Ch. 19 Sympatholytics Outline & Lecture PDF
Document Details
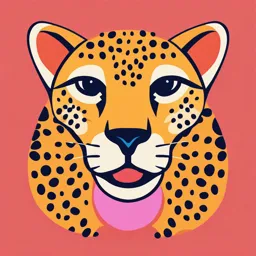
Uploaded by ImpressivePanther
Tags
Summary
This document provides an outline and lecture notes on sympatholytics, covering alpha- and beta-adrenergic receptor antagonists. The content details the mechanisms of action, side effects, and clinical uses of various drugs categorized under sympatholytic agents.
Full Transcript
Ch. 19: Sympatholytics Alpha- and Beta-Adrenergic Receptor Antagonists • • α- and β-Adrenergic receptor antagonists block the effects of neurotransmitters like NE or sympathomimetics on their respective adrenergic receptors. • This interference disrupts normal adrenergic receptor function. • It l...
Ch. 19: Sympatholytics Alpha- and Beta-Adrenergic Receptor Antagonists • • α- and β-Adrenergic receptor antagonists block the effects of neurotransmitters like NE or sympathomimetics on their respective adrenergic receptors. • This interference disrupts normal adrenergic receptor function. • It leads to a reduction in the body's sympathetic nervous system homeostatic mechanisms. • The result is predictable pharmacologic responses to the antagonists. When α2 receptors are agonized presynaptically, it also reduces sympathetic outflow by decreasing neurotransmitter release to the postsynaptic nerve. Alpha-Adrenergic Receptor Antagonists • • • • • • • • • • • • • • • • α-Adrenergic receptor antagonists selectively bind to α-adrenergic receptors and block the responses triggered by catecholamines or sympathomimetics. • Drug induced a-adrenergic blockade → inhibits the effects of these compounds on the heart and peripheral blood vessels. • It also prevents epi from inhibiting insulin secretion. Common side effects of α-adrenergic blockade include → orthostatic hypotension, reflex tachycardia mediated by baroreceptors, and impotence. Without concurrent β-adrenergic blockade, NE's cardiac stimulatory effects are maximized → leading to tachycardia • Due to these side effects, nonselective α-adrenergic antagonists are generally not suitable for managing ambulatory essential hypertension Selective alpha-1 blockers: • tamsulosin • terazosin • doxazosin • prazosin Non-selective alpha blockers (alpha-1 & alpha-2): • phenoxybenzamine → allosteric inhibitor • phentolamine → active site inhibitor rarely give alpha-1 blocker (we don’t block a-1 exclusively) will have pts on alpha-1 blockers • can expect that the alpha-1 receptors have been inhibited, vasodilated, may be on it for renal issues • ask pt why they’re on it ophthalmic drops → local effects, but need to consider if they potentially have systemic effects --------------------nonselective alpha-2: located presynaptically • a-2 normally limits release of NE (decreases NE dumped into cleft) • negative feedback • if inhibited, there will be more NE available • used in pheochromocytoma phenoxybenzamine vs. phentolamine → differences in DOA • know which is allosteric vs. active site ----------------------pheochromocytoma: • phenoxybenzamine: takes a while to work • allosteric inhibition—lock and key • long-lasting “all-star” drug → allosteric • phentolamine • active site inhibitor alpha-1 stimulation: constriction a-1 inhibition: vasodilation pheochromocytoma—adrenal medulla tumor → surges of E and NE release • causes HTN; labile, unpredictable SNS surges & catecholamine release • phenoxybenzamine—drug of choice • adrenal medulla releases: NE, E (large amt) Ch. 19: Sympatholytics • • • • a-1 blockers for prostate hypertrophy kidney: stones in ureter; a- blockers relax the smooth muscle allowing stones to pass • urinary retention MAO: breaks down NE • lives in presynaptic variscosity mitochondria • MAOI → increases NE → increased vasoconstriction • foods high in tyramine: wines, cheeses → can throw them into crisis • phentolamine – drug of choice Cocaine or meth: • cocaine prevents reuptake of NE into presynaptic variscosity • keeps NE available • hypertensive crisis from cocaine → use alpha blocker (nonselective) • beta-blockers won’t work bc there is unopposed alpha action Vasculature • Hypertension- as secondary treatment • Vasodilation leading to decreased TPR and decreased BP Bladder • Prostate hypertrophy- BPH or urine retention secondary to BPH • Relieves urine retention Kidney • Kidney stones in ureter • Acts on smooth muscle to relax • Tamsulosin (Flomax) Adrenal Medulla • Pheochromocytoma (Adrenal medulla tumor) • Phenoxybenzamine- drug of choice MAO inhibitors • Combined with foods high in tyramine • HTN crisis (180/120) • Phentolamine (drug of choice) Cocaine or methamphetamines • Can’t use beta blockers r/t unopposed alpha action • Phentolamine or Phenoxybenzamine for HTN treatment Alpha-1 blocker side effects: Vasculature •Decreased TPR and Hypotension •Flushing •Peripheral Edema- Increased capillary permeability; increased fluid leakage into interstitial space •Peripheral Edema- Decreased perfusion to kidney- reflex increase in renin •Reflex tachycardia from decreased BP • Bladder •Overrelaxation- Urinary frequency vasculature: vasodilation, decreased SVR, decreased total peripheral resistance (TPR) Eye •Iris muscle- blocked- pupil constriction- miosis •Cataract- could cause pupil prolapse (no coughing; will increase IOP) Ch. 19: Sympatholytics • o hypotension → baroreceptors stimulate SNS to maintain hemodynamic stability → reflex tachycardia Alpha-1 blocker contraindications: o cataract surgery → do not give tamsulosin o urinary incontinence → do not give alpha blockers Mechanism of Action • Phentolamine, prazosin, and yohimbine → competitive and reversible α-adrenergic antagonists. • Phenoxybenzamine, on the other hand, binds covalently to α-adrenergic receptors → resulting in irreversible and insurmountable α-receptor blockade. • Once α-blockade is established with phenoxybenzamine → high doses of sympathomimetics are ineffective until phenoxybenzamine's effects are metabolically terminated. • Phentolamine and phenoxybenzamine → nonselective α-antagonists, acting on both postsynaptic α1 receptors and presynaptic α2 receptors. • Prazosin → selectively targets α1 receptors, while yohimbine is selective for α2 receptors. • Alpha-2 mechanism: negative feedback mechanism that inhibits release NE release • BLOCKED → increase NE concentration Phentolamine • Phentolamine is a nonselective α-adrenergic antagonist, producing transient α-adrenergic blockade. • Substituted imidazoline derivative • When administered IV, phentolamine leads to → peripheral vasodilation and a rapid decrease in SBP within 2 mins • Effects lasting 10-15 mins • The vasodilation results from → α1-receptor blockade and direct action on vascular smooth muscle. • The decrease in BP triggers baroreceptor-mediated increases in SNS activity → leading to cardiac stimulation, increased HR, increased CO • Phentolamine's α2-receptor blockade enhances neural release of NE, possibly causing cardiac dysrhythmias and angina pectoris. • Increased PNS activity d/t phentolamine → result in hyperperistalsis, abdominal pain, and diarrhea. • Metabolization → primarily liver, with only about 10% of the drug excreted unchanged in the urine. Clinical Uses • Phentolamine is primarily used to treat acute hypertensive emergencies, such as those resulting from intraop manipulation of a