Climate Change Risk PDF
Document Details
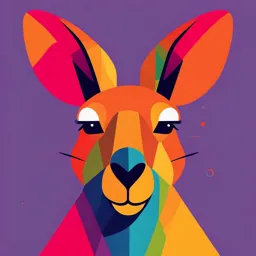
Uploaded by EasedOrangutan
Université du Québec en Abitibi-Témiscamingue (UQAT)
Tags
Related
- Ch04_SCR 2022 Sustainability and Climate Risk Exam PDF
- Climate Models and Scenario Analysis PDF
- NABARD Climate Action & Sustainability PDF
- Climate Change Vulnerability of Rice Farmers in Vietnam (PDF)
- Five Reasons Investors Should Expect the Unexpected PDF
- Unit 10: Insurance - Impact Underwriting PDF
Summary
This document explores climate change risk, classifying it into physical and transition risks. It covers learning objectives, data challenges, and examples of indirect risks. The document also delves into uncertainty of hazard model predictions and the impact on real estate and electricity sectors.
Full Transcript
Climate Change Risk Mf Learning Objectives After completing this reading you should be able to: @ Describe how climate risk can translate to financial risk. © Identify TCFD disclosure parameters and key recommendation features. © Identify data challenges modeling direct physical risk. Understand the...
Climate Change Risk Mf Learning Objectives After completing this reading you should be able to: @ Describe how climate risk can translate to financial risk. © Identify TCFD disclosure parameters and key recommendation features. © Identify data challenges modeling direct physical risk. Understand the current state of climate hazard data availability and what issues asset owners must consider when interpreting climate risk data. © Define and provide examples of indirect risks. © Differentiate physical and transition risks. © Understand how hazards/drivers, exposure, and © Discuss how physical and transition risks can provide opportunities for companies and sectors. vulnerability interact to manifest physical and transition risks and provide examples of each. © Identify the drivers of transition risk. Categorize transition risks (e.g., technology, market) and provide © Define stranded assets and discuss how different ‘examples of each. sectors may experience stranded asset risk. © Define and differentiate acute and chronic hazards. Provide examples. @ Understand current industry trends for each transition risk category and strategies companies can take to reduce risk or manifest climate-related opportunities. © Discuss uncertainty, variability, and accuracy in hazard © Understand how physical and transition risks uniquely model predictions (e.g., frequency, timeframes). affect the real estate and electricity sectors. 45 * Physical risks can have strong direct effects, but also This chapter provides a comprehensive introduction indirect ones through supply chains, legal liability, or sys- to the financial risks linked to climate change that throughout this text are referred to simply as climate risk. It explains the two main subtypes of climate. temic and second-order effects. * change targets, which is a source of transition risk, is risk: physical risk (resulting from the physical weather impacts of climate change) and transition risk (result- ing from the economic transformation to a net-zero huge and unprecedented in speed and scale. * and expand on, the topic by explaining how financial institutions can measure and manage climate risk (Chapter 6) and how climate modeling is carried out, including through scenario analysis (Chapter 7). Transition risks manifest not only through policies but also from technological change, reputational risk, and carbon economy), delving into the causes and implications of each type. Chapters 6 and 7 return to, The economic transformation required to reach climate market risk. * Climate risk transmits into the economy through corpo- rate and financial balance sheets and consumer spending patterns; however, the effects vary significantly by sector. Chapter Outline CLIMATE CHANGE 3.1 Introduction to Climate Risk 3.1 Introduction to Climate Risk 3.2 Types of Climate Risk 3.3 Physical Risks Climate-related financial risks, referred to throughout this ‘text as simply climate risk, are the financial risks linked to 3.4 Transition Risks, Opportunities, and Constraints 3.5 Transmission into Finance, the Economy, and Key Sectors Key Learning Points © Climate risk can be caused by the physical effects and changing weather patterns that result from climate * 46 Anthropogenic climate change is a huge subject that involves intense and multi-disciplinary scientific inquiry, inspires passionate political and social movements, and raises as many political, social, and moral questions as it does economic and financial ones. Science shows that it is eliminate the greenhouse gas emissions that cause it— transition risk. dioxide (CO,) and other greenhouse gases, such as methane, Climate risk results from an interaction of hazards or driv- indisputable that human-caused emissions of carbon are warming and disrupting the climate. Some arguments persist over the most appropriate means and timeline for Environmentally unsustainable or otherwise climateaffected assets can suffer from unanticipated or prema- reducing and/or eliminating emissions to mitigate climate change. However, increasing numbers of governments are converging on a goal of net-zero emissions by 2050. This ture write-offs due to either physical or transition risk, is in line with recommendations by the Intergovernmental thus becoming stranded assets. © climate change. Climate risk can affect financial balance sheets and lead to losses through standard channels such as diminished asset valuations or increased loan defaults. change—physical risk—or by efforts to reduce and ing factors with exposure and vulnerability. * RISK There is significant variability between hazards as to the accuracy with which historic data and climate models can Panel on Climate Change (IPCC), and the goals of the Paris Agreement in 2015 to keep the average global tempera- ture rise “well below 2°C above pre-industrial levels” and provide precise estimates. Global average temperatures are near certain to increase, but uncertainty persists on to “pursue efforts” to limit the rise to 1.5°C (UNFCCC, precipitation patterns. to address and adapt to the physical impacts of climate © Sustainability and Climate Risk Exam 2015). One area where debates still rage is over how best change, including what entities (companies, rich countries, etc.) should bear the brunt of the cost. and non-financial corporations. The TCFD’s outputs have Although the notion of climate risk is clearly linked to been particularly influential. It is best known for its final largely of private-sector representatives from both financial broader discussions around the phenomenon of climate report from 2017 (see below), but it has also produced change, and to international goals such as those of the Paris Agreement, climate risk is a distinct subset of these discus- This chapter largely follows TCFD categorizations, notably sions. Understanding the risks resulting from climate change does not require absolute certainty from a climate model, or conclusively assigning moral blame, but rather a focus on understanding how physical climate hazards or drivers of economic low-carbon transition affect firms and financial outcomes. other documents and technical annexes before and since. with regard to the subtypes of transition risk. Viewing climate through a risk lens means zeroing in specifically on the ways in which climate change affects assets, through hazards and drivers, exposures and vulnerabilities (Sections 3.2-3.4); how they transmit into the real and finan- cial economies affecting households, governments, compa- The source of the notion of climate risk is therefore somewhat distinct from wider climate discussions. While these nies, and the financial institutions that lend to and invest in them (Section 