Ceramics Bonding PDF
Document Details
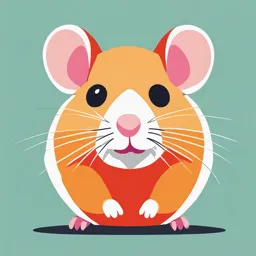
Uploaded by AstoundedAstrophysics
Prof. Muawia Qudeimat, Dr. Nadam Mustafa
Tags
Summary
This document provides an overview of bonding protocols for resin-bonded dental ceramics. It covers influencing factors for clinical success including ceramic properties, tooth preparation design, and bonding principles. The document also discusses surface treatment methods, bonding mechanisms, and the importance of selecting appropriate resin cements.
Full Transcript
Protocols for Resin- Bonded Dental Ceramics P R O F. M U A W I A Q U D E I M A T D R. N A DA M O U S TA FA Introduction Clinical success of resin-bonded ceramic restorations is influenced by the ceramic material physical, mechanical and optical properties, tooth preparation design, impression...
Protocols for Resin- Bonded Dental Ceramics P R O F. M U A W I A Q U D E I M A T D R. N A DA M O U S TA FA Introduction Clinical success of resin-bonded ceramic restorations is influenced by the ceramic material physical, mechanical and optical properties, tooth preparation design, impression technique, fabrication process, definitive insertion protocol, and bonding protocol. Influencing Factors for Clinical Success Ceramic Material Properties: Physical and optical characteristics significantly affect restoration outcomes. Tooth Preparation Design: Proper design enhances bonding effectiveness. Impression Technique: Accuracy in impressions is crucial for fit and function. Fabrication Process: The method used in creating restorations impacts longevity and performance. Insertion Protocol: Definitive insertion methods influence clinical success. Definitions: When the molecules of one substrate adhere or are attracted to molecules of the other substrate, the force of attraction is called adhesion when unlike molecules are attracted, and it is called cohesion when the molecules involved are of the same kind. The material that is used to cause adhesion or bonding is known as the adhesive, and the material to which the adhesive is applied is called the adherend. In a broad sense, bonding is simply a surface-attachment process, which is usually qualified by specifying the type of intermolecular attraction that may exist between the adhesive and the adherend. Definitions: Importance of Bonding Bonding and adhesion comprise a complex set of physical, chemical, and mechanical mechanisms that allow the attachment and binding of one substance to another. A dental bonding system performs three essential functions: (1) provides resistance to separation of an adherend substrate (i.e., enamel, dentin, metal, composite, ceramic) from a restorative or cementing material; (2) distributes stress along bonded interfaces; and (3) seals the interface via adhesive bonding between dentin and/or enamel and the bonded material, thus increasing resistance to microleakage and decreasing the risk for postoperative sensitivity, marginal staining, and secondary caries. Principles in Dental Bonding The bonding principles in dental ceramics focus on establishing a strong and durable adhesion between ceramic materials and the tooth or prosthetic substrate. Bonding is crucial to ensure longevity, aesthetic quality, and function. 1. Surface preparation 2. Chemical Bonding 3. Resin Cement Selection 4. Surface Conditioning of the Tooth or Core Substrate 5. Stress management Principles in Dental Bonding 1. Surface preparation Ceramic Etching: Ceramics, especially glass-based ceramics like lithium disilicate (e.g., IPS e.max), require etching with hydrofluoric acid (HF) to create microporosities on the surface. This process increases the surface area, enhancing the mechanical interlocking of the resin cement. Air Abrasion: Non-etchable ceramics, such as zirconia (e.g., Nexxzr Sagemax CAD CAM zirconia), benefit from air abrasion with aluminum oxide particles. This roughens the surface to increase retention. Principles in Dental Bonding 2. Chemical Bonding Silane Coupling Agent: For silica-based ceramics, applying a silane coupling agent after etching promotes chemical bonding by forming a molecular bridge between the resin and the ceramic (Dual functionality). Silane can bond effectively with the hydroxyl groups on the ceramic surface. Phosphate Monomers: For zirconia and alumina ceramics, phosphate monomers like MDP (methacryloyloxydecyl dihydrogen phosphate) in primers or cements establish a chemical bond to the ceramic without the need for HF etching. Principles