Cellular Biology 3 - Past Paper PDF
Document Details
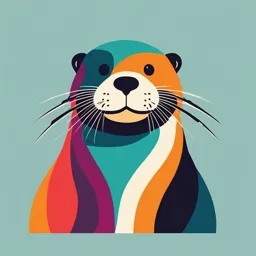
Uploaded by FlourishingExtraterrestrial
Università del Sacro Cuore
Marta Baldi
Tags
Summary
This document provides an overview of the functions and structure of the nucleus, including the nuclear envelope and pore complexes. It details the components, such as chromatin, nucleoli, and Cajal bodies. The document also explains the interaction of the nucleus with other cellular structures and the regulation of nuclear processes.
Full Transcript
Lecture Cellular Biology 3 Professor Lorena Di Pietro Author Marta Baldi Checker Sofia Fontana Date...
Lecture Cellular Biology 3 Professor Lorena Di Pietro Author Marta Baldi Checker Sofia Fontana Date 07/10/2023 Functions of the Nucleus The nucleus is an organelle specific to eukaryotic cells (not in prokaryotes) where hereditary material (DNA) is stored. It is the information processing and administrative center of the cell. It coordinates processes such as cell growth, cell metabolism, gene expression, cell proliferation, protein synthesis and regulation, and reproduction (cell division). Structure of the Nucleus The nucleus is the largest organelle in the cell and typically occupies about 10% of the cellular volume. The nucleus contains a viscous semifluid matrix similar to the cytoplasm called nucleoplasm containing: - Chromatin (condensed to form chromosomes during mitosis) - Nucleoli - Cajal bodies, GEMS (Gemini of coiled bodies), and interchromatin granule clusters - Nucleotides - Structural proteins - Enzymes Sometimes in the lab, we need to use specific antibodies that can label and mark the nucleus so that it’s more visible but usually it's visible with an optic microscope (seen in the images below). Usually, there is one nucleus per cell (with a rounded shape) but there are some exceptions: - Multinucleated cells like osteoclasts, megakaryocytes, and striated muscle cells (these also have elongated nuclei - not round) - Some white blood cells (granulocytes) have modified shape nucleus in order to recognise and categorize these type of granulocytes - Cells without a nucleus (erythrocytes) The Nuclear Envelope The nucleus is surrounded by the nuclear envelope which is a double-layered membrane. It has the same composition as all other membranes in the cell (plasma membrane). It separates the content of the nucleus (nucleoplasm) from the cellular cytoplasm and stabilize the structure of the nucleus. It allows attachment of the nucleus to the cytoskeleton of the cell, and it is selectively permeable by regulating the passage of certain molecules (because it has nuclear pores). Between the inner and outer membrane, there is a 10-50 nm space called the perinuclear space which is continuous with the lumen of the RER. The RER is continuous with the outer membrane of the nuclear envelope. The inner membrane is anchored by integral membrane proteins to a fibrillar network called the nuclear lamina. The inner and outer membranes have different structures because they have different integral proteins embedded within them. The proteins provide structure for the nuclear envelope. The entire content of the nuclear envelope is referred to as the nucleoskeleton. It is similar to the cytoskeleton of the cell. The cytoskeleton is a net of proteins that give shape to the cell which will be studied in greater detail later. The nucleoskeleton includes more than 80 unique integral membrane proteins localized at the the nuclear envelope in mammalian cells. Among these proteins, we have 3 particular families of them, characterized by a distinct motif: there is the SUN protein family (mostly located in the inner nuclear membrane), the KASH protein family (in both the inner and the outer membrane) and the LEM protein family (mostly localized in the inner nuclear membrane and in the nucleoplasm). These proteins regulate specific mechanisms of the nucleus. Nesprins (nuclear envelope spectrin repeat proteins) are expressed primarily on the outer nuclear membrane part of the LINC complex. The LINC complex is a protein network that connects the nuclear envelope to the cytoskeleton (which is outside the nucleus) and the nuclear lamina (which is inside the nucleus). It establishes connections between the cytoskeleton and the nucleoskeleton. Nesprin-1 and -2 bind to the actin microfilaments. Nesprin-3 binds to plectin, which is bound to the intermediate filaments. Nuclear Pore Complexes The nuclear envelope has nuclear pore complexes (NPCs). There usually are 3,000 - 4,000 pores on a nuclear envelope. They are gateways through the nuclear envelope, and transport through them is highly regulated. They are made of proteins called nucleoporins arranged in a specific manner. There is symmetry between the outer side and the inner side of the nuclear pore complex. Some proteins synthesized in the cytoplasm