pheochromocytoma or autonomic nervous system hyperreflexia. • A single IV dose of phentolamine (30 to 70 μg/kg or 1-5 mg) rapidly and temporarily lowers SBP • For the intraop resection of a pheochromocytoma → a continuous phentolamine infusion (0.1-2 mg per minute) may be administered to maintain normal BP • In cases of accidental extravascular admin of a vasoconstricting sympathomimetic → local infiltration with a phentolaminecontaining solution (5-15 mg in 10 mL of normal saline) is an appropriate treatment. Phenoxybenzamine • Haloalkylamine derivative that acts as a non-selective alpha-adrenergic antagonist • Combines covalently with alpha-adrenergic receptors • Blockade at postsynaptic A1 receptors is more intense than at A2 receptors Pharmacokinetics • Phenoxybenzamine is incompletely absorbed from the GI tract. • Onset of α-adrenergic blockade → slow and can take up to 60 mins to reach peak effect, even after IV admin • Delay in onset is d/t → time required for structural modification of the phenoxybenzamine molecule, necessary to activate the drug • Phenoxybenzamine has a long elimination half-time of about 24 hrs → increases the potential for cumulative effects with repeated doses • Allosteric inhibitor CV Effects • Phenoxybenzamine, when admin to a supine and normovolemic pt w/o increased SNS activity → little effect on SBP • Orthostatic hypotension → prominent side effect, especially in pts with preexisting HTN or hypovolemia. • Compensatory vasoconstriction may be impaired, leading to → exaggerated BP decreases in response to blood loss or vasodilating drugs (volatile anesthetics) Ch. 19: Sympatholytics • Despite decreases in BP → CO cardiac increased, and RBF remains relatively unchanged, unless preexisting renal vasoconstriction is present or significant hypotension occurs. • Cerebral and coronary vascular resistances → not significantly altered • Maternal treatment with phenoxybenzamine → result in neonatal hypotension and resp distress in the first 72 hrs of life in newborns Non-Cardiac Effects • Phenoxybenzamine prevents inhibitory action of epi on insulin secretion. • Catecholamine-induced glycogenolysis in skeletal muscles or lipolysis → not affected • Stimulation of the radial fibers of the iris → blocked, leading to miosis. • Sedation → SE of chronic phenoxybenzamine therapy • Nasal stuffiness results from unopposed vasodilation in mucous membranes d/t α-adrenergic blockade → leading to engorgement of the turbinates Clinical Uses • Typically admin → 0.5 to 1.0 mg/kg orally, or prazosin as an alternative • Used preop to control BP in pts with pheochromocytoma. • Chronic α-adrenergic blockade, by relieving intense peripheral vasoconstriction → allows expansion of intravascular fluid volume → resulting in decreased H&H levels • Phenoxybenzamine can reverse excessive vasoconstriction and tissue ischemia, as seen in conditions (hemorrhagic shock), but this should only be done after intravascular fluid volume has been replenished to prevent worsening hypotension • α-Adrenergic blockade with phenoxybenzamine does not have a favorable influence on the tx of PVD → characterized by intermittent claudication b/c it primarily increases cutaneous blood flow rather than skeletal muscle blood flow. • The most beneficial clinical responses to α-adrenergic blockade are observed in dzs with a large component of cutaneous vasoconstriction (Raynauds), where it helps improve blood circulation to affected areas with narrowed arteries supplying blood to the skin Yohimbine • Selective antagonist at presynaptic α2 receptors → leading to increased NE release from nerve endings. • Useful in treating idiopathic orthostatic hypotension by enhancing NE release. • Yohimbine has been used in the past for male pts with erectile dysfunction or impotence, especially if it results from vascular, diabetic, or psychogenic causes. • Can readily cross the BBB, potentially leading to → increased skeletal muscle activity, tremors, and other SEs. • Excessive doses of yohimbine can cause → tachycardia, HTN, rhinorrhea, paresthesias, and dissociative states. • Yohimbine's interaction with volatile anesthetics is suggested by observations that α2-adrenergic agonists can reduce anesthetic requirements by acting on presynaptic α2 receptors in the CNS. Doxazosin • Doxazosin is approved for treating HTN and benign prostatic hypertrophy (BPH). • Selective postsynaptic α1-receptor antagonist. • 65% bioavailable when taken orally • Peak levels → occur 2-3 hrs after oral admin, effectively relaxing prostatic and vascular smooth muscle. • Metabolism → mainly liver through O-demethylation, and the drug is primarily excreted in the feces. • The terminal elimination half-life of doxazosin → 22 hours, recommended to be taken as a single daily dose in the AM Prazosin • Selective postsynaptic α1-receptor antagonist. • Preserves the inhibitory effect of α2-receptor activity on NE release from nerve endings. • Prazosin has a lower likelihood of causing reflex tachycardia compared to nonselective α-adrenergic antagonists. • It dilates both arterioles and veins. • Onset → 30 mins following oral admin • DOA → approximately 4-6 hrs • Primarily metabolized in the liver Terazosin • α-Blocker therapy for BPH targets α1-mediated innervation of prostatic smooth muscle. • Long-acting α1-adrenergic antagonist Ch. 19: Sympatholytics • • It is administered orally. Terazosin's therapeutic benefit in BPH is attributed to its capacity to relax prostatic smooth muscle. Tamsulosin • α1a-adrenergic antagonist. • It is administered orally. • Indicated for treating S&S of benign prostatic hyperplasia (BPH). • Potential SEs → orthostatic hypotension, vertigo, syncope, and ejaculatory dysfunction. • Clearance of tamsulosin → decreased when administered with cimetidine Alfuzosin • Aelective α1a-adrenergic receptor inhibitor. • Used to treat BPH in younger populations. • Associated with fewer sexual SEs compared to tamsulosin. • Common SEs → dizziness, systemic hypotension, and reflex tachycardia. • Extensively metabolized in the liver into inactive metabolites. • Most of the drug (about 3:1 ratio) is excreted in the bile, with only 11% excreted unchanged in the urine. Sildosin • • • • • • • Highly selective α1a-adrenergic receptor antagonist. Primarily used for the treatment of BPH. Exhibits prostate-specific activity and fewer systemic SEs Rapid oral absorption with only 32% bioavailability. Subject to hepatic metabolism through two pathways. Primary metabolite is active but has only 50% of the parent compound's activity. The metabolites are excreted with a 3:2 ratio, favoring hepatic excretion over renal excretion. Tolazoline • Competitive, nonselective α-adrenergic receptor antagonist. • Historically used for treating persistent pulmonary HTN of the newborn (now replaced by nitric oxide). • SEs → systemic hypotension, reflex tachycardia, cardiac dysrhythmias, and pulmonary/gastrointestinal hemorrhages. • Primarily excreted unchanged by the kidneys. Ch. 19: Sympatholytics Alpha-2 Adrenergic Receptor Agonists • • α2-adrenergic receptor agonists → selectively bind to presynaptic α2-adrenergic receptors. Negative feedback mechanism reduces NE release from presynaptic nerve terminals → leading to decreased sympathetic outflow and BP • Most α2 receptors → located in the