in Dental Bonding 3. Resin Cement Selection Light-Cure vs. Dual-Cure: Light-cure resin cements are suitable for thin ceramic restorations where light can penetrate fully, while dual-cure cements are needed for thicker, opaque ceramics that prevent complete light penetration. Resin-Infiltration and Polymerization: Once the resin cement is applied, it flows into the surface irregularities and polymerizes to create a solid, interlocking matrix that bonds the ceramic to the tooth or core. Principles in Dental Bonding 4. Surface Conditioning of the Tooth or Core Substrate For enamel, etching with phosphoric acid is standard, while for dentin, a primer may be used to ensure adequate adhesion. In cases of bonding to metal or composite cores, surface conditioning may involve sandblasting and applying metal primers. Principles in Dental Bonding 5. Stress Management Effective bonding helps to distribute occlusal forces, reducing the risk of fracture. Proper adhesive techniques also help avoid microleakage and detachment, enhancing restoration longevity. Bonding Requirements 1. Surface cleanliness 2. Surface energy 3. Surface roughness 4. Adhesive viscosity 5. Adequate setting of adhesive Bonding Requirements Surface Cleanliness: A clean, contaminant-free surface has high surface energy, which improves wettability and adhesion. Contaminants (e.g., saliva, blood) reduce surface energy, weakening the bond by preventing the adhesive from spreading evenly. (Clean surface ->high energy -> high wettability) Surface Energy: High surface energy promotes better adhesive wettability, leading to stronger, more consistent bonding. It’s enhanced by cleaning and surface preparation techniques (mechanical/chemical/chemo-mechanical), which allow the adhesive to establish a stronger mechanical and chemical bond. Surface Roughness: Roughening the ceramic surface (e.g., by etching or sandblasting) creates micro-retentive areas for better mechanical interlocking, enhancing bond strength and durability. Smooth surfaces reduce bonding potential. Bonding Requirements Adhesive Viscosity: Optimal viscosity allows the adhesive to flow into surface irregularities. Too viscous adhesives may not penetrate well, while too thin adhesives may run off, both of which can weaken the bond. Adequate Setting of Adhesive: Proper curing ensures full polymerization, maximizing bond strength and preventing premature failure. Insufficient curing leaves uncured areas prone to breakdown, compromising adhesion and durability. Q&A Importance of Bonding ? Importance of Bonding Protocols Adhesion Facilitation: Proper bonding ensures the restorative material adheres effectively to the tooth. Marginal Adaptation: Enhances the fit between restoration and tooth structure. Microleakage Prevention: Reduces the risk of fluid and bacteria ingress. Increased Fracture Resistance: Improves durability of both the tooth and restoration. Dual Bonding Mechanism Interfaces to Consider: Tooth interface Restorative material interface Micromechanical Interlocking: Achieved through the use of priming agents and silane coupling agents. Chemical Bonds: Essential for various dental ceramics to ensure durability and performance. Composition and Longevity of Resin Cements Composition: Typically includes bis-GMA or urethane dimethacrylate resin matrix and 20%-80% inorganic fillers. Classification: Resin-Modified Glass Ionomer Composite Resin Cements Activation Modes: Light-activated, dual-activated, and autoactivated. Bonding Techniques: With Pretreatment: Total and self-etch adhesive systems. Without Pretreatment: Self-adhesive systems. Surface Pretreatment Methods Definition: Enhancements to surface topography at a microscopic level. Common Methods: Acid etching Mechanical roughening Cleaning and debris removal Selection Criteria: Based on type, composition, and mechanical properties of ceramics. Surface Pretreatment Methods Surface treatment methods can be: - Mechanical: acid etching, airborne particle abrasion, laser irradiation, or grinding with diamond rotary instruments - Chemical: silane agents, such as ceramic primers, or universal adhesives with silane and phosphate coupling agents - Chemo-mechanical: tribochemical silica coating (silicatization) uses silica-coated alumina particles followed by silanization Historical Development of Ceramics in Dentistry Early Innovations: 18th Century: Alexis Duchateau used porcelain for dentures. Late 1800s: Richmond developed a porcelain crown fused to a metal