Cell Cycle 2.0.pptx.pdf
Document Details
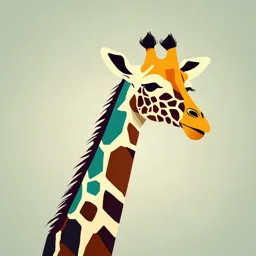
Uploaded by StimulativeWetland
Tags
Full Transcript
Cell Cycle Figure 6.3 Light Microscopy (LM) Electron Microscopy Brightfield Confocal (EM) Longitudinal section Cross section (unstained of cilium...
Cell Cycle Figure 6.3 Light Microscopy (LM) Electron Microscopy Brightfield Confocal (EM) Longitudinal section Cross section (unstained of cilium of cilium specimen) Cilia 50 μm Brightfiel (stained d specimen) 50 μm 2 μm 2 μm Transmission electron Scanning electron microscopy (TEM) Deconvolution microscopy (SEM) Phase-contrast 10 μm Differential-interference- contrast (Nomarski) Super-resolution Fluorescence 1 μm 10 μm INTERPHASE & MITOSIS RUDOLF VIRCHOW INTERPHASE It takes approximately 80% of the cell’s lifetime. Complete organelles Considered to be “resting” from cell division. INTERPHASE The duration of a cell’s life cycle varies from one organism to another. Examples Fly embryo 8 minutes mammals 24 hours INTERPHASE In higher order species, the length of cell cycle is usually controlled by how long it takes to replace damaged cells. G1 phase This stage involves preparation for synthesis and replication of the cellular machinery. It is also in this stage where the cell monitors both the internal and external environments t ensure that all preparations for DNA synthesis have been completed and overall conditions for cell division. G1 Phase G1 takes up about 41 % of the cell’s life cycle. The cell carries out all its normal activities and accommodates all growth processes. G1 completed in about 10 hours G1 checkpoints The cell checked if it is large and healthy enough to continue through the next phases towards cell division. If fails, the cell will quit the cycle and remain in G0 The proteins that activates that checkpoints in interphase is kinase. S Phase The cellular content of the DNA is duplicated in this stage of the cell cycle. The length of S phase varies according to the total DNA that the particular cell contains: the rate of synthesis of DNA is fairly constant between cells and species. Cell will take between 5 and 6 hours to complete S phase. Taking up approximately 20 % of the cell cycle. S phase in six hours G2 Phase During this stage, the cell synthesizes the proteins required to assemble the machinery required for the separation of duplicated chromosomes (mitotic process). G1 stage, the cells in G2 also monitor the internal and external environments to ensure that faithful replication of the DNA has occurred and that the conditions for cytokines is favorable. There is a major check point at the end of G2 phase that controls the entry to M-phase G2 is shorter, lasting only 4 hours in most cells. The stages of the cell cycle from G1, S and G2, are preliminary stages before the actual cell division. These preliminary stages are referred to as the interphase or resting stage while the actual cell division is the M phase. MITOSIS This takes up 20% of the cell’ life span All regular activities of the cells come to a stop when it starts dividing. M Phase This phase is characterized by an ordered series of events that leads to the alignment and separation for the duplicated chromosomes during the S phase of the cell cycle. Mitosis Mitosis is a type of cell division in which one cell ( the mother) divides to produce two new cells (the daughters ) that are genetically identical to itself. Mitosis is the part of the division process in which the DNA of the cell’s nucleus is split into two equal sets of chromosomes. Stages of Mitosis (Please Pee on the MAT) Prophase Prometaphase Metaphase Anaphase Telophase Prophase in this stage, chromatin in the nucleus begins to condense and become visible in the light microscope and now called chromosomes. The nucleolus disappears and the centrioles begin moving to the opposite ends of the cell. The fibers extend from the centromeres. Some fibers cross the cell to form mitotic spindle. Early Prophase In early prophase, the cell starts to break down