need to be transported back to the nucleus because they work in the nucleus. RNA also needs to be transported through the nuclear pore complexes. The movement of RNA and proteins through the nuclear pores is bidirectional because they can leave and enter the nucleus as needed. Each nuclear pore is composed of a set of approximately 30 different proteins (nucleoporins) repeated in multiple copies. We havea 8- folds rotational symmetry, namely, a repetition of a multiprotein module for 8 folds aroundthe opening of the pore. In the image on the left we can see the structure of the nuclear pore. Cytoplasmic filaments are used in the regulation of transport to recognize and allow the movement of proteins from the cytoplasm to the nucleus. The region in the middle is the central scaffold that creates the pore. The transmembrane ring anchors the nuclear pore complex within the nuclear envelope. The cytoplasmic ring is essential to stabilize the structure. The nuclear ring and nuclear basket create a mesh/net. The resulted mesh restricts the diffusion of large macromolecules while allowing smaller molecules to pass. FG nucleoporins (phenylalanine- glycine-rich nucleoporins) are unstructured protein regions of nucleoporins and are the regulators of nucleocytoplasmic transport. They allow fast, yet selective, transport of molecules through the nuclear pore complex, but the underlying mechanism of nucleocytoplasmic transport is not yet fully discovered. The nuclear pore is not always open, and transport is highly regulated. Transport Regulation Proteins need to be able to pass from the nucleus to the cytoplasm and vice versa. Proteins allowed to travel across the nuclear pore complex are able to interact selectively with nuclear transport receptors. These proteins can be categorized as: Importins: transporting macromolecules into the nucleus The protein that need to be imported into the nucleus has an NLS (nuclear localization signal) in its amino acid sequence, which is recognized by the importin. If the protein does not have the NLS signal, it cannot be recognized by the nuclear pore complexes and cannot enter the nucleus (the NLS amino acid sequence is Pro-Lys-Lys-Lys-Arg-Lys-Val). Below is this process as explained in the class slides (slide 29): 1. NLS binds a nuclear transport-receptor in the cytoplasm (heterodimer importin 𝛼/𝛽) 2. Importin complex interacts with cytoplasmic fibers of the NPC and translocates inside the channel, where FG nucleoporins allow the passage. 3. The GTP-binding protein Ran allows releasing the complex inside the nucleoplasm, by detaching importin 𝛽-subunit 4. Ran-GTP- importin 𝛽 complex travels backward in the cytoplasm 5. In the cytoplasm, Ran-GTP is hydrolyzed into Ran-GDP and is released from the importin 𝛽-subunit binding The following is how the professor explained it in class: Once this complex (protein bound to NLS) is inside the nucleus, another protein intervenes which is called ranGTP. RanGTP is a protein that binds GTP (a molecule similar to ATP with a lot of energy stored within its linkages). RanGTP recognizes this complex, it links to importin and releases the protein within the nucleus. The importin protein linked to ranGTP can exit the nucleus. The link between the importin and ranGTP works as a signal in the NPC to allow the exit of this complex from the nucleus. When this complex arrives in the cytoplasm the ranGTP is hydrolyzed into ranGDP and the importin is released. RanGDP then travels back inside the nucleus and is re-converted in the GTP form. This process is summarized in the image below: Exportins: exporting macromolecules out of the nucleus Exportins feature a NES (nuclear export signal) complex which is 4 hydrophobic amino acids residues in different combinations Below is this process as explained in the class slides (slide 30): 1. NES binds a nuclear exportin (CRM1) and Ran-GTP 2. The heterotrimeric NES protein-CRM1-RanGTP complex travels across the NPC 3. The complex can then diffuse to the cytoplasm where GTP is hydrolyzed to GDP and the NES-protein is released 4. The CRM1-RanGDP complex diffuses back to the nucleus 5. In the nucleus, GDP is changed into GTP. And the following is the process summarized by the professor in class: Proteins that need to exit from the nucleus feature a nuclear export signal (NES). Exportins recognize these proteins. RanGTP is recognized by NPCs which allow the exportation of the protein out of the nucleus and into the cytoplasm. RanGTP is hydrolyzed into GDP and the energy is used. The exportin and ranGDP diffuse back into the nucleus and the ranGDP is re-converted into the GTP form. There are many different types of RNA within the cell which will be studied in detail later (mRNA, rRNA, snoRNA, miRNA, and tRNA), and each of them uses different types of transports. They are molecules assembled into the nucleolus, specifically