post. 1889: Charles Land introduced all-porcelain jacket crowns. Challenges: Initial crowns had low mechanical strength, leading to high failure rates. Ceramic Classifications Microstructure-Based Classification: Silica-based ceramics Resin-matrix ceramics Oxide ceramics Advancements: New fabrication processes and digital technologies broadened applications (inlays, onlays, veneers, etc.). Bonding Predictability: High glass content in silica-based ceramics allows for predictable bonding. Oxide ceramics require distinct bonding protocols for effective long-term adhesion. Bonding to Silica-Based Ceramics- Composition Silica-based ceramics are nonmetallic inorganic amorphous materials that predominantly consist of a glass phase, which provides translucency and aesthetic qualities. They are classified into three categories: Traditional Feldspathic Ceramics: Composed of feldspar and quartz. Leucite-Reinforced Glass Ceramics: Contain leucite crystals that enhance strength. Lithium Silicate Ceramics: Incorporate lithium disilicate, offering superior strength and esthetics. Bonding to Silica-Based Ceramics- Adhesive Bonding Necessity Due to their inherent brittleness and low mechanical properties, adhesive bonding is crucial to enhance the durability of these ceramics. Bonding to Silica-Based Ceramics- Bonding Process HF Acid Etching: Hydrofluoric acid (HF) is used to selectively dissolve the glass phase, exposing the silicon dioxide (SiO₂) and creating microretentive surfaces. The etching time and concentration of HF depend on the ceramic’s glass and crystalline content. Surface Topography: Acid etching leads to increased roughness, facilitating micromechanical interlocking with resin cements. Bonding to Silica-Based Ceramics- Silane Coupling Agent Following etching, a silane coupling agent is applied to form chemical bonds between the ceramic surface and resin cements. This enhances adhesion due to the silane’s ability to create a covalent bond with both Application of a silane coupling the inorganic ceramic and the organic agent resin. Bonding to Silica-Based Ceramics- Silane Coupling Agent The chemical reaction between a silane coupling agent and an etched (or activated) glass ceramic surface is crucial in bonding silica-based ceramics, such as lithium disilicate, to adhesives and resin cements. This reaction creates a durable chemical bond that enhances the longevity and stability of the restoration. Here’s how the reaction works: 1. Surface Activation (Etching) of the Glass Ceramic 2. Application of Silane Coupling Agent 3. Hydrolysis of Silane Molecule 4. Condensation Reaction with Ceramic Surface 5. Polymerization with Resin Cement 1. Surface Activation (Etching) of the Glass Ceramic The glass ceramic surface is etched with hydrofluoric acid (HF), which creates microporosities and increases surface roughness. This step also exposes silica (SiO₂) groups on the ceramic surface, making it reactive and ready for bonding. After etching, the ceramic is thoroughly rinsed to remove any residual acid and dried. This high-energy, rough surface promotes strong adhesion. 2. Application of Silane Coupling Agent Silane coupling agents, such as 3- methacryloxypropyltrimethoxysilane (MPS), are applied to the etched ceramic surface. Silane is a bifunctional molecule with two different reactive ends: Silicon (Si)-containing groups (usually -Si(OCH₃)₃) at one end, which react with the ceramic surface. Methacrylate groups (-CH₂=CHCOO) at the other end, which bond with the resin matrix. 3. Hydrolysis of Silane Molecule Silane molecules require a small amount of moisture to hydrolyze and activate. When applied to the ceramic surface, the methoxy groups (-OCH₃) on the silicon end of silane react with moisture, forming silanol groups (-SiOH): This reaction allows silanol groups to interact with the ceramic surface. 4. Condensation Reaction with Ceramic Surface The silanol groups (-SiOH) on the hydrolyzed silane molecules then undergo a condensation reaction with the hydroxyl groups (- OH) on the silica-rich ceramic surface. This forms a strong, covalent Si-O-Si bond between the ceramic and the silane: This Si-O-Si linkage is chemically stable and creates a durable, strong bond to the ceramic. 