CNS, particularly in the brainstem and locus ceruleus. • Peripheral α2 receptor inhibition can affect insulin release and induce glucagon from the pancreas. • Clinical effects → hypotension, bradycardia, central sedation, and mild analgesia, all related to sympatholytic effects. • A-2 stimulation → INHIBITS NE release • decreased NE in synaptic cleft • drugs: clonidine, dexmedetomidine Mechanism of Action • α2-adrenergic receptor agonists selectively target α2-adrenergic receptors in a competitive manner. • Binding of α2 agonists can be displaced from CNS binding sites → leading to a reversal of CNS effects. • Withdrawal from even short-term use → can trigger a rebound effect, causing a significant increase in sympathetic outflow, resulting in elevated HR and potentially dangerous HTN • Centrally-acting alpha-2 agonists: • Spread throughout the central and peripheral nervous system. • Locations: • Pontine locus coeruleus • Medullospinal tract • Rostral ventrolateral medulla • Dorsal horn of the spinal cord • When stimulated, cause neuromodulation: • Sedation, • Analgesia, • Vasodilatation • Bradycardia • Little effect on the respiratory drive, which accounts for their good safety profile. • All of these are a result of decreased NE in the synaptic cleft. • The 2 major drugs in this group are clonidine and dexmedetomidine. • Anesthesia implications: • Sedation for minor procedures • Adjuvant to general and regional anesthesia • Analgesia • Premedicating agents • Precedex is potent and highly selective for alpha 2 receptors (1,620/1); Clonidine is 220/1 – know these numbers; dex has higher affinity for a2 than clonidine • Precedex Effects: • Sedation • goal: comfortable, calm pt who is arousable and cooperative • pt who is NOT arousable should have dose reduced • arousability → test for appropriate sedation (EEG, BIS) • if pt too awake, give more. • dose-dependent • minimal resp. depression • known action: • hyperpolarization of LC neurons • alpha-2A receptor subtype • resembles normal sleep • reversible → atipamezole • amnesia → questionable → but may cause upper airway obstruction • synergistic with other sedatives • Hemodynamics • combination of effects mediated by: Ch. 19: Sympatholytics • • • reduction of central SNS activity (alpha-2A) • reduction of presynaptic NE release (alpha-2A and C) • stimulation of VSM cells (alpha-2B) • stimulation of endothelium • stimulation of central imidazole receptors • some vagomimetic activity → leads to bradycardia, decreased BP • HR effects • bradycardia requiring tx • heart block (no catecholamines?) Effects of alpha-2 agonists: • endocrine • dec NE release • dec insulin release • dec cortisol release • inc GH release • baroreceptors stay intact • normal response to vasoactive drugs • attenuates stress response • ventilation • minimal effect on RR, Ve, PaCO2 • small dec in Ve/ETCO2 • no potentiation of opioid-induced resp. depression • sedation → upper airway obstruction • irregular RR with large boluses • BP can increase when you give dex, because vasoconstriction may dominate. Reduce dose or change the drug. • propofol, GS, epidurals → all reduce SNS activity and tone • HR effects • 20% reduction in HR • tx of bradycardia: • normal response to atropine and glycopyrrolate • be cautious of coronary perfusion • sinus pause/arrest • no effect on ICP • reduces IOP • no effect on relaxants • prolongs local anesthetic actions • dec metabolism • dec O2 consumption • dry mouth • dec bowel motility • dec psychomotor performance • not amnestic • slows EEG • prevents opioid induced rigidity (chest wall rigidity when giving fentanyl too fast) • neuro/cardiac protection • orthostatic hypotension Dosing (dexmedetomidine only) • loading dose: 0.5-1 mcg/kg over 10 min • infusion: 0.2-0.7 mcg/kg/hr • onset: 5-10 min • offset: 30-60 min • wean; don’t abruptly stop • Ch. 19: Sympatholytics Clonidine • Clonidine admin leads to dose-dependent reductions in HR & BP • Clinically used for treating resistant HTN, tremors a/w central stimulant medications, and opioid withdrawal. • Partial agonist of α2 receptors with a preference ratio of 400:1 for α2 over α1 receptors. • Available in IV, oral, and transdermal • Primarily metabolized in the liver but is mainly excreted unchanged in the urine and, to a lesser extent, in the bile and feces. • The terminal half-life of clonidine ranges from approx 12-16 hrs but can be highly variable in individuals with liver or kidney dysfunction Dexmedetomidine • Selective α2 agonist with a strong preference for α2-receptors (1,600:1). • Primarily admin IV as an infusion → 0.1 to 1.5 μg/kg per min • Relatively short terminal elimination half-life → 2 hrs • Commonly used in ICU & OR as a sedative and analgesic d/t its central sympatholytic effects. • Liver metabolism plays a significant role in its biotransformation, and it is primarily excreted in the urine. • Liver impairment → increase plasma levels and extend the DOA due to reduced metabolism during infusion • Lead to physiological dependence, resulting in withdrawal symptoms, such as tachycardia, HTN, and anxiety, after just a few days of admin • Interestingly, large IV boluses (0.25-1 μg/kg over 3-5 mis) → can induce paradoxical HTN with a decrease in HR, resembling the effect of phenylephrine due to α1 stimulation. Ch. 19: Sympatholytics Beta-Adrenergic Receptor Antagonists (Beta Blockers) • • • Selectively bind to β-adrenergic receptors → blocking the effects of catecholamines and sympathomimetics on the heart, airways, and blood vessels. Continuation of β-antagonist therapy is recommended throughout periop period → to maintain desired drug effects and prevent SNS hyperactivity (rebound) associated with abrupt discontinuation. Propranolol → serves as the standard β-adrenergic antagonist against which other β-adrenergic antagonists are often compared. 1st Generation (Nonselective; Block B1 and/or B2) •Timolol •Propanolol (Inderal) •Sotalol 2nd Generation (Selective; B1) •Esmolol Mechanism of Action •Metoprolol • β-Adrenergic receptor antagonists → have selective affinity for •Atenolol β-adrenergic receptors and act through competitive inhibition. •Acebutolol • Competitive antagonism results in a rightward shift of the dose-response •Bispropolol curve for the agonist, but the slope of the curve remains unchanged, 3rd Generation (Nonselective: B1, B2, and/or allowing a full pharmacologic effect with sufficiently large doses of the Alpha 1) agonist. •Labetolol (safe in pregnancy) • Chronic use of β-adrenergic antagonists → lead to an increase in the •Carvedilol #of β-adrenergic receptors, necessitating dose adjustments to maintain desired drug effects. • β-Adrenergic receptors → GPCRs that are stimulated by agonists like epi & NE • Agonist binding to β-adrenergic receptors activates G proteins, which, in turn, stimulate adenylate cyclase to produce cyclic adenosine monophosphate (cAMP). • cAMP activates protein kinases, leading to the phosphorylation of proteins such as L-type voltage-dependent calcium channels and troponin C. • In the heart, β-adrenergic stimulation results in positive chronotropic (heart rate), inotropic (contractility), and dromotropic (conduction) effects, along with negative lusitropic (relaxation) effects and inadvertent positive bathmotropic (excitability) effects. • These