3.5); and how different sectors are differen- wider discussions are carried out through the United Nations Framework Convention on Climate Change, and tially affected. periodic intergovernmental summits, such as the one that resulted in the Paris Agreement, the notion and analysis of 3.2 Types of Climate Risk climate risk comes largely from a combination of financial Climate risk is usually divided into two broad categories: regulators and private-sector institutions. A good example is the Taskforce on Climate-Related Financial Disclosures (TCFD), formed in 2015 under the aegis of the G20 Finan- cial Stability Board. This international body is chaired by Michael Bloomberg, an entrepreneur, and is composed physical risk and transition risk. Physical risks (see Section 3.3) arise from the physical climate (and weather) impacts that result from the changing climate, whereas transition risks arise from the economic transformation and any dislocation needed to drastically reduce, and eventually TCFD FRAMEWORK AND RECOMMENDATIONS: FINAL REPORT (2017) The TCFD singles out physical and transition risk as the governance structures the firm has in place to address main sources of climate risk. It splits physical risk in two and take responsibility for climate issues, including at subtypes: acute and chronic. It splits transition risk into four subtypes: policy and legal, technology, market, and reputational. TCFD recommendations state that firms should disclose on four key parameters, ranging from the concrete to the abstract, so that their shareholders and other stake- holders are aware and fully informed of the progress the firms have made in their preparedness to tackle climate change. These are metrics and targets, that is, the metrics used by the firm in question to assess climate-related risks and opportunities; risk management, that is, what processes the firm has in place to manage climate risks; the firm’s strategy surrounding climate change; and the board level. Key features of the recommendations are that they are intended to be © adoptable by all organizations; © included in financial filings; * designed to solicit decision-useful, forward-looking information on financial impacts; and * [strongly focused] on risks and opportunities related to transition to lower-carbon economy. Source: TCFD Final Report Chapter 3 Climate Change Risk ® 47 eliminate, net greenhouse gas emissions to reach net-zero emissions—a goal that many countries have set for them- eliminating emissions—for instance through conversion to hydrogen-based production—as opposed to having to prematurely close a facility, thus stranding it (see Section 3.2.1). selves to reach by 2050; however, some countries have set slightly earlier goals (for example, Finland has set a goal for 2035), and some countries have set slightly later goals (for example, China has a goal for 2060), and there At the corporate level, vulnerability can refer to the lack of preparation for such issues as climate change mitigation and adaptation planning, or it can apply to a lack of financial are other jurisdictions that have not yet made any net-zero resilience, such as through insurance-based mechanisms. commitments. Overall, the interaction between hazards or drivers, expo- Physical risks result from hazards that are usually subdivided into acute and chronic hazards. The former includes sures, and vulnerability then produces the overall effect of climate risk (see Figure 1). weather-related or weather-exacerbated events, whose incidence are increasing with climate change, such as 3.2.1 Stranded Assets floods, hurricanes, and wildfires. The latter includes gradual, long-term trends such as rising average temperatures and sea levels. The equivalent drivers of transition risk include factors such as tighter government policies to reduce emis- sions (e.g., through carbon taxes), technological changes (e.g., cheaper renewables making fossil fuel-based power generation less economical by comparison), and bottom-up consumer pressures for sustainable products. But for both kinds of risk to become manifest, hazards (or driving factors) are not enough alone. In the face of these hazards and factors, different kinds of assets and companies will have differing levels of exposure and vulnerability. Exposure, here, is used in the classic financial sense of assets or firms that are in a vulnerable place or setting. A factory or warehouse in a low-lying coastal area would be exposed to sea level rise, and consequently so would the firm that owns the factory. A high-emissions facility, such as a coal-fired power plant or a steel plant, would be exposed to tighter climate policy, such as a higher carbon price. Vulnerability—a concept linked to notions of resilience, flexibility, and adaptation—is less of a focus in traditional financial risk, but it is integral to considerations of climate risk, especially physical climate risk. It refers to the propensity or predisposition of the asset (or firm) to suffer adversely from its exposure to hazards. At the facility level, vulnerability to physical climate risk typically depends on physical infrastructure: For example, of two neighboring factories in a flood zone, only one may have flood pumps The notion of stranded assets is particularly important in the context of climate risk, and it has grown to be an important focus of research and analytical efforts in recent years to better understand climate, environmental, and broader sustainability risks. Stranded assets are assets that have “suffered from unanticipated or premature write-downs, devaluations or conversion to liabilities” (Caldecott, 2013). The concept of assets left behind by external forces, leading to their having reduced valuations or even becoming worthless, is not new, and it links to the idea of creative destruction. While the metaphor is obviously a physical one, the concept was first significantly applied to climate risk in the early 2010s in relation to the notion of “unburnable carbon” (for example, known oil or coal reserves that cannot be fully exploited if the agreed goals of limiting warming to 2°C or 1.5°C are to be met, thus making coal mines or oilfields into “stranded assets”). From there, the concept extended quite naturally to high-emitting industrial assets such as coal-fired power plants (see section 3.4.1) or steel plants. The concept of stranded assets still refers most commonly to these assets with high transition risk, though the definition has been steadily expanding. For instance, physical risks can equally create stranded assets, indeed harkening closer to the physical meaning of the original metaphor. Just as a boat stranded on a lakeshore after a drought lowers the water level, a beverage factory can be stranded by the increased frequency of climate-induced Vulnerability can also be discussed at the facility level droughts limiting availability to fresh water. Unlike stranded assets due to transition risk, which are likely to be more concentrated in the energy and industrial sectors, stranding due for transition risk, referring to the ease of reducing or to physical risk can occur across nearly all sectors, but it is installed. 