes in gene expression and therefore occur more quickly GENERAL PRINCIPLES OF CELL SIGNALING Cell-Surface Receptors Relay Extracellular Signals via Intracellular Signaling Pathways Many extracellular signals activate intracellular signaling pathways to change the behavior of the target cell. A cell-surface receptor protein activates one or more intracellular signaling pathways, each mediated by a series of intracellular signaling molecules, which can be proteins or small messenger molecules. Signaling molecules eventually interact with specific effector proteins, altering them to change the behavior of the cell in various ways. Intracellular signaling proteins can relay, amplify, integrate, distribute, and modulate via feedback an incoming signal. In this example, a receptor protein located on the cell surface transduces an extracellular signal into an intracellular signal, which initiates one or more intracellular signaling pathways that relay the signal into the cell interior. Each pathway includes intracellular signaling proteins that can function in one of the various ways shown; some, for example, integrate signals from other intracellular signaling pathways. Many of the steps in the process can be modulated via feedback by other molecules or events in the cell. Note that some proteins in the pathway may be held in close proximity by a scaffold protein, which allows them to be activated at a specific location in the cell and with greater speed, efficiency, and selectivity. We review the production and function of small intracellular messenger molecules, more commonly called second messenger molecules Feedback regulation within an intracellular signaling pathway can adjust the response to an extracellular signal. (A) In this simple example, a downstream protein in a signaling pathway, protein Y, acts to increase the activity of the protein that activated it—a form of positive feedback. Positive feedback loops can ignite an explosive response, such as the activation of the proteins that trigger cell division. (B) In a simple example of negative feedback, protein Y inhibits the protein that activated it. Negative feedback loops can generate oscillations, similar to the way that populations of predators and prey can seesaw: an increase in prey (here, protein T) would promote the expansion of predators (protein Y); as the number of predators increases, the availability of prey will fall (via negative feedback), which will ultimately cause the predator population to decline. As the predators disappear, the prey populations will recover, providing GENERAL PRINCIPLES OF CELL SIGNALING Some Intracellular Signaling Proteins Act as Molecular Switches The activity of monomeric GTPases is controlled by two types of regulatory proteins. Guanine nucleotide exchange factors (GEFs) promote the exchange of GDP for GTP, thereby switching the protein on. GTPase-activating proteins (GAPs) stimulate the hydrolysis of GTP to GDP, thereby switching the protein off. GENERAL PRINCIPLES OF CELL SIGNALING Cell-Surface Receptors Fall into Three Main Classes Cell-surface receptors fall into one of three main classes. (A)An ion-channel-coupled receptor opens in response to binding an extracellular signal molecule. These channels are also called transmitter-gated ion channels. (B)When a G-protein-coupled receptor binds its extracellular signal molecule, the activated receptor signals to a trimeric G protein on the cytosolic side of the plasma membrane, which then turns on (or off) an enzyme (or an ion channel; not shown) in the same membrane. (C)When an enzyme-coupled receptor binds its extracellular signal molecule, an enzyme activity is switched on at the other end of the receptor, inside the cell. Many enzyme-coupled receptors have their own enzyme activity (left), while others rely on TABLE 16–2 SOME FOREIGN SUBSTANCES THAT ACT ON CELL–SURFACE RECEPTORS Substance Normal Signal Receptor Action Effect Barbiturates and benzodiazepines ( γ-aminobutyric acid (GABA) stimulate GABA-activated ion- relief of anxiety; sedation Valium and Ambien) channel-coupled receptors Nicotine acetylcholine stimulates acetylcholine- constriction of blood vessels; elevat activated ion-channel- ion of blood pressure coupled receptors Morphine and heroin endorphins and enkephalins stimulate G-protein- analgesia (relief of pain); euphoria coupled opiate receptors Curare acetylcholine blocks acetylcholine-activated ion- blockage of