5. Polymerization with Resin Cement On the opposite end of the silane molecule, the methacrylate group (-CH₂=CHCOO) remains unreacted during the bonding with the ceramic. This end is free to polymerize with the methacrylate groups in resin-based adhesives and cements when they are applied over the silanated surface. During polymerization of the resin cement, these methacrylate groups co-polymerize, creating a covalent bond between the ceramic (through the Si-O-Si bond) and the resin matrix. Bonding to Traditional Feldspathic Ceramics- Composition and Properties Feldspathic ceramics are a mixture of leucite (potassium aluminosilicate) and glass, exhibiting low to medium flexural strength (up to 120 MPa). They are known for their excellent translucency and esthetics, making them suitable for various dental applications. Bonding to Traditional Feldspathic Ceramics- Applications Commonly used as veneers, inlays, onlays, and full-coverage crowns, especially those produced via CAD/CAM technology. Bonding to Traditional Feldspathic Ceramics- Clinical Performance Feldspathic veneers show a survival rate of 95.5% over 10 years; inlays/onlays report an 88.7% survival rate over 17 years, indicating their reliability in clinical settings. Bonding to Traditional Feldspathic Ceramics- HF Acid Etching Impact HF etching selectively dissolves the glass phase, revealing silicon dioxide and altering the surface topography to enhance bonding. Micromechanical Interlocking: The roughened surface increases contact area for better adhesion. Surface Cleaning: Removes contaminants and unwanted oxides, improving bonding effectiveness. Bonding to Traditional Feldspathic Ceramics- Optimal Etching Protocol A recommended protocol is using 9.8% HF acid for 2 minutes, followed by the application of a silane coupling agent. This balance optimizes surface roughness without excessively weakening the ceramic. Resin Cement Selection and Properties- Light-Cure Resin Cements Provide extended working times and ease of excess cement removal prior to light activation, thus simplifying finishing procedures. Color Stability: Light-cure resins generally maintain better color stability over time as they do not contain chemical activators that may lead to discoloration. Resin Cement Selection and Properties- Dual-Cure Resin Cements Combine the benefits of light-activated and chemical-activated systems, achieving high degrees of polymerization while providing a longer working time. Mechanical Properties: These cements typically have superior strength and hardness but may exhibit reduced color stability due to the oxidation of tertiary amines present in the formulation. Resin Cement Selection and Properties- Bonding Protocols Various bonding techniques (total-etch, self- etch, self-adhesive) yield similar long-term outcomes, yet total-etch systems typically provide a stronger bond and minimize microleakage more effectively than self- etch systems. Microstructure and Mechanical Properties The microstructure of feldspathic ceramics influences their mechanical performance, particularly the balance between the glass and crystalline phases. Higher glass content generally enhances translucency but can compromise strength. Acid Etching Chemistry The chemistry of HF etching involves the reaction of HF with silica to form soluble silicates, which is fundamental in creating a surface conducive to bonding. Topographic Changes: The dissolution of the glass phase results in increased surface roughness and a more uniform crystal structure, which contributes to the effectiveness of subsequent bonding processes. Bonding to Leucite-Reinforced Glass Ceramics- Composition and Properties Leucite-reinforced feldspathic ceramics contain up to 45% leucite, a potassium aluminosilicate crystal that enhances the material's mechanical properties. Flexural Strength: Exhibits medium flexural strength values of up to 140 MPa, which is higher than traditional feldspathic ceramics. Crack Propagation Resistance: Leucite acts as a crack reflector, helping to redirect and mitigate crack propagation, thus enhancing the durability of the ceramic. Bonding to Leucite-Reinforced Glass Ceramics- Clinical Performance Survival Rates: 94.6% survival rate for leucite-reinforced ceramic inlays and onlays after 5 years. 