Cell Investigation Methods PDF
Document Details
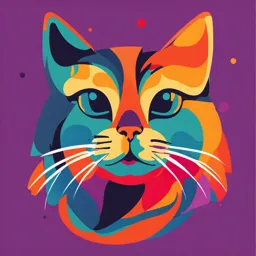
Uploaded by GoldResilience
Gökçe ERDEMİR CİLASUN
Tags
Summary
This document discusses various methods used for investigating cells. It covers different types of microscopy, including phase-contrast, interference, and dark-field microscopy. The document also explores techniques for analyzing cell components, such as centrifugation, and explains concepts like stem cells and cloning.
Full Transcript
Cell Investigation Methods Asst. Prof. Gökçe ERDEMİR CİLASUN Learning Objectives Student can list the methods of examining the cell, can know the properties of microscopes, can explain the methods of analyzing the cell by separating it into its components, can explain the different...
Cell Investigation Methods Asst. Prof. Gökçe ERDEMİR CİLASUN Learning Objectives Student can list the methods of examining the cell, can know the properties of microscopes, can explain the methods of analyzing the cell by separating it into its components, can explain the different types of centrifugation method, can explain the concepts of stem cell and cloning. METHODOLOGY Methods that Techniques used study the cell as a for the analysis of whole cell components Examination with Vital Examination fixation Cell Cultures Vital Examination It is the microscopic examination of a living cell in a liquid medium without damaging the cell. During this examination, some dyes such as neutral red, which is harmless or only slightly harmful to the cell, are used. Special microscopes used in vital examinations are as follows. 1. Phase - contrast microscope 2. Interference microscopy 3. Dark field microscopy 1. Phase - contrast microscope When the cell is alive, it is highly permeable to visible light. The amount of light passing through the various parts of the cell is so close that the human eye or photographic film cannot distinguish the difference. By adding some optical parts to a normal light microscope, the difference between the light intensity passing through various parts of the cell is increased. The phase-contrast system does not increase the separation power of the microscope, it only increases the contrast between the various parts of the cell. It creates a contrast by converting the differences in the phase of light passing through different parts of the cell with different light refraction coefficients into differences in light intensity. 1. Phase - contrast microscope The light passing through the nucleus is slightly delayed, creating a difference between the phases of light passing through the nucleus and the adjacent cytoplasm, and the cell is examined in this way. The advantage of this method is that it makes it possible to examine the internal structure of living cells without the use of any staining process. 2. Interference microscopy The principle of the interference microscope is the same as that of the phase-contrast microscope. In this microscope, calcite or quartz plates are added in front of the condenser. Through these double-refracting plates, light is emitted in two beams. One of these rays passes through the object under investigation and the other through the medium surrounding the object. 2. Interference microscopy When these two beams are incident on each other, there is a phase difference between the beams because the beam passing through the object is slightly delayed. In this way, cells can be examined in unstained preparations by creating an image in the form of a relief. The delay varies depending on the thickness of the object and the refractive index of the medium. 3. Dark field microscopy It was created by attaching a special condenser to the light microscope. This condenser ensures that all rays come from the side of the object and are reflected and reach the eye. In a dark field microscope, since the illuminating rays are directed from the side, the boundary between the different phases in which the light is scattered in a dark field is seen brightly. Examination with fixation Since the light refraction coefficients of different parts of living cells are close to each other, it is difficult to examine them under a microscope. In order to better observe the details of the cell, the cell must be fixed and then stained with different dyes. Only after this staining is it possible to examine the cells with a microscope. Today, normal light microscopes, fluorescent microscopes, X-ray microscopes and electron microscopes are used to examine fixed and stained cells. Light Microscope These microscopes consist of two main parts: mechanical and optical. The mechanical part consists of the microscope head, microscope body/arm, coarse (macro) and fine (micro) adjustment mechanism, object table and carriage. The optical section is the part mounted on the mechanical section that allows the image to be taken and examined. This section consists of: ocular, tube (mono, bino or trinocular), objective, condenser