Post Translational Process Lecture 8 PDF
Document Details
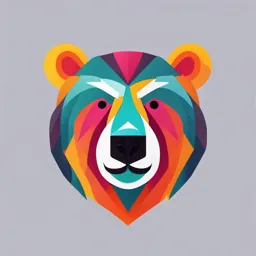
Uploaded by FancyWashington243
Universitas Indonesia
Tags
Related
- BIOL 111 Lecture 7 Protein Structure and Function I (2018) PDF
- Lecture 13: Protein Processing, Targeting, and Degradation PDF
- Proteins Part 2 2024 - PDF
- Transcription, Translation, and Protein Trafficking - PDF
- Protein Structure and Post-Translational Modification PDF
- Abnormal Proteins and Protein Aggregation BMS 532 Lecture Notes PDF
Summary
This document is a lecture on post-translational processes in molecular biology, discussing topics such as protein folding, chaperones, and modifications like phosphorylation, acetylation, and glycosylation. Detailed diagrams and explanations support the concepts presented in the lecture.
Full Transcript
POST TRANSLATIONAL PROCESS Lecture 8 Molecular Biology Department of Biology Faculty of Mathematics and Natural Sciences Universitas Indonesia CONTENTS Introduction to Post Translational Modification Post Translational Events? ...
POST TRANSLATIONAL PROCESS Lecture 8 Molecular Biology Department of Biology Faculty of Mathematics and Natural Sciences Universitas Indonesia CONTENTS Introduction to Post Translational Modification Post Translational Events? Post Translational Modifications (PTM) modifications that occur on a protein, catalysed by enzymes, after its translation by ribosomes is complete. the addition of a functional group covalently to a protein as in phosphorylation and neddylation (ubiquitination), but also refers to proteolytic processing and folding processes necessary for a protein to mature functionally. The components of a protein The 20 amino acids commonly found in proteins Figure 4–2 A protein is made of amino acids linked Figure 4–3 Some of these side chains are nonpolar togetherinto a polypeptide chain. and hydrophobic (“water-fearing”), others are The amino acids are linked by peptide bonds to form a negatively or positively charged, some are reactive, polypeptide backbone of repeating structure (gray and so on. boxes), from which the side chain of each amino acid projects. Long polypeptide chains are very flexible, as many of the peptide bonds that link the carbon atoms in the polypeptide backbone allow free rotation of the atoms they join. Thus, proteins can in principle fold in an enormous number of ways. The shape of each of these folded chains, however, is constrained by many sets of weak noncovalent bonds that form within proteins. These bonds involve atoms in the polypeptide backbone, as well as atoms in the amino acid side chains. The noncovalent bonds that help proteins fold up and maintain their shape include hydrogen bonds, electrostatic attractions, and van der Waals attractions Three types of noncovalent bonds help proteins fold. HOW A PROTEIN FOLDS INTO A COMPACT CONFORMATION? An important factor governing the folding of any protein is the distribution of its polar and nonpolar amino acids. 1. The polar amino acid side chains tend to lie on the outside of the protein, where they can interact with water; 2. The nonpolar amino acid side chains are buried on the inside forming a tightly packed hydrophobic core of atoms that are hidden from water. CONTENTS Introduction to Post Translational Modification Post Translational Events Post Translational Events POST TRANSLATIONAL It is the chemical MODIFICATIONS (PTM) modification of protein after its translation. Key role in functional Proteomics. They regulate activity, localization and interaction with other cellular molecules such as proteins, nucleic acids, lipids and cofactors. Post-Translational Modifications POST TRANSLATIONAL PTMs: MODIFICATIONS (PTM) Protein folding Protein sorting Protein degradation PROTEIN FOLDING CHAPERONE-ASSISTED PROTEIN FOLDING Chaperone proteins assist the proper folding of many proteins. Chaperone proteins have multiple functions: 1. they prevent a polypeptide from folding too early, 2. aid the correct folding at the proper time, 3. promote the correct folding of a misfolded polypeptide, 4. assist the unfolding of certain proteins. PROTEIN FOLDING Co-translational folding is facilitated by ribosome- associated chaperones Although some proteins fold autonomously after release from the ribosome, most require the actions of the ATP-dependent Hsp70 or Hsp60 chaperones. The Hsp70 proteins (DnaK) act like a clamp to hold the hydrophobic part of the protein whereas the Hsp60 proteins (GroES + GroEL) form a barrel that provides a protected environment for folding. DISULFIDE BOND FORMATION Many protein molecules are either attached to the outside of a cell’s plasma membrane or secreted as part of the extracellular matrix → disulfide bonds (also called S–S bonds) form as cells prepare newly synthesized proteins for export. A critical step in the folding of some proteins is the formation of one or more disulfide bonds that covalently link pairs of sulfur- containing cysteine residues. Cysteine residues can exist stably either in a reduced form known as a thiol (–SH) or in an oxidized form in which one cysteine residue Disulfide bonds. forms a covalent S-S bond, a disulfide bond, Covalent