into ribonucleoproteins to travel across the nucleus (you can see it in the image below). In the nucleus, many RNAs are synthesized, and they need to be transported out because they work inside the cytoplasm. Only mature RNA can exit the nucleus. RanGTP also helps RNA to exit from the nucleus. The Nuclear Lamina The nuclear lamina is a nuclear skeletal structure of laminospecific proteins (lamins and lamin-associated proteins) which is similar to a net. It creates support under the nuclear envelope and stabilizes it and regulates its shape. The nuclear lamina is similar to the cellular cortex (made of cytoskeletal proteins) under the plasma membrane. The nuclear lamina interacts with chromatin and may therefore participate in determining the 3D organization of chromatin in the interphase nucleus. It also plays an active role in regulating processes like cell differentiation and proliferation (mitosis), DNA replication and repair, transcription, gene expression and epigenetic modifications. Lamins are an example of intermediate filaments of the cytoskeleton. They are linear proteins that contain an NLS signal which makes them easily recognizable by importins which then transport the lamins into the nucleus to form the nuclear lamina. Lamins are synthesized in the RER then they are transported into the nucleus to assemble into homodimers. Multiple homodimers assemble in antiparallel directions to form tetramers and then the tetramers bind in series to form filaments. Filaments are very strong structures. Many filaments create the mesh of the nuclear lamina, which is very resistant and stabilizes the nucleus. The assembly of the nuclear lamina is regulated by Ser-phosphorylation by cyclin-dependent kinase-2 (CDK2). We classify 4 different types of lamins (A, B1, B2, C), with similar structure and conserved elements. Sequence motifs required for targeting newly synthesized lamins to the nuclear envelope have been identified: Like the nuclear envelope, the nuclear lamina go through assembly and disassembly (both of the processes are regulated by phosphorylation; in particular lamins are disassembled due to Ser-phosphorylation by cyclin-dependant kinase-2 also called CDK2) during cell division. During cell division, the nucleus disappears, and DNA is released into the cytoplasm. The nuclear lamina is split into many parts which are taken in by many vesicles. Lamins A and C are then released in the cytoplasm as dimers while lamin B stays attached to vesicles resulting from nuclear envelope disaggregation. In the final stages of nuclear division, those vesicles fuse back together and recreate the nuclear lamina and nuclear envelope in the 2 daughter cells. Laminopathies are genetic disorders caused by mutations in genes coding for lamins. The alterations caused by these mutations most often arise in muscle tissue, but there are a lot more problems that can appear (heart, bones, fat, skin, growth, and face abnormalities). The issue caused by these mutations does not only affect the shape of the nuclear lamina, but also the DNA organization within the nucleus. This leads to problems with DNA transcription and replication. An example of a laminopathy is Hutchinson-Gilford Progeria Syndrome (HGPS) which leads to the premature aging of all cells caused by a mutation in the LMNA gene. This is a very rare disorder that affects about 1 in 4 million new-borns worldwide. INNER COMPARTMENTS OF THE NUCLEUS Into the nucleus we can find different molecules, like DNA, proteins, and enzymes. All these molecules play a role in regulating processes that happen in the nucleus, but we must mention also some other structures important for it: NUCLEOLI CAJAL BODIES CHROMATIN GEMS INTERCHROMATIN GRANULE CLUSTERS NUCLEOLUS It could be one or more regions of the nucleus, not surrounded by a membrane. Some cells have more than one nucleolus in their nucleus; this could happen during the proliferative cell cycle, both under normal and pathological conditions, and it can also be observed in neuronal nuclei at early stages of their maturation. The nucleolus is the ribosome producing factory, the site of ribogenesis: into the nucleolus ribosomes are produced and assembled. Moreover the synthesis and processing of rRNAs also take place in this structure. RIBOSOMES They are small intercellular structures made of both RNA and proteins. Ribosomes are the site of translation or protein synthesis in the cell. We have thousands of ribosomes in our cells, and we can find them most in the cytoplasm. They are composed by two subunits: large subunit and small subunit, each of them is made of ribosomal proteins and ribosomal RNA (rRNA). There are four types of rRNA that differ on the length of the sequence: 5S rRNA 5.8S rRNA 18S rRNA 28S rRNA Ribosomal proteins are synthetized in the cytoplasm and there are specific signals that allows them to translocate