and light source. Light Microscope The rays from the light source are focused by the condenser to form a cone of light on the object placed on the object table. The rays emitted from the object in the form of a light cone are collected by the objective and transmitted to the eye through the eyepiece. The most important feature of a microscope is its resolution. Resolution is the ability to clearly distinguish two point-shaped objects standing side by side. Moving the objective closer to the object causes the angle θ to increase, thus increasing the resolving power and making the image appear larger and clearer. Light Microscope Today's light microscopes generally use visible wavelengths between 0.4 μm (400 nm) and 0.7 μm (700 nm). Objects closer than 0.2 μm to each other or objects smaller than 0.2 μm in length cannot be seen well with normal light microscopes, no matter how large the image is magnified. For this reason, fluorescence microscopes using fluorescent rays with wavelengths shorter than 0.4 μm (400 nm) or even electron microscopes using electrons with a wavelength of 0.004 nm have been developed. 1mm = 1000µm 1mm= 3 10 μm= 6 10 nm= 107 A° 1µm = 1000nm 1nm = 10A° 1 A°(angström) = 1.0 × 10-10 meters The magnification of the microscope is calculated as follows: The magnification of the microscope = Ocular x Objective Example: Ocular = 15x Objective = 20x ✓The magnification of the microscope = 15 x 20 = 300 Fluorescence Microscope Fluorescence Microscope Fluorescent substances absorb light of a certain wavelength and then re-emit it at a longer wavelength. If such a compound is illuminated with the low-wavelength light it absorbs, then examined through a filter that allows only the light emitted from that compound to pass through, it is seen as bright regions in a dark background. Utilizing this property, fluorescence microscopes have been developed to examine objects stained with various fluorescent dyes. Fluorescence Microscope With a fluorescence microscope, objects as small as 0.1 μm can be examined. This microscope has the same structure as a normal light microscope but differs from it in terms of light source and some optical parts. In these microscopes, mercury vapor bulbs that emit ultraviolet wavelength light are used as the light source. The optical parts used in the microscope such as condenser, objective and ocular are made of quartz which does not absorb ultraviolet light. Fluorescence Microscope Fluorescence microscopes are equipped with a series of special filters to select the wavelength of light used. The first series of filters traps the light coming from the source and passes only the rays that can be absorbed by the fluorescent dye with which the object is stained. The second series of filters prevents the reflected rays from being emitted into the environment and passes only the higher wavelength rays coming from the object. Polarizing Microscope Polarizing microscopes are constructed by adding two light polarizing plates or prisms to normal light microscopes. The first of these plates, the polarizer, polarizes the beam from the light source and sends it to the object. The other plate, the analyzer, is placed directly above the objective or eyepieces. When the analyzer is rotated 360° around itself, the microscope field is seen as dark and light every 180°. Polarizing Microscope If the axes of the polarizer and the analyzer are perpendicular to each other (90°), the microscope field is dark. An anisotropic material delays the incident light and, depending on its refractive coefficient, translates these rays. Due to this inversion, the substance under investigation is seen as a bright region within a dark area. Polarization microscopy does not increase the discrimination power of the microscope, it only increases the contrast, allowing certain objects to be seen better. X-Ray Microscope X-ray microscopy is used to study the structure of atoms and molecules smaller than 1 nm. The mechanism of this microscope is based on the principle of diffraction, in which X-rays that encounter small molecules change their path. The shape obtained in this system is not an image of the object under study, but various concentric dots or lines that show the structural features of that object. X-Ray Microscope In an X-ray microscope, electronic lenses are used to direct X-rays from a source onto the object. The X-rays that leave the object are then incident on a fine-grained photographic plate or a fluorescent screen. X-ray diffraction micrographs are thus obtained. By evaluating the concentric dots and lines in these micrographs, the structure of that molecule is revealed. Electron Microscope Electron Microscope The wavelength of the electrons can be reduced to 0.004 nm in electron microscopes, which are based on the principle that the separation power can be greatly reduced by using electrons and the wavelengths can be shortened by increasing the speed of the