some structures and build others up, setting the stage for division of the chromosomes. The chromosomes start to condense ( making them easier to pull apart later on) The Mitotic Spindle begins to form. The spindle is a structure made of microtubules, strong fibers that are part of the cell’s “skeleton”. Its job is to organize the chromosomes and move them around during mitosis. The spindle grows between the centrosomes as they move apart. The Nucleus, a part of the nucleus where ribosomes are made, disappear. This is a sign that the nucleus is getting ready to break down. Late Prophase (Prometaphase) In late prophase ( sometimes called prometaphase), the mitotic spindle begins to capture and organize the chromosomes. The chromosomes finish condensing, so they are very compact. The nuclear envelop breaks down, releasing the chromosomes. The mitotic spindle grows more, and the some of the microtubules starts to “capture” chromosomes. Spindle Anatomy Microtubules can bind to chromosomes at the kinetochore, ( a patch of protein found on the centromere of each sister chromatid). Centromeres are the regions of DNA where the sister chromatids are mostly connected. Microtubules that bind a chromosomes are called kinetochore microtubules. Microtubules that don’t bind to kinetochores can grab on the microtubules form the opposite pole stabilizing the spindle. More microtubules extend from each centrosome towards the edge of the cell, forming a structure called Aster Metaphase In metaphase, the spindle has captures all the chromosomes and lined them up at the middle of the cell, ready to divide. All chromosomes align at the metaphase plate (not a physical structure, just a term for the plane where the chromosomes line up.) At this stage, the two kinetochores of each chromosome should be attached to microtubules form opposite spindle poles Before proceeding to anaphase, the cell will check to make sure that all the chromosomes are at the metaphase plate with their kinetochore correctly attached to microtubules. This is called the Spindle Checkpoint and helps ensure that the sister chromatids will split evenly between the two daughter cell s when they separate in the next step. If the chromosomes is not properly aligned, the cell will halt division until the problem is fixed. Anaphase The sister chromatids separate from each other and are pulled towards opposite ends of the cell. The protein “glue” that hols the sister chromatids together is broken down, allowing them to separate. Each is now its own chromosome. The chromosomes of each pair are pulled towards opposite ends of the cell. Microtubules not attached to chromosomes elongate and push apart, separating the poles and making the cell longer. All of these processes are driven by motor proteins , molecular machines that can “walk: along microtubule tracks and carry a cargo. In mitosis, motor proteins carry chromosomes or other microtubules as they walk. Telophase The cell is nearly done dividing, and it starts to re- establish its normal structures as cytokinesis ( division of the cell contents) take place. The mitotic spindle is broken down into its building blocks. Two new nuclei form, one for each set of chromosomes. Nuclear membranes and nucleoli reappear The chromosomes begin to decondense and return to the “stringy” form. Cytokinesis The division of the cytoplasm to form two cells, ovelaps with the final stages of mitosis. It may start in either anaphase or telophase, depending on the cell, and finishes shortly after telophase. In animal cells, cytokinesis is contractile, pinching the cell in two like a coin purse with a drawstring. The drawstring is a band of filaments made of a protein called actin and the pinch crease is known as the cleavage furrow. In Plant cells can’t be divided like this because they have a cell wall and are too stiff. Instead, a structure called cell plate forms down the middle of the cell, splitting it into two daughter cells separated by a new wall. Regulation of the Cell Cycle The control of the cell cycle is necessary for the normal function of the process. 