through the nuclear pore complexes, into the nucleus. In the nucleolus we can distinguish three major components: Fibrillar center (FC) Dense fibrillar component (DFC) Granular component (GC) The fibrillar center is the site where all the genes coding for ribosomal RNA are transcribed (we have over 200 copies of these genes in our genome, and this helps to respond to the high demand of ribosomes into cells). In the FC all ribosomal RNA are synthesized except for the 5S RNA (synthesized outside the nucleus). All the RNA produced are not mature yet so in the FC they are also processed to become working RNA. Into the granular component occur the assembly of ribosomal subunits, made of RNA and ribosomal proteins. Once assembled the subunit can exit from the nucleus passing through the nuclear pore complex, to let this happen the subunit of ribosome needs to be recognized by specific proteins that will help it to pass. There is a gate provided by FC domains of nucleoporins that need some specific signals to open. We already know that the nucleolus is the site of ribogenesis, but it is also the site where all the types of RNA are produced, not only the ribosomal RNA. It also responds to cell stress by either shrinking or enlarging or disrupting or producing different proteins, and it can regulate more processes, like the cell cycle. Nucleolus is not the only region present into the nucleus, other structures at specific stages of cell cycle can be recognized into it. They are regions where happen RNA processing of different RNA molecules and they are very dynamic structures: they aren’t always present in a cell; their presence depends on the demand, and they could be present or absent depending on different stimuli that arrive to the cell. CAJAL BODIES (COILED BODIES) In the photo below, you can see the cajal bodies (green) which are small spherical sub- organelles (0.3-1.0 m in diameter) in the nucleus of proliferative cells (embryonic cells and tumor cells) or metabolically active cells like neurons (cajal bodies are typically associated to nucleoli in neurons). They are regions of the nucleoplasm not surrounded by a membrane and contain proteins that helps the processing of maturation of RNA and regulation of telomere’s length. Cajal bodies are implicated in RNA-related metabolic processes: snRNPs and snoRNPs biogenesis maturation and recycling histone mRNA processing telomere maintenance (assemble RNA which is used by telomerase to add nucleotides to the ends of telomeres) INTERCHROMATIN GRANULE CLUSTERS Clusters of 1-to-many m containing 20-25 nm granules connected in abeaded chain fashion by thin fibrils Located in the interchromatin regions of the mammalian cell nuclei Enriched in pre-mRNA splicing (essential for RNA proteins maturation) factors Usually appear as irregularly shaped structures that vary in size and number They act fast and rapidly in specific moment, in specific ways, because the cell has specific needs DNA The structure: DNA and RNA are nucleic acids, the unit that composes their molecule is the nucleotide. The nucleotide is formed by three parts: Pentose sugar Phosphate group Nitrogenous base (Adenine, Thymine, Guanine, Cytosine, Uracil) The different nucleotides differ each other for the presence of different bases. They can create a filament binding one each other through a condensation reaction between the sugar of a nucleotide and the phosphate group of the next nucleotide; this reaction is called phosphodiester linkage. Each filament has a specific direction, because it grows starting from 5’ end, that expose the phosphate group, to the 3’ ends that expose the sugar. By the covalent bonds between the phosphate groups and the Pentose sugars we form a DNA strand. The DNA molecules is composed by two DNA strands, so to form a DNA molecule the nitrogenous bases of two strands need to bind each other. The two strands have to be antiparallel because we need linkages between bases that are very specific: Adenine can bind just to Thymine and Guanine can bind just to Cytosine. Because of that if we know the sequence of a DNA strand we can discover the sequence of his antiparallel strand, indeed if we have an A on the first one, then we will have a T on the other. Adenine and Thymine interact each other with two hydrogen bonds while Guanine and Cytosine interact with three hydrogen bonds. DNA molecule is arranged as a double helix. Also, RNA is a nucleic acid but there are three main differences between DNA and RNA. DNA RNA Nitrogenous bases. Cytosine, Guanosine Cytosine, Guanosine Adenine, Thymine Adenine, Uracil Structure Double strand (double helix) Single strand (specific structure for each different type of RNA) Pentose sugar Deoxyribose Ribose Each of our cell contains in the nucleus (around five micrometers diameter) a two meters DNA molecule (about 3 billion DNA base pairs). PROKARYOTES