electrons. The separation power of the microscope is, theoretically, 0.002 nm. In practice, however, the separation power of such electron microscopes is 0.1 nm, since it is more difficult to correct aberrations in electron lenses than in glass lenses. Structurally, a Transmission Electron Microscope (TEM) is similar to a light microscope but larger. Electron Microscope In these microscopes, the electron source is a filament in a cylindrical column. Since electrons are deflected when they collide with air molecules, this cylinder is evacuated to create a vacuum. The accelerated electrons pass down the vacuum cylinder as an electron beam. Magnetic coils placed at regular intervals in the cylinder, like glass lenses, focus the electron beam. The sample to be examined is placed in the vacuum so that it coincides with the electron beam. Electron Microscope Some of the electrons passing through the sample are scattered depending on the density of the material, while the remaining electrons are focused either on a screen or on a photographic plate to form an image. The sections examined with TEM are two-dimensional images of tissues and cells. However, Scanning Electron Microscopy (SEM) is used to obtain a three- dimensional image. In SEM, electrons are used for the image, but these electrons do not pass through the sample, but are scattered on its surface. Therefore, in this method, the sample to be examined is very thinly coated with a heavy metal such as platinum after fixation. Thus, the electrons reflected from this metal during the examination create a three-dimensional image of the external structure of the object under investigation. Transmission Electron Scanning Electron Light Microscope Microscope (TEM) Microscopy (SEM) Cell Cultures Cell culture technique is a method used to solve many problems related to cell biology. Living organisms usually obtain various nutrients and growth factors from the external environment. In vitro, various external factors are needed for cells to grow and develop. For this reason, culture systems have emerged to mimic in vivo conditions and are still widely used. The basis of this technique is based on the storage of cells from different tissues, especially embryonic tissues, in specific media and their ability to grow in these media. Cell Cultures Various media used in this technique have been developed. These media usually contain complex carbohydrate mixtures, amino acids, salts, vitamins, hormones and various growth factors. The salt concentration of the medium is prepared isotonically to avoid osmotic imbalance. Bicarbonate is usually added to the medium with CO2 as a buffer and is used to maintain an optimum pH of 7.4. The pH indicator added to the medium determines pH fluctuations and bacterial contamination by its color change. Vitamins and hormones often act as cofactors in cell growth. Techniques used for the analysis of cell components A wide variety of chemical techniques are used to study cell components. In this way, proteins, lipids, nucleic acids, etc. can be identified. In addition to these chemical techniques, various other techniques have been developed for more comprehensive studies. Among these; we can give examples of the technique of analyzing the cell by breaking it down into its parts, Recombinant DNA Technology, which has been developing in recent years. Separating the Cell into Sections In this technique, the boundaries of the cells are first homogenized by various mechanical and chemical means. The various organelles or macromolecules in the homogenate are separated according to their mass (Rate zonal centrifugation) or density (Equilibrium density - gradient centrifugation). These fractions are then analyzed using various techniques. Homogenization Techniques A wide variety of techniques are currently used to disrupt cells. These include ultracentrifugation and techniques using homogenizers of very different structures. Homogenization is carried out in a buffer solution or sucrose solution. The reason for using sucrose is that sucrose does not cause damage to the organelles because it cannot pass through the organelle membranes. Homogenization Techniques Again, the pH and content of the buffer solutions used are very important in homogenization. Buffer series specific to the macromolecule to be obtained should be selected. Homogenized tissues or cells are then separated by centrifugation methods. Centrifuge Methods Differential – Velocity Centrifugation Rate-Zonal Centrifugation Equilibrium Density Gradient Centrifugation Centrifuge Methods By centrifugation methods, various organelles or particles of different sizes and densities can be obtained separately on the basis of their different settling velocities. For this reason, homogenized cells are first subjected to Differential velocity centrifugation and then either Rate zonal centrifugation according to their mass or Equilibrium density gradient centrifugation, which