48 & Sustainability and Climate Risk Exam Physical Risk = Acute weather hazards (floods, cyclones, droughts) Facility level: Anything in a Facility level: Extent of hazard zone (infrastructure, adaptive infrastructure (e.g. residential property, flood pumps, fire breaks) * Chronic weather Corporate level: Viability of Corporate level: Firms with contingency plans; access to insurance facilities/supply chains in hazard areas commercial facilities) hazards (sea level rise, heat, water stress) - Hazards / aon x Exposure x Vulnerability = Policy changes (carbon _| Facility level: HighFacility-level: Extentof emissions assets (fossil fuel | ability to decarbonize (e.g. tax, coal shutdowns) Transition Risk = Technological changes (cheaper renewables) = Consumer pressure power plants, steel plants, | biomass or hydrogen ICE vehicles) conversion) Corporate level: Firms with business operations dependent on emissi ns Corporate-level: Viability/robustness of transition plans Source: Caldecott et al. (2021, forthcoming) Physical and Transition Risk more likely to be concentrated geographically: for example, sea level rise and increased coastal flooding may strand any number of facilities—warehouses, factories, commercial or the physical impacts of climate change are already here, residential properties—in vulnerable areas without adequate in the near future, it is certain that the effects of climate flood defenses (see Section 3.3). That said, some sectors are change will continue to worsen. The exact outcome will more vulnerable than others—see Section 3.6 for a discussion depend on future emissions, which affect the amount of of physical risk in agriculture and real estate. warming. Current policies put the globe on track for 3°C or While beyond the scope of this chapter, it should be noted that stranded assets can also refer to assets stranded by non-climate environmental risks. Habitat destruction and biodiversity loss can lead to declines in, or even collapses of, ecosystem services (for example, a lack of pollination in a particular area stranding fruit orchards). Tighter restrictions on local air pollution, such as particulates and smog, can and specific events can be definitively linked to climate change through attribution science (see Section 3.3.1). And more of warming, though commonly used scenarios range from 4.5°C of warming by 2100 to 1.5°C if the most ambitious Paris goals are met. Often underappreciated, however, is that even if the world manages to hit these ambitious targets of 1.5°C or 2°C, the physical impacts will still be severe. One important reason is that the distributional con- sequences are uneven: 1.5 or 2°C of warming on average end up stranding assets such as polluting factories or old vehicles in areas that impose stricter curbs on pollution. still means more intense heat waves, more intense precipitation, and more droughts, among other phenomena. For example, the IPCC reports that a world with an average of 3.3 Physical Risks 1.5°C of warming compared to pre-industrial times—the most optimistic of all climate outcomes—still means Arctic Before considering physical risks as such, it is important to winters that are on average 4.5°C warmer, and more fre- understand what the physical impacts of climate change entail. Rather than being an abstract future phenomenon, quent Mediterranean droughts, and bouts of heavy precipitation across several regions (IPCC, 2018). Chapter 3 Climate Change Risk B 49 From a risk perspective, physical risks result from hazards, and as discussed above, these are typically subdivided into with human activities estimated to have caused warming of approximately 1.0°C above pre-industrial levels (see left graph, below), and where climate models predict a clear acute and chronic hazards, which then interact with exposure and vulnerability. This section covers the hazards in trend of further warming to come, with the main variable being the amount of greenhouse gas emissions humanity detail, splitting into examinations of acute and chronic hazards as outlined by the recommendations of the Taskforce on Climate-Related Financial Disclosures (TCFD)—recom- continues to release into the atmosphere (see right graph, mendations that have been followed by stakeholders since on the next page). Under a high-emission scenario, temperatures are predicted to rise by at least 4°C by 2100, whereas they were put in place. It then discusses data availability under a low-emissions scenario, they will stabilize around and granularity and touches on exposure and vulnerabil- 1.5-2°C. (For more on scenario analysis, see Chapter 7.) ity. Finally, it closes with a short discussion of the (limited) But for many other physical climate hazards, understanding them is not as straightforward. While all major climate models agree that precipitation patterns will change, they disagree as to the magnitude and even as to whether opportunities from physical risks, while recognizing that most corporate and investor action toward physical risk will, by necessity, be focused on reducing or avoiding the potential for losses. precipitation will increase or decrease). This is why graphics from the IPCC use cross-hatching, a form of shading that looks like diagonal squares, to indicate where models agree. In the graphic below, cross-hatching indicates where 3.3.1 Acute and Chronic Climate Hazards Physical climate hazards are typically subdivided into acute and chronic hazards. The former includes weather- at least two-thirds of models agree whether precipitation will increase or decrease in 2100 scenarios with 1.5°C related or weather-exacerbated events whose incidence is increasing with climate change, such as floods, hurricanes, and wildfires. The latter includes gradual, long-term trends, or 2°C of warming (with large portions of the globe not covered). This can be contrasted with the far more robust like rising average temperatures and rising sea levels agreement on the direction and magnitude of temperature (see Table 1). change. There is a strong scientific evidence base, both from his- Another important point to note is that models give varying torical evidence and from cross-comparing extremely degrees of accuracy not just for different hazards but also sophisticated climate models, that all of the above hazards are linked to climate change in general. However, there is a wide range in the ability of models to predict the mag- for different timescales. While the models used by the IPCC are designed to look several decades ahead and provide reasonable and fairly robust estimates for 2100, climate models provide only limited information for the next one or nitude or frequency of specific hazards, the specific timeframe of their occurrence, or their specific locations. two decades, particularly on smaller geographical scales, Average global temperature rise makes for a good first example, as the trend is clearest here in both historical data, because natural variability in climate can mask the effect of Key Chronic Climate Hazards: human-caused changes in the climate (Fiedler et al., 2021). Key Acute Climate Hazards: © Average temperature rise Increased incidence of the following: © Sea level rise * Storms and hurricanes * Changing precipitation patterns * Droughts © Heatwaves 50 Sustainability and Climate Risk Exam © Precipitation extremes and floods © Wildfires Average Temperature Rise to Date: Projected Temperature Rise: snd data prepared by Berkeley Earth and combined with ocean data adapted from the UK Hadley Centre (Global temperature anomalies relative to 1820-1900 average ‘Vertical lines indicate 95% confidence intervale 1860 1880 1900 1920 1940 1960 1980 2000 go id Global Temperature Anomaly (°C) Global Average Temperature 1850-2020 2020 -0.4 Source: Global Temperature Report for 2020 (Berkeley Earth). Model mean global mean temperature change for high neres 2 204 | global mean Trodel tnean temperature Change for low 214 ood 3 9 20 1900 ae T 1950 T RCP2.6 T 2000-2050 Year 2100 Source: Collins, M., M. Wehner, et al., 2013: Long-term Climate Change: Projections, Commitments and Irreversibility. In: Climate Change 2013: The Physical Science Basis. Contribution of Working Group | to the Fifth Assessment Report of the Intergovernmental Panel on Climate Change. EXAMPLE 1 OF MODEL UNCERTAINTY: Mean temperature change at 1.5°C GMST warming GLOBAL PRECIPITATION ‘Mean temperature change at 2.0°C GMST warming CHANGE Temperature (°C) os 1 15 Mean precipitation change at 1.5°C GMST warming 2 3 6 8 ‘Mean precipitation change at 2.0°C GMST warming Source: IPCC Chapter 3 Climate Change Risk @ 51 EXAMPLE 2 OF PREDICTIONS MODEL UNCERTAINTY: CESM1-BGC VALIDATING WILDFIRE CCSM MPI-ESM-MR: 0 10.0 20.0 50.0 100.0 Reprinted from Kloster, Sil ia, Lasslop, Gitta “Historical and future fire occurrence (1850 to 2100) simulated in CMIP5 Earth System Models” (March 2017) Global and Planetary Change, Volume 150, Pages 58-69. Of course, some climate hazards will require an interac- measurements of the actual area burnt from satellite data tion between climate events and local conditions, such as (see the panel labeled GFEDv4S above), showed that many flooding or wildfires. Large amounts of rainfall within a short period of time make it more likely that a river will overflow its banks, but the particular topography of a specific river valley also affects how and where flooding will occur (not to mention the presence, or absence, of human-built flood defenses). Droughts make it more likely for fires to start, but wildfires need vegetation to burn, meaning models must take both climate and land cover into account—and, models overestimated the burning potential in North and South America while underestimating it in Africa. To point out the shortcomings of, and disagreements between, models is not to say that they are not useful, merely that given some uncertainty in the models themselves, calculating actual direct physical risk is a step more difficult (see next section). ideally, human land-use patterns as well. The interaction For acute physical hazards, it is not necessary to rely on of these multiple factors makes it particularly difficult for models exclusively. The increased incidence of acute haz- models to accurately predict wildfire locations. One study ards is already visible in the natural catastrophe data from to validate models, which compared five different model- the past several decades of (see graph, below), and this predicted wildfire areas (panels 1-5 of Example 2, above) to. trend is expected to continue. 52 Sustainability and Climate Risk Exam Number of Events , g Total ml Hydrological events ml Meteorological events al limatological events ml Geophysical events Moreover, a relatively new offshoot of climate science, attribution science, is now able to show how specific events are require an interaction between climate events and local conditions, as discussed above in Section 3.3.1 with regards (partially or almost completely) caused by climate change. to wildfires. Droughts make it more likely for fires to start, but wildfires need vegetation to burn. Secondly, and perhaps more importantly, as discussed above in Section 3.2, According to research of this type, the devastating Australian wildfires of 2019-2020, for example, were made at least 30% more likely by climate change, and the huge Thai floods of 2011 would likely not have occurred without climate change. The July 2019 heatwave over France and the Netherlands would have been extremely unlikely to occur without human influence on climate. Similarly, the Siberian heatwave of 2020, when temperatures reached a record 38°C in Verk- hoyansk, far above the Arctic Circle, would have been almost impossible without human-caused climate change. physical risks occur only when there are also exposure and vulnerability. Getting precise enough data on these hazards is not neces- sarily straightforward either. Some is available open source or free from academic institutions or international organizations or non-governmental organizations such as the World Resources Institute; other climate data can be bought from specialist consultancies, some of which also offer their own ready-made ways to evaluate exposure and vulnerability. For 3.3.2 Direct Physical Risks and Data Granularity some hazards, such as flooding, which depends on hyper-local Understanding direct physical risks as they apply to a specific asset or company, or even country, is not straightfor- JBA Consulting, an industry leader in its field, sells flood map ward. Beyond the differences between the different global that is downscaled from global climate models appears at a climate models’ estimates for various hazards (see above), readjusting these local climate models to derive regional or much rougher resolution, such as 100 km x 100 km, and even still suffers from lack of robustness over shorter timescales, as local estimates leads to less precision. discussed in Section 3.3.1. Moreover, climate hazards do not, alone, cause climate risks With climate data in hand, mapping exposures is not exces- to become apparent. Firstly, certain types of physical risks sively difficult, and it only requires precise location data to topography, resolution matters tremendously: for instance, data at a 5m x 5m resolution. Much of the other climate data Chapter 3 Climate Change Risk ® 53 cross-compare with climate hazards. Investors in, owners of, or lenders to specific physical assets, such as a particu- lar warehouse or wind farm, should already know where the asset is located. And although a firm should know the location of its own production and office facilities, investors in, or lenders to a firm may not currently know precise geolocations. Vulnerability, however, is an area where there is still very little visibility or data because it ultimately refers to facility-level preparedness: Two nearby factories in the same flood-prone area might be affected differently if only one has flood walls, For instance, a company could be sued for not adequately preparing for physical risks. One such example currently making its way through US courts is Conservation Law Foundation v. Shell, an ex-ante claim by the Conservation Law Foundation, a non-governmental organization, filed in 2017 against Shell, the multinational oil and gas firm, claiming it had failed to satisfactorily prepare its Providence Fuel Terminal for sea level rises and the increased frequency and intensity of severe weather events (“Conservation Law Foundation, Inc. v. Shell Oil Products US"). basement pumps, or worker contingency plans. A firm plan- A third, more nebulous, category are the indirect physical risks that can be considered systemic in their propagation ning a large greenfield capital expenditure, such as a new effects and their multifaceted nature. A good example is factory, will be able to hire consultancies that specialize in climate engineering to evaluate the present state of adaptive infrastructure, such as floodwalls or the cost of building the nascent literature from both academia and policymakers quantifying the effects of heat stress on worker productiv- them. But a large firm may not have detailed information on all of its own legacy assets, let alone on interdependencies through its supply chains (see also next section). And other ity. The International Labor Organization, for instance, estimates that by 2030, when the global temperature is expected to have risen by 1.3°C, the share of total working hours lost will be 2.2%, or the equivalent of 80m full-time counterparties with stakes in a firm's continued well-being, jobs (up from 1.4% and 35m jobs as recently as 1995). In such as banks, institutional investors, auditors, or credit rat- monetary terms, it estimates this loss at USD 2.4 trillion ing agencies, may have even more limited data access. in purchasing power parity terms (compared to USD 280 billion in 1995, meaning a net loss of over USD 2.1 trillion 3.3.3 Indirect Risks: Supply Chain, Legal, and Systemic Risks Physical risks do not only affect assets and the companies that own them, through exposure to hazards, but there are several other ways in which climate-related physical risks can affect assets and firms. Perhaps the most straightforward of these is supply chain risk. Of course, this risk is partly a matter of classification. Two identical firms, one that is vertically integrated and another that has outsourced production to suppliers, may both be subject to the same physical risks in the production process— only for the first, this would be counted as affecting the firm itself, and for the second, it would count as a supply chain risk. Nonetheless, all firms rely to an extent on suppliers. A second important kind of indirect risk is liability risk, where firms suffer financial consequences after being held legally liable. Liability risk can exist for both physical and transition risks (see Section 3.4.1 on transition-related in 35 years of climate change alone). It further warns that these are likely to be underestimates, as they are based on the assumption of limiting warming to 1.5°C by 2100, which looks extremely unlikely (ILO, 2019). 