neuromuscular transmi channel-coupled receptors ssion, resulting in paralysis Strychnine glycine blocks glycine-activated ion- blockage of inhibitory synapses in s channel-coupled receptors pinal cord and brain, resulting in sei zures and muscle spasm Capsaicin heat stimulates temperature- induces painful, burning sensation; sensitive ion-channel- prolonged exposure paradoxically l coupled receptors eads to pain relief Menthol cold stimulates temperature- in moderate amounts, induces a co sensitive ion-channel- ol sensation; in higher doses, can c coupled receptors ause burning pain G-PROTEIN-COUPLED RECEPTORS Stimulation of GPCRs Activates G-Protein Subunits Some Bacterial Toxins Cause Disease by Altering the Activity of G Proteins Some G Proteins Directly Regulate Ion Channels Many G Proteins Activate Membrane-bound Enzymes That Produce Small Messenger Molecules The Cyclic AMP Signaling Pathway Can Activate Enzymes and Turn On Genes The Inositol Phospholipid Pathway Triggers a Rise in Intracellular Ca2+ G-PROTEIN-COUPLED RECEPTORS A Ca2+ Signal Triggers Many Biological Processes Some GPCR Signaling Pathways Generate a Dissolved Gas That Carries a Signal to Adjacent Cells GPCR-triggered Intracellular Signaling Pathways Can Achieve Astonishing Speed, Sensitivity, and Adaptability G-PROTEIN-COUPLED RECPTORS Largest family of cell surface receptors (>700 GPCRs in humans; >1000 involved in sense of smell in mice) diverse (mediate many pathways) Signals (proteins, peptide, amino acids, FAs All same structure (7 TM domains) G-PROTEIN-COUPLED RECEPTORS Stimulation of GPCRs Activates G-Protein Subunits Signal molecule binds GPCR - conformational change occurs and activates G protein located on the cytosolic side of PM G proteins all have 3 subunits (a, b, g) 2 tethered to PM by short lipid tail a subunit decreases affinity for GDP and exchanged for GTP Activation breaks ups G-protein subunits – a subunit/GTP detaches from bg complex and both interact with targets in PM Longer the target proteins remain bound to activated a and bg complexes, the more prolonged the signal A subunit has intrinsic GTPase activity (hydrolyze GTP to GDP) – back to inactive conformation An activated GPCR activates G proteins by encouraging the α subunit to release its GDP and pick up GTP. (A) In the unstimulated state, the receptor and the G protein are both inactive. Although they are shown here as separate entities in the plasma membrane, in some cases they are associated in a preformed complex. (B) Binding of an extracellular signal molecule to the receptor changes the conformation of the receptor, which in turn alters the conformation of the bound G protein. The alteration of the α subunit of the G protein allows it to exchange its GDP for GTP. This exchange triggers an additional conformational change that activates both the α subunit and the βγ complex, which dissociate to interact with their preferred target proteins in the plasma membrane. The receptor stays active as long as the external signal molecule is bound to it, and it can therefore activate many molecules of G protein. Note that both the α and γ subunits of the G protein have covalently attached lipid molecules (red) that help anchor the subunits to the plasma membrane. The G protein α subunit switches itself off by hydrolyzing its bound GTP to GDP. When an activated α subunit interacts with its target protein, it activates that target protein for as long as the two remain in contact. (In some cases, this interaction will inactivate the target protein; not shown.) The α subunit then hydrolyzes its bound GTP to GDP— an event that takes place usually within seconds of G- protein activation. The hydrolysis of GTP inactivates the α subunit, which dissociates from its target protein and—if the α subunit had separated from the βγ complex (as shown)—reassociates with a βγ complex to re- form an inactive G protein. The G protein is now ready to couple to another activated receptor, as in. Both the activated α subunit and the activated βγ complex can interact with target proteins in the plasma membrane G-PROTEIN-COUPLED RECEPTORS Some Bacterial Toxins Cause Disease by Altering the Activity of G Proteins Shut down a signal e.g. cholera toxin – modifies the a subunit of a G protein (Gs) that stimulates adenylyl cyclase Prevents Gs from hydolyzing bound GTP and locks G protein in an active state continuously stimulating adenylyl cyclase resulting in outflow of Cl- and