separates particles according to their density. Differential – Velocity Centrifugation The homogenate to be separated into portions (fractions) is subjected to increasing centrifugal force. At each stage, the centrifugal force and time are adjusted for the settling of a specific fraction. After each step, the bottom sediment (pellet) is removed and separated for special analysis, while the upper liquid portion (supernatant) is centrifuged again at a higher speed. Thus, all fractions in the homogenate are obtained separately. While organelles or particles of very different mass and density can be separated by this method, organelles or particles of similar mass and density cannot be separated. Therefore, the fractions obtained by this method are again subjected to centrifugation techniques that separate particles according to their mass or density. Rate-Zonal Centrifugation In this method, the previously obtained fractions are added to a centrifuge tube containing a special solution. The solution in the tube is usually sucrose solution. When sucrose is placed in the tube and centrifuged, the bottom of the tube is more concentrated than the top of the tube. When the homogenate added to such a tube is centrifuged, the particles move up and down the tube at a rate controlled by the centrifugal force, the mass of the particle, the density difference between the particle and the medium (sucrose), and the friction between the particle and the medium. When the centrifugation is complete, molecules of different sizes are located in different regions of the tube. This is why this technique is called Rate-Zonal Centrifugation. The sucrose solution, which is located in the tube in different concentrations from top to bottom, prevents the fractions separated by mass from mixing with each other again during the acceleration and deceleration of the centrifuge. Equilibrium Density Gradient Centrifugation It is similar to rate-zonal centrifugation. The most commonly used solution in this method is cesium chloride (CsCl). Because cesium (Cs+) ions are very dense, they form a force field in ultracentrifugation and precipitate. A gradient is therefore created with more Cs+ at the bottom of the tube. Meanwhile, Cl- ions, which follow Cs+ to neutralize the charge, are also more present at the bottom of the tube. In such a centrifugation, the bottom of the tube is about 0.02 g/ml denser than the top. Therefore, molecules of different densities (even if their density is less than 0.02 g/ml) can be easily separated from each other in this technique. Equilibrium Density Gradient Centrifugation Example: When protein, DNA and RNA to be separated by this method are added to cesium chloride solution, protein with a density of 1.3, DNA with 1.6-1.7 and RNA with 1.75-1.85 g/ml can be easily separated. These densities given here are different from the densities of these molecules inside the cell. This is because the CsCl ions in the solution bind to proteins and nucleic acids at different rates, slightly increasing their actual density. Cs+ ions in CsCl solution bind very little to proteins, but bind to DNA through phosphate groups and to RNA through the phosphate group and the hydroxyl group of ribose. RNA is therefore the most dense of all. Recombinant DNA Technology Recombinant DNA technology (rDNA technology) can be briefly defined as the production of genes from an organism (cloning) and the use of these genes for various purposes. The methods used in rDNA technology can be categorized under 3 headings. These are: Classical applications, Hybridization methods, Polymerase Chain Reaction (PCR) methods. Classical applications Classical applications, which are the basis of all methods applied in rDNA technology, take place in 4 stages. 1. Cutting the desired DNA fragments using enzymes called restriction endonucleases. 2. Transferring these fragments to a suitable carrier (vector) cut with the same enzyme. 3. Replication of the fragment + vector complex by transfer to a host organism (cloning). 4. Selection of clones containing specific DNA fragments. Hybridization Methods Hybridization methods are used to determine whether a gene or DNA segment responsible for specific functions exists in a cell or microorganism. Hybridization is the pairing of special DNA fragments, called probes, which have been previously amplified and prepared, with the target DNA molecule whose properties are to be investigated. Hybridization methods commonly used by various laboratories: 1. Southern blot (DNA) 2. Northern blot (RNA) 3. Dot blot methods Polymerase Chain Reaction (PCR) PCR is a method that enables in vitro amplification of intact or fragmented DNA or RNA fragments of an organism or a mutant gene. This method allows results to be obtained even from 1-2 cells obtained from different tissues such as hair strands and sperm. PCR is the process of amplifying a gene or DNA region through a series of replication cycles using