responses are blunted by β-adrenergic receptor antagonists (beta-blockers), with the majority (about 75%) of β receptors in the myocardium being β1 receptors, while the remaining 20% are β2 receptors. • beta-1: Gs → adenyl cyclase → cAMP • beta-1: heart, kidneys, adipose tissue • main action: heart • increases HR: beta-1 receptors on SA and AV nodes • beta-1 agonists directly increase HR • increases contractility • increases conduction • kidney: JG cells (release renin) • if blocking beta-1, what are the targets? • slows HR—inhibits b1 stimulation on SA and AV nodes • arrythmias—Afib/flutter → beta-1 blocker will slow it down (good for SVTs) • decrease contractility— for LVH, hx of MI, anything that increases myocardial O2 demand • b1 blockers will decrease myocardial O2 demand • kidney: decreasing renin release, thus decreasing BP • Esmolol • short-term tx; esmolol is very short acting • Metoprolol • more long-acting • pts will likely be on it • 1st Gen Nonselective B-blockers: • beta-2: bronchodilator, vasodilates skeletal muscle vessels, gallbladder relaxation, Na+/K+ pump (stimulates pump) • beta-2 blockers: • sotalol—arrhythmias • timolol—eye drops • propranolol Ch. 19: Sympatholytics • thyroid storm: upregulated b1 receptors or increased sensitivity • hypertensive crisis, increased JG release of renin (b1) • b2 blocker will decrease sensitization in the heart (propranolol) • propranolol → thyroid storm • liver dz: liver receives blood from splanchnic circulation • portal HTN causes splanchnic backpressure → varices • want to constrict these vessels (blocking b2 → constriction) • propranolol for variceal tx • migraine prophylaxis—propranolol • nonselective b-blockers • pain receptors near dura mater can be mechanically stimulated if vessels are dilated in dura. Want to constrict these vessels, so they won’t mechanically stimulate the pain receptors. • beta-2 agonist → tremor, dilate • beta-2 blocker/nonselective a-blocker → stops tremors • beta-2 blocker → constrict; hyperkalemia; hypoglycemia • why give b-blocker? inc HR, BP • check CBG • 3rd Gen: nonselective b1, b2, a1 • labetolol (Trandate) → will give frequently • carvedilol (Coreg): non-decompensated HF to dec myocardial O2 demand • in cart: • esmolol (Brevibloc) • labetolol • esmolol: for short-acting needs; provides a good start Structure-Activity Relationships • β-Adrenergic antagonists → derived from the β agonist drug isoproterenol. • The chemical structure of these drugs, specifically substitutions on the benzene ring → determines whether they act as antagonists or agonists at β-adrenergic receptors. • Levorotatory (left-handed) forms of β antagonists and agonists → more potent than their dextrorotatory (right-handed) counterparts. • For instance, the dextrorotatory isomer of propranolol is significantly less potent (less than 1%) at blocking β-adrenergic receptors compared to the levorotatory isomer. Classification • β-Adrenergic receptor antagonists are categorized into 2 main groups: • Nonselective for β1 and β2 receptors (propranolol, nadolol, timolol, pindolol) • Cardioselective for β1 receptors (metoprolol, atenolol, acebutolol, betaxolol, esmolol, bisoprolol, nebivolol). • The degree of β-receptor selectivity → vary with dosage, and high doses of an antagonist may lead to a loss of selectivity. • These drugs are also classified as partial or pure antagonists, depending on whether they possess intrinsic sympathomimetic activity. • Cardioselective β-adrenergic antagonists → suitable for pts with asthma and reactive airway disease → less effect on peripheral β2 receptors responsible for vasodilation. • β1-receptor blockade results in several cardiac effects, including reduced HR, slower conduction through the AV node, decreased inotropy (contractility), increased lusitropy (ability to relax), and generally reduced bathmotropy (ability to initiate electrical impulses). These effects are more prominent during physical activity. • These changes lead to a decrease in myocardial O2 demand, reducing the risk of MI during exercise • The slowed HR also increases diastolic perfusion time, potentially improving myocardial blood flow. • β2-receptor blockade can increase the risk of bronchospasm in individuals with reactive airway disease and worsen symptoms of peripheral vascular disease. • β-adrenergic antagonists may have a membrane-stabilizing effect on the heart, similar to class I antiarrhythmic drugs like quinidine • However, this effect is only observed at much higher plasma concentrations than those required for adequate βadrenergic blockade. Ch. 19: Sympatholytics Pharmacokinetics • β-adrenergic receptor antagonists vary in their pharmacokinetics → primarily elimination half-time • Esmolol has a brief half-time of about 10 mins, while other drugs have longer durations. • Understanding elimination half-time → crucial in determining dosing intervals in the periop setting or when switching to a different β-adrenergic receptor antagonist. • Propranolol and nebivolol → are the only β-adrenergic receptor antagonists that are highly protein-bound • They have high Vd and rapid distribution after IV admin • These drugs are eliminated through various pathways, and their elimination should be considered when pts have renal or hepatic dysfunction. • Therapeutic plasma concentrations can vary significantly among these drugs and among patients, with multiple factors contributing to interpatient variability, including differences in baseline sympathetic nervous system activity, flat doseresponse curves, the impact of active metabolites, and genetic variations in β-adrenergic receptors that affect individual responses to the drug and plasma concentrations. Heart • Coronary Heart Disease • Arrythmias • Acute Rate control: Afib and Aflutter • Chronic- Prevention of tachyphylaxis • Hypertension • Heart Failure (compensated) • Aorta • Dissection • Aneurysm Vascular System • Alpha 1 Blocker- Dilation- Increased BF- Decreased total PVR and Afterload • Labetolol and Carvedilol Brain and Spinal Cord • Anxiety- Decreases SNS • Migraines • Blocks central tremors Eye • Aqueous Humor Production- Decreases • Timolol- Glaucoma Thyroid • Increased thyroid hormone increase SNS sensitivity (B1 and alpha 1) No BB with Cocaine use or Pheochromocytoma due to unopposed alpha blockade. Heart: • b1 is cardioselective • dec myocardial O2 demand and HR in: CAD, rate control (Afib/flutter) • compensated HF: body trying to maintain for its losses, can still get adequate CO & SV • decompensated HF: don’t mess w/ HR and SV, betablockers contraindicated • aorta: dissection or aneurysm → give BB Ch. 19: Sympatholytics Beta-Blocker Side Effects: Target Organ Systems • Heart • Heart block • Hypotension • Orthostasis with 3rd generation • why? alpha antagonism → vasodilation • Lungs • B2 block- bronchospasm or bronchoconstriction (Contraindicated with • don’t give B2 blockers to asthma, COPD pts—test question • avoid 1st & 3rd gen BBs • GI • Increases GI motility (diarrhea) • Vascular • High TG • B2 supply to muscle and brain tissue- slight drop in supply- fatigue • CNS • Blunting leads to nightmares, insomnia, ED • Pancreas • Increased blood glucose • Glucose unawareness • Treatment of Overdose • Fluids • Atropine (faster than glycopyrrolate) • Glucagon asthma or COPD) Propranolol • Nonselective β-adrenergic receptor antagonist with no intrinsic sympathomimetic activity, making it a pure antagonist. • It equally antagonizes both β1 and β2 receptors. • Propranolol → reference standard against which other β-adrenergic antagonists are often compared. • When administering propranolol, dosing is typically increased incrementally until the desired physiologic plasma concentrations are achieved, as evidenced by a resting HR of 55- 60 bpm Cardiac Effects • Primary effects are on the heart. • It decreases HR and myocardial contractility d/t β1-receptor blockade, leading to reduced CO • These effects are especially noticeable during exercise or in the presence of increased sympathetic activity. • HR reduction by propranolol lasts longer than the negative inotropic effects, indicating potential distinctions in β1 receptors. • Simultaneous β2-receptor blockade increases PVR, including coronary vascular resistance. • Propranolol may paradoxically increase systolic ejection time and ventricular dilatation, which can raise myocardial oxygen demand. • Nevertheless, the drug's oxygen-sparing effects due to HR and contractility reduction generally outweigh these changes. • This means propranolol can alleviate myocardial ischemia, despite increased coronary vascular resistance. • Na retention a/w propranolol therapy → linked to intrarenal hemodynamic changes resulting from reduced CO Pharmacokinetics • Propranolol → absorbed quickly and almost entirely from the GI tract. • However, extensive hepatic first-pass metabolism limits its systemic availability, accounting for up to 90-95% of the absorbed dose. • Significant individual variation exists in the extent of hepatic first-pass metabolism, leading to considerable differences in plasma concentrations among patients receiving similar oral doses. • Due to hepatic first-pass metabolism, the oral dose of propranolol (ranging from 40-800 mg per day) needs to be much higher than the IV dose (0.05 mg/kg, given in 0.5-1.0 mg increments every 5 minutes). • Propranolol → not effective IM Protein Binding • Propranolol exhibits extensive binding to plasma proteins (90-95%). • Increases in free fatty acids, often induced by heparin → lead to reduced plasma protein binding of propranolol. Ch. 19: Sympatholytics • Hemodilution during cardiopulmonary bypass can also impact drug protein binding due to changes in protein concentration within the pump prime. Metabolism • Propranolol primarily cleared from the plasma through hepatic metabolism. • After oral admin of propranolol, an active metabolite → called 4-hydroxypropranolol is present in the plasma, potentially contributing to its effects. • Elimination half-time for propranolol → 2-3 hrs, and that of 4-hydroxypropranolol is even shorter. • There is no clear correlation btw the plasma concentration or total dose of propranolol and its therapeutic effects. • Additionally, propranolol assays may not detect 4-hydroxypropranolol. • Decreases in hepatic blood flow can significantly reduce the elimination of propranolol • Propranolol itself may reduce its clearance rate by lowering CO and hepatic blood flow • Changes in hepatic enzyme activity can also impact metabolism. • Renal failure does not significantly affect the elimination half-time of propranolol, but it may lead to the accumulation of metabolites. Clearance of Local Anesthetics • Propranolol reduces the clearance of amide local anesthetics by affecting hepatic blood flow and inhibiting liver metabolism. • This decrease in clearance is more significant (46%) than what would be expected from a maximum 25% reduction in hepatic blood flow • This suggests that propranolol also affects drug metabolism in the liver. • Bupivacaine clearance, which is a low-extraction drug → relatively insensitive to changes in hepatic blood flow. • Consequently, the 35% decrease in bupivacaine clearance with propranolol is attributed to reduced metabolism. • The clearance of drugs with low extraction ratios is inversely related to plasma protein binding • Propranolol may increase the binding of bupivacaine to α1-acid glycoprotein, responsible for 90% of bupivacaine binding, which could explain the clearance reduction. However, propranolol doesn't alter α1-acid glycoprotein concentrations. • Propranolol pretreatment can lead to increased serum concentrations of mepivacaine, suggesting that propranolol and other β antagonists may enhance systemic toxicity of certain amide local anesthetics. Clearance of Opioids • Chronic propranolol treatment → reduces pulmonary first-pass uptake of fentanyl. • This leads to a significantly greater amount of injected fentanyl entering the systemic circulation shortly after admin • The interaction btw these 2 drugs → due to the inhibitory effect of propranolol, a basic lipophilic amine, on the pulmonary uptake of fentanyl, which is also a basic lipophilic amine. Nadolol & Pindolol • Non-selective beta-antagonists (beta-blockers) • Nadolol—long DOA; 1x daily admin Pharmacokinetics • Nadolol has slow and incomplete absorption from the GI tract, estimated at around 30%. • It undergoes minimal metabolism, with approximately 75% of the drug excreted unchanged in the urine and the rest in bile. • Nadolol exhibits wide individual variations in plasma concentrations, not due to differences in metabolism. • It has a long elimination half-time of 20-40 hours, allowing for once-daily dosing. • Pindolol, on the other hand, has a shorter elimination half-time (3 to 4 hours), but this duration can be prolonged to over 11 hours in patients with renal failure. Timolol • Nonselective β-adrenergic receptor antagonist that is as effective as propranolol • Effective in the tx of glaucoma → b/c its ability to decrease IOP, presumably by decreasing the production of aqueous humor • Administered as eyedrops in the tx of glaucoma, but systemic absorption can cause bradycardia & increased airway resistance • Indeed, bradycardia and hypotension that are refractory to tx with atropine have been observed during anesthesia in pediatric and adult patients receiving topical timolol with or without pilocarpine • Associated with impaired control of ventilation in neonates → resulting in unexpected postop apnea o Immaturity of the neonate’s BBB may facilitate access of this drug to the CNS. Ch. 19: Sympatholytics Pharmacokinetics • Rapidly and almost completely absorbed after oral admin • Extensive first-pass hepatic metabolism limits the amount of drug reaching the systemic circulation to about 50% of that absorbed from the GI tract • Protein binding → not extensive • Elimination half-time → 4 hrs Metoprolol • Selective β1-adrenergic receptor antagonist → prevents inotropic and chronotropic responses to β-adrenergic stimulation • Conversely, bronchodilator, vasodilator, and metabolic effects of β2 receptors remain intact such that metop → less likely to cause adverse effects in pts with chronic obstructive airway disease or PVD and in patients vulnerable to hypoglycemia • It is important to recognize, however, that selectivity is dose related, and large doses of metoprolol are likely to become nonselective, exerting antagonist effects at β2 receptors as well as β1 receptors • Can increase airway resistance in asthmatics treated with metoprolol, but less than propranolol • Metoprolol-induced increases in airway resistance → more readily reversed with β2 -adrenergic agonists (terbutaline) Pharmacokinetics • Readily absorbed from the GI tract, but this is offset by substantial hepatic first-pass metabolism such that only about 40% of the drug reaches the systemic circulation • Low protein binding is low; estimated to account for about 10% of the drug • No active metabolites • A small amount (<10%) of the drug appears unchanged in urine • 2 available oral formulation of metoprolol → metoprolol tartrate and metoprolol succinate • Metoprolol tartrate elimination half-time of → 2-3 hrs and correlates to a need for at least a twice daily dosing strategy with a 3 or 4 times daily strategy providing a more reliable control of HR • Metoprolol succinate → significant extended time to peak concentrations and overall decreased plasma concentrations compared to metoprolol tartrate at equal daily doses • Metoprolol succinate elimination half-time → 5-7 hrs and used in once daily dosing regimens but in some pts can still result in β-blocker withdrawal tachycardia at 24 hours necessitating twice daily dosing • Overall, plasma concentrations of metoprolol do not correlate with therapeutic effects of the drug Atenolol • Even more selective β1-adrenergic antagonist that may have specific value in pts in whom the continued presence of β2receptor activity is desirable. • In patients at risk for CAD who must undergo noncardiac surgery → tx with IV atenolol before and immediately after surgery, followed by oral therapy during the remainder of the hospitalization, decreases mortality and the incidence of CV complications for as long as 2 years • Periop admin of atenolol to pts at high risk for CAD → decreases the incidence of postop myocardial ischemia • Antihypertensive effect of atenolol → prolonged, permitting this drug to be admin once daily for the tx of HTN • Like nadolol, atenolol does not enter the CNS in large amounts, but fatigue and mental depression still occur • Unlike nonselective β-adrenergic antagonists → atenolol does not potentiate insulin-induced hypoglycemia and can thus be admin with caution to pts with DM whose HTN is not controlled by other antihypertensives Pharmacokinetics • About 50% of an orally administered dose is absorbed from GI tract • Peak concentrations occurring 1-2 hrs after oral admin • Undergoes little or no hepatic metabolism and is eliminated primarily renally • Elimination half-time → 6-7 hrs; this may increase to more than 24 hrs in pts with renal failure Betaxolol: • Cardioselective β1-adrenergic antagonist. • No intrinsic sympathomimetic activity and weak membrane-stabilizing activity. • High doses have β2-adrenergic antagonist effects on bronchial and vascular smooth muscle. • Nearly complete absorption after oral dose. • Long elimination half-time (11 to 22 hours). • Primarily cleared by metabolism, with less renal elimination. • Useful for HTN Ch. 19: Sympatholytics • Topical preparation for chronic open-angle glaucoma. • Lower risk of bronchoconstriction in airway hyperreactivity compared to timolol. Bisoprolol: • Highly β1-selective antagonist. • No significant intrinsic agonist activity. • Elimination half-time → 9-12 hrs • Equally eliminated by renal and nonrenal mechanisms. • Pharmacologically inactive metabolites. • Prominent negative chronotropic effect. • Used for essential HTN and improves survival in mild to moderate congestive heart failure. Nebivolol: • Extremely potent and selective β1-antagonist. • No significant intrinsic agonist activity. • May exhibit low β2-antagonism at high doses or in patients with specific cytochrome P450 polymorphisms. • Elimination half-time → 12-19 hrs • Once-daily regimen with both renal and fecal elimination. • Approved for the treatment of essential hypertension. Esmolol: • Rapid-onset and short-acting selective β1-adrenergic receptor antagonist. • Administered only IV • Full therapeutic effect → within 5 mins and action ceases within 10-30 mins after discontinuation. • Useful for preventing or treating intraoperative increases in blood pressure and heart rate in response to noxious stimulation. • Can protect against HR and SBP increases during tracheal intubation when administered IV. • Effective in blunting the increase in SBP associated with laryngoscopy and tracheal intubation. • No significant influence on HR Prevention of Tachycardia and Hypertension: • Esmolol (100-200 mg IV) → prevent periop tachycardia and HTN when administered before anesthesia induction. • Prior admin of esmolol (500 μg/kg per minute IV) → reduces increase in HR during electroconvulsive therapy with methohexital and succinylcholine. • Used in resection of pheochromocytomas and perioperative management of conditions like → thyrotoxicosis, pregnancyinduced HTN, and cardiovascular toxicity from epi or cocaine. • May help in hypertrophic obstructive cardiomyopathy and hypercyanotic spells in ToF Cautions on β-Adrenergic Antagonists: • Administering β-adrenergic antagonists in response to excessive sympathetic nervous system activity (e.g., cocaine or epinephrine-induced) can lead to pulmonary edema and cardiovascular collapse. • Esmolol's β1 selectivity may unmask β2-mediated vasodilation. • Esmolol doesn't produce additional negative inotropic effects in patients chronically treated with β-adrenergic antagonists. Pharmacokinetics: • IV admin only • Rapid hydrolysis by plasma esterases. • Elimination half-time → 9 mins • Limited CNS and placental transfer → due to poor lipid solubility. • No significant impact on succinylcholine DOA • Clinically insignificant amounts of methanol are produced during hydrolysis. Class-Related Side Effects: • β-Adrenergic antagonists have similar side effects across the class. • Impact cardiovascular system, airway resistance, carbohydrate, and lipid metabolism. • May cause hypoglycemia. • Potential additive effects with anesthesia drugs (sedation, bradycardia, hypotension). • Some β-adrenergic antagonists can cross the BBB and placenta. • GI SEs, fever, rash, myopathy, alopecia, thrombocytopenia. Ch. 19: Sympatholytics • Decreased high-density lipoproteins and increased triglyceride and uric acid levels. • Primary CI → preexisting AV heart block or acute non-tachycardia-induced cardiac failure. • Nonselective β-adrenergic antagonists or high doses → not recommended for reactive or obstructive airway disease • May mask signs of hypo- or hyperglycemia in patients with DM CV Effects: • β-Adrenergic antagonists produce negative inotropic (reduced contractility) and chronotropic (decreased heart rate) effects. • Slows the conduction speed (dromotropy) of cardiac impulses through the AV node and decreases the rate of spontaneous phase 4 depolarization. • Preexisting AV heart block may be accentuated by β-adrenergic antagonists. • Mechanism of Action: • CV effects result from the removal of SNS innervation to the heart (β1-blockade). • Membrane stabilization occurs only at high plasma concentrations of the antagonist. • Nonselective β-adrenergic blockade can impede LV ejection due to unopposed α-adrenergic receptor–mediated peripheral vasoconstriction. • The magnitude of CV effects → most pronounced when SNS activity is increased, as in exercise or cardiac failure. • β-adrenergic antagonists attenuate