water into gut (may lead to death) Whooping cough (pertussis toxin)- alters a subunit Gi that inhibits adenylyl cyclase locking in an inactive state stimulating coughing G-PROTEIN-COUPLED RECEPTORS Some G Proteins Directly Regulate Ion Channels ~20 different types of mammalian G proteins that are enzymes or ion channels in the PM Some directly regulate ion channels (e.g. heartbeat) Nerves that signal a slowdown in heartbeat release acetylcholine that binds GPCR on surface of heart pacemaker cells. bg complex bind the intracellular face of K+ channels, opening the channel and K+ moves out and slows the heart rate a subunit hydrolyzes GTP to GDP returning G protein to inactive state. A G protein directly couples receptor activation to the opening of K+ channels in the plasma membrane of heart pacemaker cells. (A)Binding of the neurotransmitter acetylcholine to its GPCR on heart cells results in the activation of the G protein, Gi. (B)The activated βγ complex directly opens a K+ channel in the plasma membrane, increasing its permeability to K+ and thereby making the membrane harder to activate and slowing the heart rate. Note, the activated α subunit of this G protein can inhibit the enzyme adenylyl cyclase (not shown), hence its designation as an inhibitory Gi. (C)Inactivation of the α subunit by hydrolysis of its bound GTP returns the G G-PROTEIN-COUPLED RECEPTORS Many G Proteins Activate Membrane-bound Enzymes That Produce Small Messenger Molecules Adenylyl cyclase – most frequent target enzyme for G proteins Produces cAMP and phospholipase C (generates inositol triphosphate and diacylglycerol) – second messengers Inositol phosphate promotes cytosolic accumulation of Ca2+ as a signaling molecule Extracellular ligand (first messenger) Enzymes activated by G proteins increase the concentrations of small intracellular signaling molecules. Because each activated enzyme generates many molecules of these second messengers, the signal is greatly amplified at this step in the pathway. The signal is relayed onward by the second messenger molecules, which bind to specific signaling proteins in the cell and influence their activity. G-PROTEIN-COUPLED RECEPTORS The Cyclic AMP Signaling Pathway Can Activate Enzymes and Turn On Genes Activated G protein a subunit switches on adenylyl cyclase causing an increase in cAMP from ATP cAMP phosophodiesterase converts cAMP to AMP caffeine is a stimulant that act by inhibition phosphodiesterase blocking cAMP degradation and cAMP messenger remains cAMP exerts is effect on cAMP-dependent protein kinase A (PKA) PKA normally inactive and is activated by cAMP and phosphorylates intracellular serine/threonine residues of proteins G-PROTEIN-COUPLED RECEPTORS Many cellular responses mediated by cAMP Epinephrine (adrenaline) binds adrenergic receptors (skeletal muscle and increases intracellular cAMP decreasing amount of glycogen by activating PKA that promotes glycogen breakdown maximizing glucose available for muscle activity / and FA breakdown to synthesize ATP Cyclic AMP is synthesized by adenylyl cyclase and degraded by cyclic AMP phosphodiesterase. Cyclic AMP (often abbreviated cAMP) is formed from ATP by a cyclization reaction that removes two phosphate groups from ATP and joins the remaining phosphate group to the sugar part of the AMP molecule. The degradation reaction breaks this new bond, forming AMP. The concentration of cyclic AMP rises rapidly in response to an extracellular signal. A nerve cell in culture responds to the binding of the neurotransmitter serotonin to a GPCR by synthesizing cyclic AMP. The concentration of intracellular cyclic AMP is monitored by injecting into the cell a fluorescent protein whose fluorescence changes when it binds cyclic AMP. Blue indicates a low level of cyclic AMP, yellow an intermediate level, and red a high level. (A) In the resting cell, the cyclic AMP concentration is about 5 × 10 –8 M. (B) Less than 1 minute after adding serotonin to the culture medium, the intracellular concentration of cyclic AMP has TABLE 16– 3 SOME CELL RESPONSES MEDIATED BY CYCLIC AMP Extracellular Signal M Target Tissue Major Response olecule* Epinephrine heart increase in heart rate and force of contract ion Epinephrine skeletal muscle glycogen breakdown Epinephrine, glucago fat fat breakdown n Adrenocorticotropic h adrenal gland cortisol secretion ormone (ACTH) *Although all of the signal molecules listed here are hormones, some responses