disulfide bonds form between adjacent with another cysteine. cysteine side chains. These crosslinkages can join either two parts of the same polypeptide chain or two different polypeptide chains. A number of secreted and membrane proteins in both bacteria and eukaryotes contain disulfide bonds. (a) In bacteria, the oxidoreductase DsbA catalyzes disulphide bond formation in the periplasm. DsbA is reoxidized by DsbB which transfers the reducing equivalents to coenzyme Q from which the reducing equivalents are transferred to the respiratory chain and ultimately to oxygen during aerobic growth. (b) In eukaryotes, sulfhydryl oxidoreductases denoted protein disulphide isomerases (PDIs) catalyze disulfide bond formation in the endoplasmic reticulum. In yeast, the PDI is reduced by FAD-dependent oxidases Ero1p and Erv2p. Again the reducing equivalents are ultimately transferred to oxygen. Proteins required for protein disulfide bond formation. SORTING MOTIFS Protein localization in the cell depends on recognition of a diverse set of amino acid sequences within proteins that are recognized by the various sorting and targeting machineries. Sorting motifs → sorting signals or signal sequences in some cases, are sequence identifiers within proteins that target the proteins to the appropriate localization machinery. Sorting motifs may be present at either the N- or C-terminus end of a protein (or both) and can be specific amino acids, or a patch of amino acids with specific features. PROTEIN SORTING General mechanism of protein translocation across a membrane. The sorting motif (red) of the protein to be translocated can be recognized by the receptor as the protein is being translated or after synthesis is complete. If the protein is folded it may need to be unfolded by a chaperone protein. After the signal sequence is recognized and the protein is brought to the membrane, it is threaded through the translocon. The protein can be pushed across the membrane as it is being extruded from the ribosome or it may be pulled across the membrane by the refolding promoted by chaperones on the other side of the membrane. PROTEIN SORTING The nuclear localization sequence (NLS) is recognized by soluble nuclear import receptors that also bind to components of the nuclear pore complex. After passing through the aqueous pore, the GTP-bound Ran protein stimulates release of the imported protein from the receptor. For proteins trafficking out of the nucleus, binding of an export receptor to a protein with a nuclear export signal (NES) is promoted by Ran-GTP. This tri-partite complex passes through the pore, whereupon release of the exported protein from the receptor is stimulated by hydrolysis of the GTP bound to Ran. Transport in and out of the eukaryotic cell nucleus. POST TRANSLATIONAL CLEAVAGE OF THE POLYPEPTIDE CHAIN Some polypeptides must be cleaved in Chymotrypsin generation by order to become biologically active. specific cleavage. The chymotrypsin enzyme Ex: For many proteins in bacteria and eukaryotes, is translated as an inactive the removal of this N-terminal methionine by a methionine aminopeptidase is a critical step in their precursor called maturation. chymotrypsinogen in pancreatic cells. Proteolytic processing is widely used for Chymotrypsinogen is then the timely activation of polypeptides. transported to the gut, Ex: Chymotrypsin → inactive precursor called where a protease cleaves chymotrypsinogen, transported to the gut where a the inactive precursor at protease cleaves the inactive precursor at specific specific amino acid amino acid positions to generate the active positions to generate the chymotrypsin enzyme. active enzyme. Some proteins contain self-excising The three fragments generated by the proteolysis domains called inteins. remained connected by disulfide bonds. Post Translational Cleavage of The Polypeptide Chain Insulin maturation by specific cleavage and Autoprocessing reactions disulfide bond formation. catalyzed by proteins. The inactive (a) Self-excision catalyzed preproinsulin precursor by inteins. Key contains a signal nucleophilic attacks sequence (red), which are catalyzed by directs the co- conserved serine, translational import of the cysteine or threonine protein into the residues. endoplasmic reticulum. (b) Autoprocessing There the signal reaction catalyzed by sequence is cleaved off. the Hedgehog protein. Three disulfide bonds are In this case, the key formed. Subsequently, nucleophilic attack is cleavage by three catalyzed by an additional proteases exogenous cholesterol gives rise to active molecule. insulin. LIPID MODIFICATION OF PROTEIN Covalent attachment of lipids targets proteins to membranes : 1. acylation by fatty acyl groups such as myristoyl or palmitoyl 2. prenylation by isoprenoid groups such as farnesyl or geranylgeranyl 3. addition of glycoinositol phospholipids (referred to as glycosylphosphatidylinositol (GPI) anchors). Lipid Modification of Protein Acylation processes target the N- and C-termini of proteins The relatively rare 14-carbon saturated fatty acid myristoyl is attached to proteins at the N-terminus via a stable amide linkage to an N-terminal glycine