AND EUKARYOTES DNA DNA in prokaryotes is organized in a single circular molecule, located in the cytoplasm in a specific region called nucleoid (in prokaryotes there aren’t compartments or a nucleus). In some specific cases bacteria also contain smaller additional circular DNA molecules, called plasmids, that is not essential. Plasmids do not contain genes essential for the bacterium’s survival, but they help the bacterium to overcome occasional stressful situations (antibiotic resistance). On the contrary, in eukaryotic cells the DNA is organized into different linear structures, the chromosomes, and the number of these molecules’ changes for each species. Each eukaryotic chromosome contains a single linear DNA molecule DNA PACKAGING In eukaryotic cells the chromosomes are composed of DNA and associated proteins and, in order to let the DNA enter into the nucleus, it is packaged in a specific way with the help of these proteins. The result of this packaging is an organized structure called chromatin. Also in bacteria we can find numerous small proteins molecules associated with the chromosomal DNA, causing it to fold into a more compact structure. It is important to maintain the DNA in a state that is accessible to enzyme and regulatory proteins. However, the packaging of the DNA is not always the same because sometimes it changes during different stages of the cell cycle. It could happen that sometimes the DNA is packaged and can enter into the nucleus but it’s not possible to read it anymore. The only way to read DNA during the packaging is during the cell division. Chromatin and chromosomes are the alternative states of DNA organized inside the nucleus: chromosomes are clearly visible during mitosis while during the other stages the DNA is less condensed (chromatin). It is important to understand that both packaging of the DNA and the expression regulation are crucial in order to insert the entire genome inside the nucleus and then to organise it to follow the next important processes inside the nucleus. So, DNA is expressed in 2 alternative structural organizations each depending on the functional status of the cell. These 2 organizations of the DNA are chromatin and chromosomes. Chromatin Chromosome DNA is packaged inside the nucleus, but it The DNA is fully condensed and organized occupies the entire nucleoplasm in chromosomes Here all 3 pictures represent the onion root magnified at different factors. The second and third one shows more clearly the stages of the cell cycle, depicting the DNA organization in each phase. In this picture we have the DNA content of a typical human cell (diploid state). Homologous chromosomes meaning chromosome pairs inherited from each parent. All the chromosomes are arranged regarding their length and dimensions. We have 23 pairs of chromosomes, 44 autosomes and 2 sex chromosomes X and Y based on the gender. In females there are two X chromosomes and in males one X and one Y. In the picture on the left we can observe that the number of chromosomes varies between species and is not related to the size and biological complexity of an organism. For example, a dog has 78 chromosomes, so the number of chromosomes represents the number of linear molecules in which DNA is organised. Meanwhile, in humans we have 46 different DNA molecules. As mentioned before, the DNA is associated with proteins to be packed and transported inside the nucleus. There are two types of proteins associated with the DNA: histones and non-histone proteins. Histones are present in high quantity and we recognize multiple copies of the genes that encode for these proteins. Non-histone proteins, on the other hand, are less abundant and more than 1000 types of these proteins are identified. They are involved in a range of activities such as: DNA packaging, DNA replication and gene expression. Histones are basic proteins (enriched in lysine and arginine residues), extremely conserved across species that intervene in the first steps of DNA packaging. There are five types of histones: - H1 - H2A - H2B - H3 - H4 DNA and histones are complexed into repeated units named nucleosomes (11 nm diameter). The last 4 types of histones intervene in the first step of DNA condensation: the formation of the nucleosome (DNA+ histones) that will be now described: - First step: The DNA double helix is wrapped around protein complexes (histones) to form nucleosome. This arrangement is called beads on a string (as you can see in the image below). A nucleosome consists of 8 histone molecules: 2 H2A, 2 H2B, 2 H3 AND 2 H4. As you know, the DNA is negatively charged due to the presence of the phosphate group, so the positively charged of the basic histones interact with the DNA. There are about 147 base pairs of supercoiled DNA wrapping around a disk-shaped complex of 8 histones (histone octamer) The following pictures shows the