to local mediators and to neurotransmitters are also medi ated by cyclic AMP. Epinephrine decreases glycogen levels in skeletal muscle cells. The hormone activates a GPCR, which turns on a G protein (Gs) that activates adenylyl cyclase to boost the production of cyclic AMP. The increase in cyclic AMP activates PKA, which phosphorylates and activates an enzyme called phosphorylase kinase. This kinase has two targets: it phosphorylates and thereby activates glycogen phosphorylase, the enzyme that breaks down glycogen. It also phosphorylates and thereby inactivates glycogen synthetase, the enzyme that makes glycogen. Because these reactions do not involve changes in gene transcription or new protein synthesis, they occur rapidly A rise in intracellular cyclic AMP can activate gene transcription. PKA, activated by a rise in intracellular cyclic AMP, can enter the nucleus and phosphorylate specific transcription regulators. Once phosphorylated, these proteins stimulate the transcription of a whole set of target genes. This type of signaling pathway controls many processes in cells, ranging from hormone synthesis in endocrine cells to the production of proteins involved in long-term memory in the brain. Activated PKA can also phosphorylate and thereby regulate other proteins and enzymes in the cytosol, G-PROTEIN-COUPLED RECEPTORS The Inositol Phospholipid Pathway Triggers a Rise in Intracellular Ca2+ GPCR activate membrane-bound phospholipase C (enzyme rather than second messenger) and work by activated Gq Phospholipase C propagates the signal by cleaving a lipid molecule in PM, called inositol phospholipid (phospholipid with the sugar inositol attached) Cleavage by phospholipase C generates two second messengers inositol 1,4,5-triphosphate (IP3) diacylglycerol (DAG) G-PROTEIN-COUPLED RECEPTORS IP3 is water soluble, binds Ca2+ channels in ER, Ca2+ rushes into cytosol and signals other proteins DAG is a lipid and remains in PM Recruits and activated protein kinase C (PKC) – needs Ca2+ to become active. Activated PKC phosphorylates intracellular proteins G-PROTEIN-COUPLED RECEPTORS A Ca2+ Signal Triggers Many Biological Processes Fertilization of an egg by a sperm triggers an increase in cytosolic Ca 2+ in the egg. This starfish egg was injected with a Ca2+-sensitive fluorescent dye before it was fertilized. When a sperm enters the egg, a wave of cytosolic Ca2+ (red)—released from the ER—sweeps across the egg from the site of sperm entry (arrow). This Ca2+ wave provokes a change in the egg surface, preventing entry of other sperm, and it also initiates embryonic development Calcium binding changes the shape of the calmodulin protein. (A) Calmodulin has a dumbbell shape, with two globular ends connected by a long α helix. Each of the globular ends has two Ca2+-binding sites. (B) Simplified representation of the structure, showing the conformational changes that occur when Ca2+-bound calmodulin interacts with an isolated segment of a target protein (red). In this conformation, the α helix jackknifes to surround the target. G-PROTEIN-COUPLED RECEPTORS Some GPCR Signaling Pathways Generate a Dissolved Gas That Carries a Signal to Adjacent Cells Nitric oxide (NO) is produced by activated GPCR NO diffuses to neighboring cells and converts NO into nitrate and nitrites One key target by NO is guanylyl cyclase (catalyzes the production of of cyclic GMP from GTP) Nitric oxide (NO) triggers smooth muscle relaxation in a blood-vessel wall. (A) Simplified drawing showing a cross section of a blood vessel with endothelial cells lining its lumen and smooth muscle cells surrounding the outside of the vessel. (B) The neurotransmitter acetylcholine causes the blood vessel to dilate by binding to a GPCR on the surface of the endothelial cells, thereby activating a G protein, Gq, to trigger Ca2+ release. Ca2+ activates nitric oxide synthase, stimulating the production of NO. NO then diffuses out of the endothelial cells and into adjacent smooth muscle cells, where it regulates the activity of specific proteins, causing the muscle cells to relax. One key target protein that can be activated by NO in smooth muscle cells is guanylyl cyclase, which catalyzes the production of cyclic GMP from GTP. Note that NO gas is highly toxic when inhaled and should not be confused with nitrous oxide (N2O), also known as laughing gas, which is sometimes used as a sedative. G-PROTEIN-COUPLED RECEPTORS GPCR-triggered Intracellular