the tachycardia of exercise and may precipitate cardiac failure in some patients. • Specific Effects: • β-adrenergic antagonists prevent inotropic and chronotropic effects of isoproterenol and baroreceptor-mediated HR increases due to peripheral vasodilator drugs. • They do not significantly alter the response to calcium, glucagon, or digitalis preparations. • β-adrenergic antagonists do not modify the response to α-adrenergic agonists such as epi or phenylephrine. • Pressor Effect of Epinephrine: • Nonselective β antagonists enhance the pressor effect of epi because they prevent β2 vasodilation and leave unopposed α-adrenergic effects to predominate. • This can lead to paradoxical HTN and may precipitate cardiac failure in patients with diseased myocardium. • Unexpected HTN may occur in pts receiving clonidine followed by a nonselective β-adrenergic antagonist. • Peripheral Vascular Disease: • Pts with PVD do not tolerate the peripheral vasoconstriction associated with β2-receptor blockade. • SEs can include cold hands and feet, particularly in nonselective β-adrenergic antagonists. • Raynaud disease and vasospasm can be accentuated by certain β-adrenergic antagonists, like propranolol. • Antidysrhythmic Effect: • The primary antidysrhythmic effect of β-adrenergic blockade → prevention of dysrhythmogenic effects of catecholamines and sympathomimetics. • This is due to a decrease in SNS activity. • Membrane stabilization is likely of little importance in the antidysrhythmic effects produced by typical doses of βadrenergic antagonists. • Treatment of Excess Myocardial Depression • Excessive myocardial depression due to β-adrenergic blockade may manifest as → bradycardia, hypotension, cardiogenic shock, bronchospasm, respiratory depression, seizures, and prolonged intraventricular conduction. • Hypoglycemia, though rare, can be life-threatening in overdose. • Treatment Options: • Initial treatment for excessive bradycardia and decreased CO due to β-blockade → atropine, admin in incremental doses of 7 μg/kg IV • Atropine can help by blocking vagal effects on the heart and unmasking residual SNS innervation. • If atropine is ineffective, direct positive chronotropic and inotropic agents are required. • Isoproterenol, a nonselective β-adrenergic agonist, can be administered by continuous infusion at doses sufficient to overcome competitive β-blockade. • In cases of excessive cardiovascular depression caused by pure β-adrenergic antagonists, pure β1-adrenergic agonists like dobutamine are recommended. • Dopamine and epinephrine → not recommended due to potential α-adrenergic–induced vasoconstriction. • Glucagon (1-10 mg IV, followed by 5 mg per hour IV) can effectively reverse myocardial depression induced by βadrenergic antagonists, as it acts independently of β-adrenergic receptors, stimulating adenylate cyclase and increasing cAMP concentrations. Ch. 19: Sympatholytics • • • • • • • Calcium chloride (250-1,000 mg IV) may also act independently of β-adrenergic receptors to offset excessive cardiovascular depression. • Glucagon is particularly effective in life-threatening bradycardia and is the drug of choice to treat massive β-adrenergic antagonist overdose. • In cases of unresponsive, life-threatening bradycardia, the placement of a transvenous PM may be necessary, with the caveat that a massive overdose may raise myocardial thresholds to prevent electromechanical capture. • Hemodialysis should be reserved for patients refractory to pharmacologic therapy when attempting to remove minimally protein-bound, renally excreted β-adrenergic antagonists. Airway Resistance: • Nonselective β-adrenergic antagonists like propranolol increase airway resistance, leading to bronchoconstriction due to blockade of β2 receptors. • This effect is more pronounced in patients with preexisting obstructive airway disease. • Selective β1-adrenergic antagonists (e.g., bisoprolol, metoprolol, esmolol) are less likely to increase airway resistance compared to nonselective antagonists. Metabolism: • Nonselective β-adrenergic antagonists (e.g., propranolol) interfere with glycogenolysis in response to epi during hypoglycemia. • They can blunt the tachycardiac response to hypoglycemia, which is a warning sign in insulin-treated diabetics. • As a result, nonselective β-adrenergic antagonists → not recommended for pts with DM treated with insulin or oral hypoglycemics. • Altered fat metabolism is observed as these antagonists prevent sympathomimetics or sympathetic nervous system stimulation from increasing plasma concentrations of free fatty acids. Distribution of Extracellular Potassium: • Distribution of potassium across cell membranes is influenced by SNS activity, including β2-adrenergic receptors that facilitate intracellular movement of potassium. • β-adrenergic blockade inhibits K+ uptake into skeletal muscles, potentially increasing plasma K+ concentration. • Increases in plasma K+ associated with potassium infusion are greater in the presence of β-adrenergic blockade, as seen with propranolol. • While animal studies suggest that β-adrenergic blockade may prolong increases in plasma potassium after succinylcholine administration, this hasn't been conclusively demonstrated in patients. • Selective β1-adrenergic antagonists may impair skeletal muscle uptake of K+ less than nonselective β-adrenergic antagonists. Interaction with Anesthetics: • Myocardial depression caused by anesthetics may be additive with β-adrenergic antagonists. • Clinical experience and studies in patients and animals suggest that additive myocardial depression with β-adrenergic antagonists and anesthetics is generally not excessive. • Tx with β-adrenergic antagonists can be safely maintained throughout the perioperative period in most cases. • An exception may be patients treated with timolol, as profound bradycardia has been observed in the presence of inhaled anesthetics. • The extent of additive CV effects with inhaled anesthetics and β-adrenergic antagonists varies, with enflurane showing the greatest effects and isoflurane the least. • Sevo and des, like isoflurane, do not appear to be associated with significant additive cardiovascular effects when administered to patients on β-adrenergic antagonists. Opioids and Anesthetics: • Preexisting β-adrenergic blockade does not alter CV responses to opioids such as fentanyl. • Excessive sympathetic nervous system activity, as seen with ketamine or hypercarbia, may unmask negative inotropic effects of concomitantly administered anesthetics, leading to decreased systemic blood pressure and cardiac output. Effects on the Nervous System: • β-Adrenergic antagonists may cross the BBB and produce side effects. • Side effects may include → fatigue, lethargy, vivid dreams, and peripheral paresthesias. • Psychotic reactions are rare, and while memory loss and mental depression have been alleged, they have not been definitively linked to β-adrenergic antagonist therapy. • Review of clinical trials showed no significant increased risk of depressive symptoms, with only small increased risks of fatigue and sexual dysfunction. • Long-term and high-dosage effects on the nervous system are less clear. Ch. 19: Sympatholytics • Some β-adrenergic antagonists, such as atenolol and nadolol, are less lipid-soluble and may