internal organization of the nucleosome. It is important to remember that the C-term mediates the dimerization of the histone molecules and also allows the interaction of different histones with the nucleosome. While the N-term extends out of the structure and are targeted by enzymes to make the chromatic accessible to proteins for duplication, transcription, regulation, recombination and repair. - Second step: This step is about the organization of DNA in more packed structures called 30-nm chromatin fiber. This step requires the intervention of H1 system which represents the linker histone that binds to part of the linker DNA that connects one nucleosome core paticle with the next one. As you can see in the image below, the linker DNA links the two nucleosomes (figure a), and many such linkages creates a more complex structure (figure b) in which each histone is intertwined. Alternating nucleosomes interact across the helix via the linker DNA. The final structure is referred to as 30 nm diameter of DNA. histone H1: the linker between two nucleosomes - Third step: These further steps of the DNA packaging include the presence of different proteins. In this image, the green rings made of cohesin tighten the loops of the DNA so that the 30 nm-thick chromatin fibre is further organized into wide loops attached to a protein ‘scaffold’ (80-100 nm diameter). There are 100 proteins that represent the protein scaffold in which the DNA structure is linked to form the loops, brought together by coeshin. - Fourth step: Now we move to the final step which occurs during cell division. It is important that the cell organises the DNA and distributes all the molecule from the mother to the daughter cells. Here the intervention of condensins (proteins) is required to assemble the chromosomes and fully condense the DNA. For example, non-histone proteins will allow the formation of chromosomes. It is calculated that 1 m chromosome contain about 1 cm of linear DNA. It is crucial to follow this specific packaging in order to allow the entire DNA to fit inside nucleus and to guarantee the distribution of the DNA. The compact structure of the chromosome makes the DNA inaccessible to replication and transcription factors. The nuclear lamina intervenes in the process of chromosome organization. The nuclear lamina acts as a sort of scaffold/matrix for the loops of DNA in order to help the organization of DNA in the nucleus. The chromatin is highly organized in interphase. The maximum efficiency of the various nuclear processes is ensured. The chromosomes occupy specific regions inside the nucleus that vary among different cell types. In this image you can see in different colors the different chromosomes which occupy a specific region inside the nucleus. An example of organization of chromosomes in the interphasic nucleus is nucleolus In the outer gray region we have DNA pieces that codify for ribosomal RNA. In the inner black region we have all the DNA with specific genes. On the right we have the structure of the chromosome, composed of two arms called chromatids, each of them having a double DNA helix. The chromatids are linked together in a site called centromere. Each chromatid is composed of two arms: P arm (the short arm) and Q arm (the longer one). During interphase about 10% of chromatin remains in a partially condensed status and is called heterochromatin. The peripheral regions of the nucleus are thought to contain factors that promote transcriptional repression (favorable environment for heterochromatin). The rest of the chromatin, during interphase, return to a dispersed (less condensed) active state called euchromatin. This is also more accessible to proteins. In the cell we have two types of heterochromatins: - Constitutive heterochromatin: This chromatin is permanently inactive and the DNA does not have genes so we have repeated units of DNA that does not interfere in the gene expression. Even though it does not have genes it is still important. The majority of constitutive heterochromatin is found at the pericentromeric regions of chromosomes but is also found at the telomeres and throughout the chromosomes. An example of constitutive heterochromatin is the long arm of chromosome Y. They also inhibit genetic recombination between homologous repetitive sequences. - Facultative heterochromatin: DNA regions that are selectively inactivated in some stages or in specific cell types of an organism. For example the X chromosome inactivation in female leads to the formation of the ‘Barr Body’ in cells. Incorrect inclusion of genes in the process of heterochromatization can cause some diseases: - The gene coding for b-globin is located near a region of heterochromatin - If due to a hereditary deletion, the region of heterochromatin is extended, the b-globin gene is poorly expressed, and the person suffers from severe anemia.