95.2% survival rate for leucite-reinforced ceramic crowns after 11 years. Retention: None of the failed restorations experienced loss of retention, indicating effective bonding and durability. Bonding to Leucite-Reinforced Glass Ceramics- Bonding Process HF Acid Etching: Recommended etching protocol involves 9.8% HF acid for 1 minute. The shorter etching time compared to feldspathic ceramics is due to a lower glass phase content. The etching creates microretentive surfaces that enhance bonding. Bonding to Leucite-Reinforced Glass Ceramics- Resin Cement Selection Various types of resin cements demonstrated similar long-term outcomes, provided that HF acid-etched surfaces were subsequently treated with silane, ensuring strong chemical bonds. Bonding to Lithium Silicate Ceramics- Composition and Properties Lithium silicate ceramics feature a glass phase predominantly composed of lithium disilicate and lithium orthophosphate, leading to enhanced mechanical properties. Flexural Strength: With values up to 470 MPa, lithium silicate ceramics are among the strongest silica-based ceramics available, providing exceptional fracture resistance without compromising translucency. Bonding to Lithium Silicate Ceramics- Clinical Applications Commonly used for inlays, onlays, crowns, three-unit fixed dental prostheses in anterior regions, and implant-supported crowns. Survival Rates: Reported survival rates are 96.7% for pressed lithium silicate posterior full coverage crowns and 95.2% for partial coverage inlays and onlays, with no instances of debonding noted during 16.9 years of clinical follow-up. Bonding to Lithium Silicate Ceramics- Bonding Process HF Acid Etching: Requires only 20 seconds of etching with a lower HF acid concentration of 4.6%. This process effectively removes the glass phase surrounding the crystalline structure, enhancing surface roughness for improved bonding. Silane Coupling Agent: After etching, applying a silane coupling agent is crucial for forming durable chemical bonds with resin cements. Bonding to Lithium Silicate Ceramics- Resin Cement Performance Various resin cements have been shown to produce durable resin bonds over the long term: Advancements in Bonding Protocols Universal Bonding Agents: Recent developments include universal bonding agents designed to bond to both tooth structures and a wide variety of dental materials. These agents typically contain silane to extend their applicability to silica-based ceramics. Effectiveness: Although universal agents simplify bonding protocols, their specific silane concentrations and other components may reduce effectiveness, leading researchers to recommend using a separate layer of silane to optimize bond strength. Advancements in Bonding Protocols- Ceramic Primers New ceramic primers that include silane and special phosphate monomers have been developed, allowing them to bond effectively to both silica-based and metal-oxide ceramics. Bonding to Lithium Silicate Ceramics- Mechanical Properties The presence of leucite and lithium disilicate enhances the ceramics' ability to withstand stress and resist fracture, which is crucial for their application in high-load-bearing situations. Bonding to Lithium Silicate Ceramics- HF Acid Etching Chemistry The etching process involves a chemical reaction where HF selectively dissolves the glass phase of the ceramics, leading to increased surface area and roughness, which facilitate better mechanical interlocking with resin cements. Bonding to Lithium Silicate Ceramics- Silane Chemistry Silane coupling agents function by forming siloxane bonds with the hydroxyl groups on the etched ceramic surface, thereby improving the adhesion between the ceramic and the resin cement. Bonding to Polymer-Infiltrated Ceramic Network Materials (PICN)- Composition and Characteristics PICNs is a dual-network structure comprising a ceramic skeleton and infiltrated resin phase, generally fabricated by infiltrating the polymerizable monomers into the pre-sintered ceramic skeleton and curing by heat-induced polymerization PICN materials combine ceramics and polymers, featuring an interpenetrating network made of 86 wt% aluminum oxide-enriched leucite and 14 wt% urethane dimethacrylate and triethylene glycol dimethacrylate. This unique structure aims to replicate the mechanical behavior of natural teeth, offering advantages such as reduced brittleness, improved flexibility, and enhanced machinability compared to traditional silica-based ceramics. Bonding to Polymer-Infiltrated Ceramic Network Materials (PICN)- Performance Advantages Flexibility and Fracture Toughness: PICN materials exhibit lower rigidity and enhanced toughness, contributing to better performance in dental applications. Machinability: Reduced milling times and improved internal and marginal accuracy allow for simpler finishing procedures. Wear Resistance: Similar to composites, these materials demonstrate low abrasive wear on opposing teeth, preserving dental