be associated with a lower incidence of CNS effects. • Effects on the Fetus: • β‐adrenergic antagonists can cross the placenta and potentially affect the fetus. • Possible fetal effects → bradycardia, hypotension, and hypoglycemia, especially with lipophilic drugs like labetalol and propranolol. • High levels of protein binding in some β‐adrenergic antagonists may reduce their effects on the fetus. • Labetalol is categorized by the US FDA as pregnancy category C, with "potential benefits may outweigh the risks." • The American College of Obstetrician Gynecologists (ACOG) supports the use of labetalol in pregnancy based on longterm clinical use data. • β‐adrenergic antagonists, like atenolol and metoprolol, are excreted into breast milk, with higher levels in hydrophilic compounds and low protein binding. Labetalol and propranolol have lesser excretion into breast milk, and ACOG supports their use during postpartum breastfeeding. • Withdrawal Hypersensitivity: • Acute discontinuation of β‐adrenergic antagonist therapy can result in excess SNS activity, which typically appears within 24-48 hours. • This enhanced activity is thought to be due to upregulation of β‐adrenergic receptors during chronic antagonist therapy. Clinical Uses: • β‐adrenergic antagonists have multiple clinical uses. • In equivalent doses, all β‐adrenergic antagonists are equally effective in producing the desired therapeutic effects. • Pts on β‐adrenergic receptor antagonist therapy should have their medication continued uninterrupted through the periop period. • Pts at high risk for myocardial ischemia and undergoing major surgery → benefit from preop and postop tx with β‐adrenergic receptor antagonists. Treatment of Essential Hypertension: • Chronic therapy with β-adrenergic antagonists gradually decreases SBP • The antihypertensive effect of β-adrenergic blockade is primarily due to decreased HR, resulting in decreased cardiac output. • Large doses of β-adrenergic antagonists may also decrease myocardial contractility. • Systemic vascular resistance often remains unchanged, and orthostatic hypotension is absent. • β-adrenergic antagonists are sometimes used in combination with vasodilators to prevent reflex increases in HRand cardiac output. • All orally administered β-adrenergic antagonists are equally effective antihypertensive drugs. • Nonselective β-adrenergic antagonists, like propranolol, prevent the release of renin from the JG apparatus, contributing to their antihypertensive effect. • β-adrenergic antagonists also prevent compensatory Na+ and water retention that may occur with vasodilator treatment. Management of Angina Pectoris: • Orally administered β-adrenergic antagonists are effective in reducing the likelihood of myocardial ischemia and angina pectoris. Ch. 19: Sympatholytics • • • This effectiveness is achieved by decreasing myocardial oxygen requirements through lowered heart rate and myocardial contractility. An important measure is to reduce the heart rate during exercise to ensure that it does not exceed the threshold for myocardial ischemia. Combining β-adrenergic antagonists with calcium channel blockers, which act on different aspects of the myocardial oxygen supply-to-demand ratio, does not provide greater benefits in clinical studies and may lead to more adverse effects than using a single drug. Treatment of Acute Coronary Syndrome: • Historically, it was recommended that all acute myocardial infarction (AMI) patients receive IV β-adrenergic antagonists as early as possible. • Contraindications for β-blocker treatment in AMI include severe bradycardia, unstable LV failure, and AV heart block. • Relative contraindications include asthma or reactive airway disease, depression, excessive fatigue, and peripheral vascular disease. • β-blockers should not be withheld from pts with DM, but hypoglycemia signs may be masked. • Recent studies have raised concerns about the use of β-blockers in patients presenting with acute coronary syndrome within the first 8 hours, particularly those with ST-elevation myocardial infarction (STEMI) or cardiogenic shock. • The timing of β-blocker administration and the specific clinical scenario should be considered when deciding on treatment. Periop β-Adrenergic Receptor Blockade: • Recommended for patients at risk for MI during high-risk surgery (known CAD, positive preoperative stress tests, diabetes mellitus treated with insulin, LV hypertrophy). • The goal of preoperative therapy → achieve a resting HR btw 65-80 bpm • All β-adrenergic receptor antagonists, except those with intrinsic sympathetic nervous system activity, decrease mortality. • Admin of atenolol before and after noncardiac surgery in high-risk patients may reduce mortality and CV complications for up to 2 years after surgery. • Perioperative β-adrenergic receptor blockade can decrease the incidence of postoperative myocardial ischemia, associated with a reduced risk of death at 24 months. • The mechanism of these beneficial effects is not fully understood. • Preop oral therapy can be initiated with atenolol, bisoprolol, or metoprolol. • Intravenous administration of atenolol, metoprolol, or esmolol may be considered for patients seen on the morning of surgery. • Notable contraindications to β-blocker therapy include severe reactive airway disease, bradycardia, shock, or left ventricular failure. • The choice of β-blocker and the initiation regimen should consider patient-specific factors and avoid overly aggressive dosing. • Initiating low-dose β-blocker regimens preoperatively or postoperatively is preferred by many practitioners. Treatment of Intraoperative Myocardial Ischemia: • β-blockers may be beneficial when evidence of myocardial ischemia is observed intraoperatively. • Suitable drugs include esmolol, metoprolol, atenolol, or propranolol, which should be titrated to achieve the desired heart rate. • Nitroglycerin is often added to this treatment regimen to supplement its effects. Suppression of Cardiac Dysrhythmias: • β-blockers are effective in controlling dysrhythmias related to SNS stimulation (e.g., thyrotoxicosis, pheochromocytoma, perioperative stress). • They are useful for managing ventricular and atrial dysrhythmias, including atrial fibrillation, atrial flutter, and torsades de pointes. • Acebutolol, metoprolol, atenolol, propranolol, and timolol are approved for the prevention of sudden death following acute myocardial infarction. Management of Congestive Heart Failure: • Controlled studies have shown that metoprolol, carvedilol, and bisoprolol improve ejection fraction and increase survival in patients with chronic heart failure. • Initial doses of β-blockers for heart failure treatment should be minimal and gradually increased. Ch. 19: Sympatholytics Prevention of Excessive Sympathetic Nervous System Activity: • β-blockers are used to attenuate HR and BP changes in response to various stressors. • They can effectively manage conditions such as hypertrophic obstructive cardiomyopathies, pheochromocytoma, hyperthyroidism, tetralogy of Fallot, and anxiety states. • Intraoperatively, propranolol has been used to prevent reflex baroreceptor-mediated heart rate increases during vasodilatorinduced controlled hypotension. Preoperative Preparation of Hyperthyroid Patients: • Thyrotoxic patients c