3.3.4 Opportunities, Resilience, and Adaption While many climate risk management discussions seek to balance risks with opportunities, when discussing physical risk specifically, the argument is harder to make. Physical risks, at least for private-sector counterparties, tend to bring more downside than upside. Occurrences such as hurricanes, wildfires, or floods can bring huge losses through facility destruction and business interruption. Paying large amounts to adapt and fortify facilities against physical risks, such as through building firebreaks, floodwalls, or better air conditioning systems, does not produce a profit or revenue stream per se, but rather simply avoids large losses that would have otherwise happened. For corporations, insur- play with regard to the duties and responsibilities of cor- ance availability is crucial to mitigate financial losses, and thus insurers’ willingness to pull out of coverage in cases of particularly severe exposure to physical climate risks, as porate management or a company’s board of directors. has already occurred on a limited scale in fire-prone areas liability); for physical risk issues, liability usually comes into 54 Sustainability and Climate Risk Exam of California and Australia, would be an additional red flag. Thus, it is easy to envision a scenario where some firms and financial counterparties stop investing in areas that are most vulnerable to physical climate risks, such as low-lying coastal areas, leaving only the most vulnerable there, such as homeowners unable to sell residential properties, and allowing physical climate risk to become a problem for government exclusively. There is, however, some chance that the corporate sector can find limited opportunities in dealing with physical risk by partnering with governments and with other kinds of counterparties, such as insurers. It may be in all stakeholders’ interests to find solutions that allow for both burden- and profit-sharing through avoiding physical risks. Companies could be rewarded for staying in certain areas, or could partner with communities to build adaptive infrastructure such as flood walls that can protect both facilities and factories and surrounding communi- 3.4 Transi ion Risks and Opportunities Before discussing transition risks in detail, it is important to take a step back to contextualize just how large the “transition” in question is. Business-as-usual scenarios assume that with no economic transition, COz levels will continue to rise from the current level of around 40 gigatons (Gt) per year to possibly around 60-120 Gt. To reach international goals of keeping warming to below 2°C, emissions would need to start dropping quickly and in a sustained manner at an unprecedented pace (see purple and blue pathways of top left figure below). From decades of consistently rising global emissions, the whole global economy, especially electricity and heat production (25% of emissions), industries (21%), transportation systems (14%), and agricultural practices (24%) (see top-right figure) must be thoroughly overhauled to reach net-zero emissions by 2050. ties. Insurers, rather than simply threatening to pull coverage, Moreover, reaching the goal of limiting warming to 1.5°C, an can work proactively with firms and communities to encourage aspiration of the Paris Agreement, is nearly impossible at this stage through emissions reductions and eliminations alone the uptake of adaptive measures (e.g., through premium discounts) and share expertise on resilience. (see left figure below), as it is the stock of greenhouse gases Data: SSP database (IASA)/GCP/Riahi et al. 2017/Rogel et al. 2018 140 Scenario group Forcing target and temperature range in 2100 esc cp 100 | 6.0 Wim? (3.2-3.3°C) } 4.5 Wim? (2.5-2.7°C) 2 3.4 W/m? (2.1-2.3°C) g 2.6 Wim? (1.7-1.8°C) 5 B €§. wee 1.9 W/m? (1.3-1.4°C) 50 ore 0 ec fe}8 Zz 40 Kenrageiog Eclemrans 1980 2000 2020 2040 2060 Source: IPCC ARS Climate Change 2080 2014: Mitigation of Climate 2100 Change. Source: “Explainer: How ‘Shared Socioeconomic Pathways’ explore future climate change” (April 2018) Chart produced for Carbon Brief by Glen Peters and Robbie Andrews from the Global Carbon Project. Reprinted with permission of CarbonBrief. Chapter 3 Climate Change Risk ® 55 Pathwayto 15°C given given cumulative00. sa Sy8 CO, Emissions (GtCO,) we& Annual global CO, (Gt CO»/yr) Limiting warming to 1.5°C is increasingly difficult without large-scale negative emissions 45 40 Pathwayto TSC ger given cumulative 0 1980 2000 2020 2040 2060 2080 2100 2020 2040 2060 Year 2080 2100 Reprinted from UNEP: 1.5C climate target “slipping out of reach” by permission of CarbonBrief. in the atmosphere that matters, not the flow. Therefore, worldwide. However, currently, there is not a global carbon most models and scenarios for reaching a 1.5°C equilibrium, including ones used by the IPCC, assume very large amounts of net-negative global emissions (see bottom-right figure and ‘tax, and the price of carbon is not anywhere close to high enough on its own to reduce emissions sufficiently to reach internationally agreed-upon targets. also top-left figure). Natural ways to remove carbon from the Therefore, more recently, there has been a recognition that atmosphere, such as planting more trees, can only address a small fraction of these needs: To reach the scales assumed in these models would require massive and extremely rapid rollout of technologies and practices such as directly captur- ing CO» from the air (known as direct air capture, or DAC), or bioenergy with carbon capture and storage—growing plants, which absorb CO> from the air, then burning them to generate electricity, but capturing the CO2 from combustion and burying it, thus resulting in net removal. the means through which emissions (the drivers of transmis- sion risk) will actually be reduced will be both more varied and less neat than simply imposing a high carbon tax that would seem an optimal solution in economic models, but that has so far struggled to gain ground in light of political realities. This section follows TCFD-outlined best practices Seeing the pace and change required to meet any of these climate goals, it is easy to imagine that these would be in describing transition risk drivers as including policy and legal risks (encompassing factors ranging from mandatory shutdowns to legal liability); technology risks (e.g., cheaper renewables making fossil fuel-based power generation less economical by comparison); reputational risks (e.g., from pursued mainly, or even exclusively, through government bottom-up consumer pressures for sustainable products); policies, and therefore that policies would be the primary and other risks, such as market risks. or even only source of transition risk. For decades, both This section concludes with a discussion of the various policy developers and academic economists have discussed opportunities