Signaling Pathways Can Achieve Astonishing Speed, Sensitivity, and Adaptability GPCR arose early in evolution as a basic need to respond to the environment (taste, smell, see) Speed achieved by intracellular signaling cascade e.g. rod photoreceptor in the eye (noncolor vision) – light is sensed by rhodopsin, a GPCR Rhodopsin stimulated by light activates a G protein (transducing) The activated a subunit activates an intracellular signaling cascade that causes action channels to close the plasma membrane of the photoreceptor, lower neurotransmitter release and sends impulse to the brain. A rod photoreceptor cell from the retina is exquisitely sensitive to light. The light-absorbing rhodopsin proteins are embedded in many pancake-shaped vesicles (discs) of membrane inside the outer segment of the photoreceptor cell. When the rod cell is stimulated by light, a signal is relayed from the rhodopsin molecules in the discs, through the cytosol, to cation channels in the plasma membrane of the outer segment. These cation channels close in response to the cytosolic signal, producing a change in the membrane potential of the rod cell. By mechanisms similar to those that control neurotransmitter release in ordinary nerve cells, the change in membrane potential alters the rate of neurotransmitter release from the synaptic region of the cell. Released neurotransmitters act on retinal nerve cells that pass the signal on to the brain. The light-induced signaling cascade in rod photoreceptor cells greatly amplifies the light signal. When rod photoreceptors are adapted for dim light, the signal amplification is enormous. This light-sensing cascade functions as follows. In the absence of a light signal, the second messenger molecule cyclic GMP is continuously produced by guanylyl cyclase in the cytosol of the photoreceptor cell. The cyclic GMP then binds to cation channels in the photoreceptor cell plasma membrane, keeping them open. Activation of rhodopsin by light triggers the activation of the α subunit of a G protein called transducin. Transducin turns on an enzyme called cyclic GMP phosphodiesterase, which breaks down cyclic GMP to GMP (much as cyclic AMP phosphodiesterase breaks down cyclic AMP.The sharp fall in the cytosolic concentration of cyclic GMP reduces the amount of cyclic GMP bound to the cation channels, which therefore close. Closing these channels decreases the influx of Na+, thereby altering the voltage gradient (membrane potential) across the plasma membrane and, ultimately, the rate of neurotransmitter release. The red arrows indicate the steps at which amplification occurs, with the thickness of the arrow roughly indicating the magnitude of the amplification. ENZYME-COUPLED RECEPTORS Activated RTKs Recruit a Complex of Intracellular Signaling Proteins Most RTKs Activate the Monomeric GTPase Ras RTKs Activate Phosphoinositide 3-Kinase to Produce Lipid Docking Sites in the Plasma Membrane Some Receptors Activate a Fast Track to the Nucleus Some Extracellular Signal Molecules Cross the Plasma Membrane and Bind to Intracellular Receptors ENZYME-COUPLED RECEPTORS Plants Make Use of Receptors and Signaling Strategies That Differ from Those Used by Animals Protein Kinase Networks Integrate Information to Control Complex Cell Behaviors ENZYME-COUPLED RECEPTORS Generally single transmembrane proteins - cytoplasmic domain has enzymatic activity Act a low conc (10-9 M to 10-11 M), slower compared to GPCR Receptor tyrosine kinases (RTKs) -largest class of enzyme coupled receptors Activated RTKs Recruit a Complex of Intracellular Signaling Proteins Activation of an RTK stimulates the assembly of an intracellular signaling complex. Typically, the binding of a signal molecule to the extracellular domain of an RTK causes two receptor molecules to associate into a dimer. The signal molecule shown here is itself a dimer and thus can physically cross-link two receptor molecules; other signal molecules induce a conformational change in the RTKs, causing the receptors to dimerize (not shown). In either case, dimer formation brings the kinase domain of each cytosolic receptor tail into contact with the other; this activates the kinases to phosphorylate several tyrosines on the adjacent receptor tail. Each phosphorylated tyrosine serves as a specific docking site for a different intracellular signaling protein, which then helps relay the signal to the cell’s interior; these proteins contain a specialized