integrity. Bonding to Polymer-Infiltrated Ceramic Network Materials (PICN)- Bonding Protocols Surface Pretreatment: Suggested methods include air-particle abrasion (APA) with alumina particles, phosphoric acid, and HF acid etching. Optimal Bonding Technique: A systematic review suggests that APA with alumina followed by silane application is the most effective. However, recent studies advocate for 5% HF etching for 1 minute, followed by silanization, as the best bonding protocol. Bonding to Polymer-Infiltrated Ceramic Network Materials (PICN)- Mechanism of Bonding HF etching exposes the polymer network by selectively removing the ceramic matrix, enhancing surface roughness. Methoxy groups in silane bond chemically with both the ceramic and polymer components, promoting strong adhesion with resin cements. Bonding to Metal-Oxide Ceramics- Characteristics of Oxide Ceramics Oxide ceramics, including alumina and zirconia, lack a glass phase, presenting fine-grain crystalline structures that provide strength and toughness but limited translucency. The absence of glass restricts the effectiveness of HF acid etching, necessitating alternative surface treatments for bonding. Bonding to Metal-Oxide Ceramics- Fabrication and Applications Typically produced using CAD/CAM technologies, oxide ceramics are used as alternatives to porcelain-fused-to-metal restorations. Yttria-stabilized zirconia (Y-TZP) is widely utilized for frameworks and monolithic restorations. Zirconia is classified into generations based on yttria content (3Y- TZP, 4Y-TZP, 5Y-TZP), with varying mechanical and optical properties influencing clinical applications. Bonding to Metal-Oxide Ceramics- Mechanical Properties 3Y-TZP exhibits the highest fracture toughness and flexural strength (>1000 MPa) among zirconia generations and is effective in applications like copings and frameworks. Transformation Toughening: The tetragonal-to-monoclinic phase transformation in 3Y-TZP enhances fracture toughness and limits crack propagation. Bonding to Metal-Oxide Ceramics- Bonding Procedures Surface Pretreatment Methods: Effective techniques include APA, tribochemical silica coating (TBS), low-fusion porcelain application, and laser irradiation, among others. MDP Primers: The application of 10-methacryloyloxydecyl dihydrogen phosphate (MDP) or phosphate ester monomer-based primers is essential for creating durable resin bonds to 3Y-TZP. Preferred Protocol: The APC bonding concept consists of: APA with alumina or silica-coated particles (Step A). Priming with an MDP-based primer (Step P). Application of dual or self-cure composite resin cement (Step C). Considerations for Recent Zirconia Generations- High-Translucent Zirconia 4Y-TZP and 5Y-TZP zirconia generations enhance esthetics by increasing yttria content, making them suitable for full-contour restorations. The higher yttria content leads to more cubic-phase particles, enhancing translucency but reducing fracture toughness compared to 3Y-TZP. Considerations for Recent Zirconia Generations- Bonding Protocols While bonding methods for 3Y-TZP also apply to newer zirconia generations, careful attention to surface pretreatment is critical to avoid compromising mechanical properties. Studies indicate that surface treatments do not adversely affect the flexural strength of newer zirconia generations. Cleaning and Contamination Prevention- Importance of Isolation Proper isolation during resin bonding is crucial to prevent contamination, which can compromise bond strength. Tools such as rubber dams and gauze should be employed to maintain a clean environment. Cleaning and Contamination Prevention- Cleaning Methods Recommended Solutions: Alcohol solutions (ethanol and isopropanol) and phosphoric acid are effective for cleaning ceramic surfaces post-try-in. Effective Decontamination: APA with alumina particles outperforms alcohol solutions for cleaning oxide ceramics. Commercial Cleaning Agents: Products like Ivoclean, Katana Cleaner, and ZirClean effectively remove contaminants and proteins from bonding surfaces. Cleaning in ultrasonic cleaning bath in alcohol for 5 minutes to remove debris and remnants from acid Recommended bonding protocols for ceramic restorations Challenges to Dental Adhesion 1. Moisture Control and Contamination 2. Structural Variability in Dentin and Enamel 3. Polymerization Shrinkage and Stress 4. Hydrolysis and Degradation Over Time 5. Thermal and Mechanical Cycling 6. Chemical Incompatibility 7. Technique Sensitivity Thank You