presented by the transition. More so than the optimal way to reduce (and eventually eliminate) green- with physical risk, where the main focus is on avoiding or house gas emissions, and these discussions have revolved reducing the potential for losses, the converse of transition around centralized policy options such as imposing a price risk can be found in a myriad of economic and investment per ton of carbon dioxide emitted through a carbon tax or opportunities presented by the speedy, wholesale transfor- limiting quantity through a cap-and-trade scheme—both mation of the economic structure that much of the world has committed to undertake. of which have been implemented in various jurisdictions 56 Sustainability and Climate Risk Exam 3.4.1 Policy and Legal Risks The sources of policy and legal risk in causing climate transition risk are varied. As already described, the classic policy solutions for achieving emissions reductions have tended to be either carbon taxes, setting a price on emissions, or cap-and-trade schemes, which set a limit on quantity. But other important policies can also lead to transition risks. One important example is that of energy efficiency, such as energy requirements for residential buildings or emissions requirements for new automobiles—which can be set at both the level of individual vehicles or at the fleet level. Another source of policy risk is government-mandated clo- sures, ranging from cancellations of previously agreed fossilfuel infrastructure projects, such as natural gas pipelines and mandatory shutdown dates for certain types of high-emitting facilities, such as coal-fired power plants. Other examples include energy-efficiency regulations and limits on pollution. Legal risks, when a firm is held liable, can result in various consequences ranging from fines or penalties to class action damages or changes in valuation. In relation to transition risk, there are at least two potential strands of litigation that can result in risk to companies. A relatively straightforward one is a tactic increasingly adopted by activist and advocacy organizations—filing suit in court against carbon-intensive projects on the grounds that these projects breach existing environmental rules or commitments and should not have been approved. A prominent example of this is the suit filed by ClientEarth, a climate litigation NGO, against Enea, a Polish utility, alleging failure to consider the material climatetransition risks of its project to build a large €1.2 billion coal-fired power plant, Ostroteka C (“ClientEarth v. Enea,” 2019). (In this particular case, the Poznan District Court did find in ClientEarth’s favor in 2019, but they did so on a technicality, meaning the judge did not need to formally deter- mine whether Enea had failed to consider climate risks.) A much broader strand of legal risk comes from nascent efforts to directly pin legal liability on firms for their proportional contribution to the physical impacts of climate change through their emissions. A landmark case in this regard that is slowly making its way through German courts is Lliuya v. RWE, EXAMPLES OF TRANSITION RISK FROM PRICES OR CAP-AND-TRADE: SOURCES © July 2020: Germany passed a law mandating the closure of all coal-fired power plants by 2038 at the payment, Tesla’s car output was counted as part of FCA‘s fleet for regulatory purposes, thus lowering the average fleet emissions. latest and providing compensation to owners. Many of these plants will be shutting before the end of their operational lifetime, which can be 30-50 years, thus OTHER THAN CARBON © January 2021: In his first day in office, the new US president, Joe Biden, cancelled the permit for the becoming stranded. (For instance, Germany's last new planned Keystone XL oil pipeline to cross from Can- coal power station, Datteln 4, commissioned in sum- mer 2020, will thus only have a maximum of 18 years ada into the United States, thus terminating the project. Though there were also other reasons that led to to run, though the owner, Uniper, may consider clos- such a large political movement against the project, ing it earlier.) © April 2019: Fiat Chrysler Automobiles (FCA), an Italian-American automobile manufacturing group (now part of Stellantis after a 2021 merger with including opposition from Native Americans and from local ranchers, the pipeline, which would have carried emissions-intensive Albertan oil that was derived Groupe PSA of France), was forced to pay Tesla, an from tar sands (a process in which the sand has to be heated and processed to release oil), was also seen all-electric American car manufacturer, hundreds of millions of euros to avoid fines for breaking E.U. as incompatible with making progress toward climate change mitigation. standards on average fleet emissions. Through the Sources: Energy Central; Reuters; FT; New Yorker. Chapter 3 Climate Change Risk ® 57 where a Peruvian farmer alleges that the emissions of RWE, a German utility, partially caused the glaciers around assets and laggard firms reliant on outdated technology that becomes less economical, or even obsolete. a particular lake to melt due to their historic emissions, Perhaps the most powerful example of these technology which increased flood risk. This line of argument relies on risks so far involves the technological development of renewable energy. In the past decades, wind turbines and solar photovoltaic (PV) panels have fallen so steeply in price the contention that RWE can be held proportionally liable ‘on the basis of its pro-rata contribution to all human emissions, which is estimated at 0.47% of historic CO2 emissions that they are now cost-competitive with, or even cheaper than, traditional power generation technologies reliant on (Luciano Lliuya v. RWE AG). While still at an early stage, if this case succeeds, legal liability risk may be a much larger fossil fuels, such as coal-fired or natural gas power plants— concern and source of risk for firms than it is currently. even without any subsidies, surcharges, or carbon taxes (see Case Study in Section 3.6). Solar modules, as an extreme 3.4.2 Technology Risks example, have declined in price from over USD 100 per As much as changing technology is seen as a boon, it can also serve as an important driver of transition risk for older watt in 1976 (measured in 2019 dollars) to well below USD 0.50 per watt in 2019 (see graph). The price of solar modules declined by 99.6% since 1976 Price pre Watt of solar photovoltaics (PV) modules (logarithmic axis) ‘The prices are adjusted for inflation and presented in 2019 US-S. With each doubling of installed capacity the price of solar modules dropped on‘average by 20.2%. This is the leaining rate of solar modules. 10 MW 100 MW 1,000 Mw 10,000 MW 100,000MW >, Cumulative installed solar PV capacity (logarithmic axis) Data: Lafond et al. (2017) and IRENA Database; the reported learning rate is an average over several studies reported by de La Tour et al. (2013) in Energy. The rate has remained very similar since then. (OurWorldinData.org - Research and data to make progress against the world’s largest probleme. Source: IRENA, Our World in Data Lafond et al. 2017. 