interaction domain— Intracellular signaling proteins interact to form a cross-linked, gel-like matrix at the plasma membrane. Adaptor proteins contain multiple interaction domains located within flexible, unstructured regions; some (such as SH2 domains) bind to phosphorylated tyrosines on the tails of activated RTKs, while others recognize the proline-rich interaction domains (dark blue) of different adapter proteins (blue). These multivalent interactions drive the assembly of complex, cross-linked, three- dimensional signaling networks. ENZYME-COUPLED RECEPTORS Most RTKs Activate the Monomeric GTPase Ras Ras – small GTP-binding protein attached by lipid tail to cytosolic face of PM Ras resembles the a subunit of a G protein Ras is active when GTP bound / inactive when GDP bound Ras GEF encourages Ras to exchange GDP for GTP RAS switched off by Ras GAP (hydrolyzed GTP to GDP) Ras activates a MAP-kinase signaling module. The Ras protein activates a three- kinase signaling module, which relays the signal onward. The final kinase in the module, MAP kinase, phosphorylates various downstream signaling or effector proteins. Stimulate cellular proliferation, differentiation, survival, ENZYME-COUPLED RECEPTORS RTKs Activate Phosphoinositide 3-Kinase to Produce Lipid Docking Sites in the Plasma Membrane Many extracellular signaling molecules that stimulate animals to grow and divide, include insulin-like growth factor (IGF) through RTKs that involve phosphoinositide 3-kinase (PI3 kinase) - phosphorylates inositol phospholipids in PM Some RTKs activate the PI-3-kinase–Akt signaling pathway. An extracellular survival signal, such as IGF, activates an RTK, which recruits and activates PI 3- kinase. PI 3-kinase then phosphorylates an inositol phospholipid that is embedded in the cytosolic side of the plasma membrane. The resulting phosphorylated inositol phospholipid attracts intracellular signaling proteins One of these that proteins, Akt, is a protein kinase that is activated at the membrane by signaling have a special domain phosphorylation mediated by two other protein kinases (here called protein kinases 1 and that recognizes 2); protein it. 1 is also recruited by the phosphorylated lipid docking sites. Once activated, Akt is kinase released from the plasma membrane and phosphorylates various downstream proteins on specific serines and threonines Activation of Akt promotes cell survival. One way Akt promotes cell survival is by phosphorylating and inactivating a protein called Bad. In its unphosphorylated state, Bad promotes apoptosis (a form of cell death) by binding to and inhibiting a protein, called Bcl2, which otherwise suppresses apoptosis. When Bad is phosphorylated by Akt, Bad releases Bcl2, which now blocks apoptosis, thereby promoting cell survival. Akt stimulates cells to grow in size by activating the serine/threonine kinase Tor. The binding of a growth factor to an RTK activates the PI-3-kinase–Akt signaling pathway (as shown in Figure 16–33). Akt then indirectly activates Tor by phosphorylating and inhibiting a protein that helps to keep Tor shut down (not shown). Tor stimulates protein synthesis and inhibits protein degradation by phosphorylating key proteins in these processes (not shown). The anticancer drug rapamycin slows cell growth by inhibiting Tor. In fact, the Tor protein derives its name from the fact that it is a target of rapamycin. HOW WE KNOW Untangling Cell Signaling Pathways ENZYME-COUPLED RECEPTORS Some Receptors Activate a Fast Track to the Nucleus ENZYME-COUPLED RECEPTORS Some Extracellular Signal Molecules Cross the Plasma Membrane and Bind to Intracellular Receptors ENZYME-COUPLED RECEPTORS Plants Make Use of Receptors and Signaling Strategies That Differ from Those Used by Animals W.W. Norton & Company was unable to obtain permission from the rightsholder to provide this figure. To obtain a file of this figure for classroom use, we suggest you contact the rightsholder credited in the figure caption. W.W. Norton & Company was unable to obtain permission from the rightsholder to provide this figure. To obtain a file of this figure for classroom use, we suggest you contact the rightsholder credited in the figure caption. ENZYME-COUPLED RECEPTORS Protein Kinase Networks Integrate Information to Control Complex Cell Behaviors Art Slides This concludes the Slide Set for Chapter 16 Essential Cell Biology, Sixth Edition Copyright © 2023 by W. W. Norton & Company, Inc.