1. The cell cycle were not regulated, cells could constantly undergo cell division. 2. Internal regulation of the cell cycle is necessary to signal passage form one phase to the next at appropriate times. Overview: The Key Roles of Cell Division The ability of organisms to produce more of their own kind best distinguishes living things from nonliving matter The continuity of life is based on the reproduction of cells, or cell division Figure 12.1 In unicellular organisms, division of one cell reproduces the entire organism Multicellular organisms depend on cell division for – Development from a fertilized cell – Growth – Repair Cell division is an integral part of the cell cycle, the life of a cell from formation to its own division Figure 12.2 100 μm (a) Reproduction 200 μm (b) Growth and development 20 μm (c) Tissue renewal Figure 12.2a 100 μm (a) Reproduction Figure 12.2b 200 μm (b) Growth and development Figure 12.2c 20 μm (c) Tissue renewal Concept 12.1: Most cell division results in genetically identical daughter cells Most cell division results in daughter cells with identical genetic information, DNA The exception is meiosis, a special type of division that can produce sperm and egg cells Cellular Organization of the Genetic Material All the DNA in a cell constitutes the cell’s genome A genome can consist of a single DNA molecule (common in prokaryotic cells) or a number of DNA molecules (common in eukaryotic cells) DNA molecules in a cell are packaged into chromosomes Figure 12.3 20 μm Eukaryotic chromosomes consist of chromatin, a complex of DNA and protein that condenses during cell division Every eukaryotic species has a characteristic number of chromosomes in each cell nucleus Somatic cells (nonreproductive cells) have two sets of chromosomes Gametes (reproductive cells: sperm and eggs) have half as many chromosomes as somatic cells Distribution of Chromosomes During Eukaryotic Cell Division In preparation for cell division, DNA is replicated and the chromosomes condense Each duplicated chromosome has two sister chromatids (joined copies of the original chromosome), which separate during cell division The centromere is the narrow “waist” of the duplicated chromosome, where the two chromatids are most closely attached Figure 12.4 Sister chromatids Centromere 0.5 μm During cell division, the two sister chromatids of each duplicated chromosome separate and move into two nuclei Once separate, the chromatids are called chromosomes Figure 12.5-1 Chromosomal Chromosomes DNA molecules 1 Centromere Chromosome arm Figure 12.5-2 Chromosomal Chromosomes DNA molecules 1 Centromere Chromosome arm Chromosome duplication (including DNA replication) and condensation 2 Sister chromatids Figure 12.5-3 Chromosomal Chromosomes DNA molecules 1 Centromere Chromosome arm Chromosome duplication (including DNA replication) and condensation 2 Sister chromatids Separation of sister chromatids into two chromosomes 3 Eukaryotic cell division consists of – Mitosis, the division of the genetic material in the nucleus – Cytokinesis, the division of the cytoplasm Gametes are produced by a variation of cell division called meiosis Meiosis yields nonidentical daughter cells that have only one set of chromosomes, half as many as the parent cell Concept 12.2: The mitotic phase alternates with interphase in the cell cycle In 1882, the German anatomist Walther Flemming developed dyes to observe chromosomes during mitosis and cytokinesis Phases of the Cell Cycle The cell cycle consists of – Mitotic (M) phase (mitosis and cytokinesis) – Interphase (cell growth and copying of chromosomes in preparation for cell division) Interphase (about 90% of the cell cycle) can be divided into subphases – G1 phase (“first gap”) – S phase (“synthesis”) – G2 phase (“second gap”) The cell grows during all three phases, but chromosomes are duplicated only during the S phase Figure 12.6 INTERPHASE G1 S (DNA synthesis) s is ne i is ok G2 yt s to MIT C Mi (M) OTI PHA C SE Mitosis is conventionally divided into five phases – Prophase – Prometaphase – Metaphase – Anaphase – Telophase Cytokinesis overlaps the latter stages of mitosis Figure 12.7 10 μm G2 of Interphase Prophase Prometaphase Metaphase Anaphase Telophase and Cytokinesis Centrosomes Chromatin Fragments Nonkinetochore (with centriole pairs) (duplicated) Early mitotic Aster of nuclear microtubules Metaphase Cleavage Nucleolus spindle Centromere envelope plate furrow forming Plasma Nucleolus Nuclear membrane Chromosome, consisting Kinetochore Kinetochore Nuclear envelope of two sister chromatids microtubule Spindle Centrosome at Daughter envelope one spindle pole chromosomes forming Figure 12.7a G2 of Interphase Prophase Prometaphas e Centrosomes Fragments (with centriole Chromatin Early mitotic Aster of nuclear Nonkinetochor pairs) (duplicated spindle envelope e ) Centromer microtubules e Plasma Nucleolus membrane Kinetochore Kinetochore Chromosome, Nuclear consisting microtubule envelope of two sister chromatids Figure 12.7b Metaphase Anaphase Telophase and Cytokinesis Metaphase Cleavage Nucleolus plate furrow forming Nuclear Spindle Centrosome at Daughter envelope one spindle pole chromosomes forming Figure 12.7c 10 μm G2 of Interphase Prophase Prometaphase Figure 12.7d 10 μm Metaphase Anaphase Telophase and Cytokinesis Figure 12.7e Figure 12.7f Figure 12.7g Figure 12.7h Figure 12.7i Figure 12.7j The Mitotic Spindle: A Closer Look The mitotic spindle is a structure made of microtubules that controls chromosome movement during mitosis In animal cells, assembly of spindle microtubules begins in the centrosome, the microtubule organizing center The centrosome replicates during interphase, forming two centrosomes that migrate to opposite ends of the cell during prophase and prometaphase An aster (a radial array of short microtubules) extends from each centrosome The spindle includes the centrosomes, the spindle microtubules, and the asters During prometaphase, some spindle microtubules attach to the kinetochores of chromosomes and begin to move the chromosomes Kinetochores are protein complexes associated with centromeres At metaphase, the chromosomes are all lined up at the metaphase plate, an imaginary structure at the midway point between the spindle’s two poles Figure 12.8 Centrosome Aster Metaphase Sister plate chromatids (imaginary) Microtubules Chromosomes Kineto- chores Centrosome 1 μm Overlapping nonkinetochore microtubules Kinetochore microtubules 0.5 μm Figure 12.8a Kinetochores Kinetochore microtubules 0.5 μm Figure 12.8b Microtubules Chromosomes Centrosome 1 μm In anaphase, sister chromatids separate and move along the kinetochore microtubules toward opposite ends of the cell The microtubules shorten by depolymerizing at their kinetochore ends Figure 12.9 EXPERIMENT Kinetochore Spindle pole Mark RESULTS CONCLUSION Chromosome movement Microtubule Kinetochore Motor protein Tubulin subunits Chromosome Figure 12.9a EXPERIMENT Kinetochore Spindle pole Mark RESULT S Figure 12.9b CONCLUSION Chromosome movement Microtubule Kinetochore Motor protein Tubulin subunits Chromosome Nonkinetochore microtubules from opposite poles overlap and push against each other, elongating the cell In telophase, genetically identical daughter nuclei form at opposite ends of the cell Cytokinesis begins during anaphase or telophase and the spindle eventually disassembles Cytokinesis: A Closer Look In animal cells, cytokinesis occurs by a process known as cleavage, forming a cleavage furrow In plant cells, a cell plate forms during cytokinesis Figure 12.10 (a) Cleavage of an animal cell (SEM) (b) Cell plate formation in a plant cell (TEM) 100 μm Cleavage furrow Vesicles Wall of parent cell forming 1 μm cell plate Cell plate New cell wall Contractile ring of Daughter cells microfilaments Daughter cells Figure 12.10a (a) Cleavage of an animal cell (SEM) 100 Cleavage μm furrow Contractile ring of Daughter cells microfilaments Figure 12.10b (b) Cell plate formation in a plant cell (TEM) Vesicles Wall of parent cell 1 μm forming cell plate Cell plate New cell wall Daughter cells Figure 12.10c Cleavage furrow 100 μm Figure 12.10d Vesicles Wall of parent 1 μm forming cell cell plate Figure 12.11 Chromatin Nucleus condensing 10 μm Nucleolus Chromosomes Cell plate 1 Prophase 2 Prometaphase 3 Metaphase 4 Anaphase 5 Telophase Figure 12.11a Chromatin Nucleus condensing Nucleolus 10 μm 1 Prophase Figure 12.11b Chromosomes 10 μm 2 Prometaphase Figure 12.11c 10 μm 3 Metaphase Figure 12.11d 10 μm 4 Anaphase Figure 12.11e 10 μm Cell plate 5 Telophase Binary Fission in Bacteria Prokaryotes (bacteria and archaea) reproduce by a type of cell division called binary fission In binary fission, the chromosome replicates (beginning at the origin of replication), and the two daughter chromosomes actively move apart The plasma membrane pinches inward, dividing the cell into two © 2011 Pearson Education, Inc. Figure 12.12-1 Origin of Cell wall replication Plasma membrane E. coli cell Bacterial chromosome 1 Chromosome Two copies replication of origin begins. Figure 12.12-2 Origin of Cell wall replication Plasma membrane E. coli cell Bacterial chromosome 1 Chromosome Two copies replication of origin begins. 2 Replication Origi Origi continues. n n Figure 12.12-3 Origin of Cell wall replication Plasma membrane E. coli cell Bacterial chromosome 1 Chromosome Two copies replication of origin begins. 2 Replication Origi Origi continues. n n 3 Replication finishes. Figure 12.12-4 Origin of Cell wall replication Plasma membrane E. coli cell Bacterial chromosome 1 Chromosome Two copies replication of origin begins. 2 Replication Origi Origi continues. n n 3 Replication finishes. 4 Two daughter cells result. The Evolution of Mitosis Since prokaryotes evolved before eukaryotes, mitosis probably evolved from binary fission Certain protists exhibit types of cell division that seem intermediate between binary fission and mitosis Figure 12.13 Bacterial (a) chromosom Bacteria e Chromosomes Microtubule (b) s Dinoflagellates Intact nuclear envelope Kinetochore microtubule (c) Diatoms and some yeasts Intact nuclear envelope Kinetochore microtubule (d) Most eukaryotes Fragments of nuclear envelope Figure 12.13a Bacterial chromosome (a) Bacteria Chromosomes Microtubule s Intact nuclear envelope (b) Dinoflagellates Figure 12.13b Kinetochore microtubule Intact nuclear envelope (c) Diatoms and some yeasts Kinetochore microtubule Fragments of nuclear envelope (d) Most eukaryotes Concept 12.3: The eukaryotic cell cycle is regulated by a molecular control system The frequency of cell division varies with the type of cell These differences result from regulation at the molecular level Cancer cells manage to escape the usual controls on the cell cycle Evidence for Cytoplasmic Signals The cell cycle appears to be driven by specific chemical signals present in the cytoplasm Some evidence for this hypothesis comes from experiments in which cultured mammalian cells at different phases of the cell cycle were fused to form a single cell with two nuclei Figure 12.14 EXPERIMEN T Experiment 1 Experiment 2 S G1 M G1 RESULTS S S M M When a cell in the S When a cell in the phase was fused M phase was fused with with a cell in G1, a cell in G1, the G1 the G1 nucleus nucleus immediately immediately entered began mitosis—a spindle the S phase—DNA formed and chromatin was synthesized. condensed, even though the chromosome had not been duplicated. The Cell Cycle Control System The sequential events of the cell cycle are directed by a distinct cell cycle control system, which is similar to a clock The cell cycle control system is regulated by both internal and external controls The clock has specific checkpoints where the cell cycle stops until a go-ahead signal is received Figure 12.15 G1 checkpoint Control system S G1 M G2 M checkpoint G2 checkpoint For many cells, the G1 checkpoint seems to be the most important If a cell receives a go-ahead signal at the G1 checkpoint, it will usually complete the S, G2, and M phases