58 & Sustainability and Climate Risk Exam Licensed under CC-BY by the author Max Roser Other technologies, such as lithium-ion batteries, are on similarly steep learning curves, becoming ever cheaper. Batteries, in turn, can be used not only for utility-scale energy storage, further strengthening the usability of wind and This concept is related to the more abstract notion of the solar energy for electricity that would otherwise be intermit- unconscionable by wider society. While it is difficult to single out a particular firm or sector that completely went out tent, but also for other sectors. The continued cheapening of, and improvement of, battery technology makes it conceivable that the transportation sector will be the next to be hit by technology risks and “social license to operate,” whereby firms’ business models must satisfy a minimum requirement of acceptability and cannot be so noxious as to be considered immoral or of business due to this alone, some sectors, such as tobacco or certain weapon manufacturers, have at times come close—certainly close enough for this to impact their share prices and access to capital markets. Some of the highest- potential asset stranding. According to BloombergNEF, electric vehicles will hit price parity with internal combustion emission, climate-unfriendly industries, such as coal and tar engine (ICE) vehicles once battery pack prices (the single most expensive component of EVs) reach USD 100/kWh. sands, are now starting to become seen as broadly noxious, and many firms exclude lending or investing in them. Based on current trends, with 2020 battery pack prices around USD 137/kWh (down from over USD 1000 in 2010), Finally, but certainly no less important, bottom-up pressures from consumers and stakeholders can also be an important driver of change—for flexible firms, greater opportuni- the threshold of USD 100 is expected to be reached around 2023. After this point, EVs will be as affordable as, and after, ties, and for firms that are inflexible or slow to respond, even cheaper than, combustion engine vehicles—even without subsidies or further policy incentives, meaning there will transition risk. In consumer products sectors, as consumers become more aware of the climate impact of their pur- be a strong economic incentive to switch and that existing ICE fleets may end up becoming stranded assets. chases, agile firms will respond by increasing the selection and availability of sustainable products, whereas staid firms will be left behind. Consumer influence is most obvious 3.4.3 Reputational Risks and Consumer Pressure Reputational risks and bottom-up or lateral pressures from in household goods or food products (for example, the increasing recognition of the high climate footprint of meat and dairy products, which is driving part of the demand for consumers, clients, suppliers, or employees can be an plant-based meat and dairy alternatives). important source of transition risk. The classic type of repu- But using a broader definition of “consumer” to encompass tational risk is where a firm's brand and image are tarnished all sorts of client-supplier relationships, this type of pres- by its association with industries that are seen as unsavory sure can be seen to exist also in other sectors. For instance, (see example). Apple’s commitment to carbon neutrality in its supply chain EXAMPLE: GOLDMAN REPUTATIONAL SACHS RISK RELATED TO CLIMATE—CASE Goldman Sachs is arguably the most renowned and The bank has many mechanisms in place which seek to pre- prestigious Wall Street investment bank; hence, the firm empt and mitigate climate-related reputational risk. The takes issues of reputational risk seriously and singles bank's board of directors places “significant focus” on issues them out in its TCFD reporting. It says that “involvement in certain industries associated with climate change” may pose reputational risk to the firm. Specifically, it says it of reputational risk in its considerations. The bank also has may lead to “reduced client and employee loyalty, investor divestment and impacts to client activity.” a firm-wide Reputational Risk Committee, which provides oversight over transactions that include some climate-related dealings that may attract heightened reputational risk. Source: Goldman Sachs 2019 TCFD Chapter 3 Report. Climate Change and products by 2030 has led it to demand sustainable raw materials, resulting, for instance, in its purchase of the world’s first zero-carbon aluminum from an Alcoa-Rio Tinto joint venture. In a similar vein, commodity markets that have previously been fairly homogeneous have seen a trend toward separate labeling and marketing due to upward pressure. A good example is the separate labeling and certification of “green steel,” in comparison to normal steel, which allows end users to differentiate between steel made in more environmentally friendly ways (such as through the use of hydrogen) rather than the typical method that involves burning large amounts of coal. 3.4.4 Market and Other Transition Risks Other notable sources of transition risks include the notion of market risk, which in relating to climate transition risk batteries, and copper, used widely in electric vehicles and other electric equipment. Another kind of market transition risk that is already manifesting itself is through shareholder perceptions or pressures in public markets. A large portion of the value ascribed to oil & gas firms, for instance, has historically been due to their proven reserves, but now that a portion of these reserves seems likely to become stranded, market capitalizations of oil majors have fallen. Oil majors have been forced to write off asset: in June 2020, for instance, oil-major BP cut the value of its assets by a full USD 17.5 billion, and Royal Dutch Shell by USD 22 billion. 3.5 Transmission into Finance, encompasses a broad range of potential supply, demand, the Economy, and Key Sectors and pricing effects. Climate risks matter not only for the assets or firms directly affected but also because they can propagate and have an effect throughout the financial system and the broader The most straightforward kinds of market transition risks relate to the shifting demands from high-emissions products to low-carbon products and commodities. As industries and equipment transition to using cleaner fuels or become elec- trified, the demand for coal will fall, potentially completely; oil and gas demand will also likely reduce significantly, though oil has non-combustion uses such as in petrochemicals. This will then filter through to sectors such as coal mining or bulk coal shipping. Likewise, shifts and spikes in economy and key sectors. Ultimately, climate risk becomes relevant to risk managers when climate change’s physical impacts, or government policies meant to reduce emissions, have an effect on balance sheets and portfolios of firms or financial institutions. There are a number of channels through which these risks can propagate to corporate balance sheets and the financial system. This section explores are needed for low-carbon infrastructure, may cause market these through two sectoral examples: that of physical risk in real estate (with an accompanying short section on transi- disruptions. For example, there have been periodic con- tion risk) and that of transition risk in electricity generation cerns around the availability of lithium, crucial for lithium-ion (with a section on physical risk as well.) demand for low-carbon commodities, or commodities that CASE STUDY 1: REAL ESTATE Physical risks and real estate Real estate is a sector particularly affected by physical along rivers for historic reasons, flood risk is another very and if those locations are in vulnerable, exposed areas they will be subject to climate risk. Wildfire and flood widespread and potentially severe risk in the real estate sector. One analysis in 2019 found that 19% of retail climate risk, as buildings obviously have fixed locations, risk are particularly acute for real estate. In a potentially wider warning sign, in certain particularly wildfire- ravaged parts of Australia and California in 2019-2020, local insurers paused or refused to continue selling 60 residential property insurance. Due to the desirability of living near the coast, and the typical location of cities & Sustainability and Climate Risk Exam spaces and 16% of offices in Europe are exposed to floods