oligonucleotide primers that bind to this region. Each replication cycle mentioned in the PCR method 1. Denaturation of DNA 2. Primer binding 3. Extension of the primer It takes place in 3 stages. These cycles are repeated to obtain the desired number of target DNA regions. One cycle lasts about 3-5 minutes and this event is repeated about 20-40 times. The product formed in each cycle is 2 times the previous one. Thus, after a reaction time of about 20 cycles, about 1 million DNA copies can be obtained. In 1827 Karl Von Baer explained that mammals develop from an egg that comes from the mother's ovary. The fertilization of the egg with sperm creates the zygote. Development begins with the division of this fertilized Stem Cell egg (zygote) into two, then four, then eight cells. Continued division and continuous proliferation and differentiation creates hundreds of cell types that differ from the initial single fertilized egg cell in terms of content, shape, size, color and ability to move. These cells come together to form different tissues such as muscle, bone and blood. Stem Cell Stem cell is an undifferentiated cell that can divide to produce some offspring cells that continue as stem cells and some cells that are destined to differentiate (become specialized). Stem cells are an ongoing source of the differentiated cells that make up the tissues and organs of organisms. Stem cells are derived from either animal or human tissues and come from one of three sources: Embriyonic Stem Cells (ESCs) Adult Stem cells (ASCs) Induced Pluripotent Stem Cells (IPSCs) Characteristics of Stem Cells They have unlimited self-renewal capabilities. They are non-differentiated cells with unspecialized functions. They can differentiate into specific cell types under the right conditions. Embriyonic Stem Cells (ESCs) Embryonic stem cells (ESCs) are the cells of the inner cell mass of a blastocyst, formed prior to implantation in the uterus. ESCs are pluripotent and give rise during development to all derivatives of the three germ layers: ectoderm, endoderm and mesoderm. In other words, they can develop into each of the more than 200 cell types of the adult body when given sufficient and necessary stimulation for a specific cell type. Adult Stem Cells (ASCs) Adult stem cells, also called somatic stem cells, are stem cells which maintain and repair the tissue in which they are found. They can be found in children, as well as adults. There are three known accessible sources of autologous adult stem cells in humans: Bone marrow, which requires extraction by harvesting, usually from pelvic bones via surgery Adipose tissue (fat cells), which requires extraction by liposuction Blood, which requires extraction through apheresis, wherein blood is drawn from the donor (similar to a blood donation), and passed through a machine that extracts the stem cells and returns other portions of the blood to the donor. Induced Pluripotent Stem Cells (IPSCs) Adult stem cells have limitations with their potency; unlike embryonic stem cells (ESCs), they are not able to differentiate into cells from all three germ layers. As such, they are deemed multipotent. However, reprogramming allows for the creation of pluripotent cells, induced pluripotent stem cells (iPSCs), from adult cells. These are not adult stem cells, but somatic cells (e.g. epithelial cells) reprogrammed to give rise to cells with pluripotent capabilities. Using genetic reprogramming with protein transcription factors (Oct3/4, Sox2, c-Myc, Klf4) pluripotent stem cells with ESC-like capabilities have been derived. IPSCs Technology Oct3/4 Sox2 c-Myc Klf4 Types of Stem Cells by Differentiation Potential Totipotent stem cells Pluripotent stem cells Multipotent stem cells Oligopotent stem cells Unipotent stem cells Totipotent stem cells They can differentiate into embryonic, as well as extra-embryonic tissues such as chorion, amnion, yolk sac and allontois. The most important characteristic of a totipotent stem cell is that it can generate a fully-functional living organism. Example of a totipotent stem cell is a fertilized egg. Pluripotent Stem Cells Pluripotent stem cells are the descendants of totipotent cells and can differentiate into nearly all cells, i.e. cells derived from any of the three germ layers (endoderm, ectoderm and mesoderm). These three germ layers further differentiate to form all tissues and organs. Among the natural pluripotent stem cells, embryonic stem cells are the best example. Types of Stem Cells Multipotent stem cells can differentiate into a number of cell types, but only those of a closely related family of cells. An excellent example of this cell type is mesenchymal stem cells (MSCs). Oligopotent stem cells can differentiate into only a few cell types, such as lymphoid or myeloid stem cells. Unipotent cells can produce only one cell type, their own, but have the property of self-renewal, which distinguishes them from non- stem cells.