and divide If the cell does not receive the go-ahead signal, it will exit the cycle, switching into a nondividing state called the G0 phase Figure 12.16 G0 G1 checkpoint G1 G1 (a) Cell receives a go-ahead (b) Cell does not receive a signal. go-ahead signal. The Cell Cycle Clock: Cyclins and Cyclin-Dependent Kinases Two types of regulatory proteins are involved in cell cycle control: cyclins and cyclin-dependent kinases (Cdks) Cdks activity fluctuates during the cell cycle because it is controled by cyclins, so named because their concentrations vary with the cell cycle MPF (maturation-promoting factor) is a cyclin-Cdk complex that triggers a cell’s passage past the G2 checkpoint into the M phase Figure 12.17 M G1 S G2 M G1 S G2 M G1 MPF activity Cyclin concentratio n Time (a) Fluctuation of MPF activity and cyclin concentration during the cell cycle 1 S G Cdk M Degraded 2 G cyclin G2 Cdk checkpoint Cyclin is degraded Cyclin MPF (b) Molecular mechanisms that help regulate the cell cycle Figure 12.17a M G S G M G S G M G 1 2 1 2 1 MPF activity Cyclin concentration Time (a) Fluctuation of MPF activity and cyclin concentration during the cell cycle Figure 12.17b S G 1 Cdk M Degraded G2 cyclin G2 Cdk checkpoint Cyclin is degraded MPF Cyclin (b) Molecular mechanisms that help regulate the cell cycle Stop and Go Signs: Internal and External Signals at the Checkpoints An example of an internal signal is that kinetochores not attached to spindle microtubules send a molecular signal that delays anaphase Some external signals are growth factors, proteins released by certain cells that stimulate other cells to divide For example, platelet-derived growth factor (PDGF) stimulates the division of human fibroblast cells in culture Figure 12.18 1 A sample of human Scalpels connective tissue is cut up into small pieces. Petri dish 2 Enzymes digest the extracellular matrix, resulting in a suspension of free fibroblasts. 4 PDGF is added 10 μm 3 Cells are transferred to to half the culture vessels. vessels. Without PDGF With PDGF Figure 12.18a 10 μm A clear example of external signals is density-dependent inhibition, in which crowded cells stop dividing Most animal cells also exhibit anchorage dependence, in which they must be attached to a substratum in order to divide Cancer cells exhibit neither density-dependent inhibition nor anchorage dependence Figure 12.19 Anchorage dependence Density-dependent inhibition Density-dependent inhibition 20 μm 20 μm (a) Normal mammalian cells (b) Cancer cells Figure 12.19a 20 μm Figure 12.19b 20 μm Loss of Cell Cycle Controls in Cancer Cells Cancer cells do not respond normally to the body’s control mechanisms Cancer cells may not need growth factors to grow and divide – They may make their own growth factor – They may convey a growth factor’s signal without the presence of the growth factor – They may have an abnormal cell cycle control system A normal cell is converted to a cancerous cell by a process called transformation Cancer cells that are not eliminated by the immune system form tumors, masses of abnormal cells within otherwise normal tissue If abnormal cells remain only at the original site, the lump is called a benign tumor Malignant tumors invade surrounding tissues and can metastasize, exporting cancer cells to other parts of the body, where they may form additional tumors Figure 12.20 Lymph vessel Tumo r Blood vessel Glandular Cancer tissue cell Metastatic tumor 1 A tumor 2 Cancer 3 Cancer cells spread 4 Cancer cells grows cells invade through lymph and may survive from a single neighboring blood vessels to and establish cancer cell. tissue. other parts of the a new tumor body. in another part of the body. Recent advances in understanding the cell cycle and cell cycle signaling have led to advances in cancer treatment Figure 12.21 Figure 12.UN01 INTERPHASE G1 S Cytokinesis Mitosis G2 MITOTIC (M) PHASE Prophase Telophase and Cytokinesis Prometaphase Anaphase Metaphase Figure 12.UN02 Figure 12.UN03 Figure 12.UN04 Figure 12.UN05 Figure 12.UN06