and/or sea level rise, with even higher proportions in more severely affected cities, such as 23% in Glasgow (Four Twenty Seven, 2019). But perhaps the most precisely documented physical risk transmission mechanism is that of the coastal flooding risk in the United States, from both sea level rise (a chronic physical hazard) and the risk from an increased frequency of storms and hurricanes (an acute hazard) the energy use of buildings, particularly heating. After all, the only direct emissions from most residential or commercial non-industrial buildings are from heating, which often still relies on natural gas or sometimes oil in transmitting into the property market and thence to rural areas. Electricity use can also be seen to contribute to transition risk in areas where the electricity grid is banks, insurers, asset managers, and owners and the itself still highly reliant on fossil fuel-based sources of wider financial system. In this particular transmission electricity, such as coal or natural gas. gives fairly precise estimates of the magnitude of these Many jurisdictions have updated building codes to require new-builds to conform to higher energy- chain, empirical evidence from academic literature effects. An analysis by Bernstein and associates in 2019 showed that US properties exposed to sea level rise (SLR) sell for 7% less on average than equivalent unexposed efficiency standards and be heated with renewable sources of energy. While these are sometimes properties matched on key characteristics; those exposed grandfathered in for older buildings, this is not always the case, and the UK's incoming Minimum Energy those exposed at 6 ft (2m) still sell at 4.4% despite this Efficient Standard (MEES) shows how buildings can cause transition risk. The UK uses a system where all at 1 ft (30cm) of SLR sell at a 14.7% discount, whereas level of SLR not expected to be reached until 2100 at the earliest (Bernstein et al., 2019). Keenan and Bradt show that local lenders pass on proportionally more SLR risk to secondary markets than large national banks, indicating that they have better access to local soft information on SLR risk (Keenan & Bradt, 2020). And Quazad and Kahn show that catastrophes prompt mortgage originators in the United States to increase the share of mortgages just properties are given an Energy Performance Certificate (EPC) rating for energy efficiency on a scale from A to G, with G being the worst. As of 2018, the average EPC for rented property was an E. Under the MEES rule, from April 2018, properties rated F or G were not allowed to be rented out on new leases, with the rule extending to existing leases from 2023, with fines for non-compliance. below the conforming limits of mortgages eligible for This could lead to transition risk for UK lenders if securitization by the government-sponsored enterprises landlords’ credit position deteriorates from lost rental income due to their properties having become ineligible (if they do not make energy-efficiency improvements), or Freddie Mac and Fannie Mae, thus passing on SLR risk to wider markets (Ouazad, 2019) (see diagram below). Bernstein et al: (2019) US properties exposed to SLR sell for 7% less on average COASTAL PROPERTIES Greater risk of current, future flooding Lower Property velit Ze Keenan & Bradt (2020): Local lenders pass on proportionally more SLR risk to markets. Ouazad & Kahn (2019): Catastrophes increase mortgages just below limit, that are passed on. Transition risks and real estate Though transition risks are not quite as acute as physical risks for real estate, they do still exist. The main route through which transition risks affect real estate is through through the market value of F and G properties falling. The Bank of England has conducted analysis showing that the energy-efficiency rating of a property is a predictor of credit risk on residential mortgages. Chapter 3 Climate Change Risk @ 61 CASE STUDY 2: ELECTRICITY GENERATION Transition risks and electricity generation FINANCIAL COUNTERPARTIES Mandatory shutdown (Transition / policy risk) (Lenders, Investors © Lower returns Ao Rising default risk| © Loss of Coal-fired power plants revenues o Asset write-offs Electricity Utilities (stranded assets) New build renewables become cheaper than operating existing coal (Transition / technology risk) The most straightforward way in which climate risks affect the electric utilities sector is through transition risk. A good ‘example is that of coal-fired power plants, which have been an obvious focus of attention because burning coal for cu: 'OMER: Physical risks and electricity generation Even if the exposure of fossil-fuel power generation to transition risk is more obvious than the connection to physical risk, the electricity generation sector can be electricity is one of the most emissions-intensive processes; significantly affected by physical climate risk, as well. Two as high as even those from natural gas power plants (with American examples from recent years serve to highlight this: California-based Pacific Gas & Electric (PG&E) and the CO, emissions per MWh of electricity output are twice 1.0 metric ton of CO; from coal per MWh versus 0.4 metric tons per MWh from gas, based on 2019 US data). Coal-fired power plants can be affected directly through transition policy risk, where mandated shutdowns, such as Germany's phased shutdowns by 2038, means coal plants close before the end of their operational lifetimes and become stranded assets (see Section 3.4.1). But coal power generation can be affected equally by technology transition risk in the form of cost competition from renewable technologies. From a global levelized cost of electricity (LCOE) as high as USD 347 per MWh for solar PV as recently as 2009, USD 190 for offshore wind, and USD 111 for onshore wind, the LCOE for all three had dropped to USD 39, 78, and 44 for solar PV, offshore, and onshore wind, respectively, as of 2020, according to data from research firm BloombergNEF. Notably, in China, the cost of new-build solar, at USD 38 per MWh, is now very close to the cost of running existing coal-fired power plants, at USD 35 per MWh. Without taking any sort of subsidies or tax credits into account, wind and solar are now the cheapest forms of new-build electricity generation for two-thirds of the global population, and the economic incentive is strong for them to displace fossil-fuel generation even without further policy action. 62 © Higher prices Sustainability and Climate Risk Exam the related wildfires in 2018 and the Texas-based power plants and an extreme cold snap in 2021. Across the western US, climate change-induced increases in summer temperatures and the intensification of droughts have been a very significant contributor to wildfires, with climate change responsiblefor doubling the cumulative forest fire area since 1984. In 2018, ina dry and warm spell, poorly maintained equipment from PG&E, California's main power utility, ignited fires, including the camp fire that killed 84 people and destroyed the town of Paradise. The firm has been held liable in court and had to declare what has been termed the “first climate-change bankruptcy”. In January 2021, an extreme cold spell caused by a deviation in the jet stream led to unseasonably cold temperatures in Texas, which froze electricity-generation infrastructure ranging from natural gas plants and nuclear plants to wind turbines, none of which had been “winterized.” This led to rolling blackouts across the state and several people freezing to death. While the lack of preparedness had as much to do with the extreme deregulation of the Texas grid, which is separate from the rest of the United States, as with direct climate risk, the incident servesto highlight the need to prepare for more extremes going forward.