residue, yielding an N-myristoylated protein (N refers to the resulting linkage to nitrogen). The more common 16-carbon saturated palmitoyl is generally attached posttranslationally to C- terminal proximal cysteines through their nucleophilic sulfurs. Lipid Modification of Protein Prenylation is a stable modification of C- terminal cysteine residues Prenylation is the modification of a protein through the addition of a group belonging to the isoprenoid family – generally either a C15 farnesyl or C20 geranylgeranyl group – via a thioether linkage to the sulfur of a cysteine residue. Almost all prenylated proteins are small GTP-binding proteins. Ex: Ras proteins bearing both lipid modifications associate with the plasma membrane, whereas removal of the palmitoyl group to leave just the farnesyl group causes Ras to be targeted to the Golgi membrane instead. Lipid Modification of Protein Subcellular localization of Ras is regulated by farnesylation and palmitoylation. Ras proteins bearing both farnesyl and palmitoyl groups are localized to the plasma membrane whereas Ras proteins with only a farnesyl group are localized to the Golgi membrane. GPI anchors are comprised of both carbohydrates and lipids The GPI anchor is assembled in the endoplasmic reticulum through a complex series of enzymatic steps. The final step involves attachment of the GPI anchor to the C-terminus of the protein with simultaneous cleavage of the protein’s transmembrane segment so that the protein is only tethered to the membrane via the GPI anchor. The presence of the carbohydrate moiety directs GPI-anchored proteins to the cell surface via transport through the secretory pathway. Hedgehog (Hh) proteins are composed Lipid Modification of Protein of two distinct domains, the amino- terminal 'Hedge' domain (HhN), and the carboxy-terminal 'Hog' domain (HhC) Lipid modifications of the Hedgehog protein. The Hedgehog signalling protein undergoes autocatalytic cleavage that is accompanied by the attachment of a cholesterol molecule to the exposed C-terminus of the N-terminal domain. A second lipid modification, the palmitoylation of the N- terminal cysteine residue completes the processing of this signaling domain. GLYCOSYLATION OF PROTEINS Glycosylation can change the solubility of a protein and provide protein recognition sites → the most complex and diverse post-translational modifications. Protein glycosylation serves a variety of functions: 1. Inside the cell, the attachment of glycans generally increases the solubility of nascent glycoproteins and can prevent their aggregation. 2. On the cell surface, can also be bound by other proteins surrounding the cell that recognize specific glycans; this type of interaction commonly plays a role in the recognition of one cell by another. Glycosylation of Proteins Protein glycosylation in the endoplasmic reticulum and Golgi. The oligosaccharide is first transferred from its membrane-bound dolichol pyrophosphate (DPP) carrier to a polypeptide chain while the latter is still being synthesized on the ribosome. As the polypeptide chain grows within the endoplasmic reticulum, monosaccharides may be cleaved from the ends of the oligosaccharide. After completion of the synthesis of the polypeptide chain, the immature glycoprotein is transported to the Golgi apparatus where further modification occurs. New monosaccharides may be added and others removed in a multi-step process involving many different enzymes. The completed glycoprotein is finally transported to its ultimate destination. Yellow hexagons represent N-acetyl-glucosamine, blue hexagons represent mannose and red hexagons represent glucose. PROTEIN PHOSPHORYLATION, ACETYLATION, AND METHYLATION Small reversible covalent modifications play central roles throughout biology. Phosphorylation can alter protein function. Ex: both eukaryotic and bacterial genomes that are devoted to phosphorylation; about 2% of all human genes encode kinases that phosphorylate serine, threonine, or tyrosine residues. Proteins can be acetylated on side chains and at their amino termini. A variety of side chains can be methylated. Specific modifications are recognized by specialized protein domains. Thus phosphorylated tyrosines are bound by SH2 domains, acetylated lysines are bound by bromodomains and methylated lysines are bound by chromodomains. PROTEIN PHOSPHORYLATION, ACETYLATION, AND METHYLATION One example of this is tyrosine phosphorylation (which is fundamental for JAK-STAT signalling), but STATs experience other modifications, which may affect STAT behaviour in JAK- STAT signalling. These modifications include: methylation, acetylation and serine phosphorylation. The Jak–STAT signaling pathway. The JAK/STAT pathway is an Ligand binding induces cytokine receptor chain oligomerization. Weakly active Jaks important cascade of signal associated with the receptor cross-activate one another, probably by phosphorylation transduction for multiple growth of the activating site in their kinase domains. The resulting activated Jaks then factors and cytokines, which phosphorylate key tyrosines in the cytoplasmic tail of the cytokine receptor. These sites regulates gene expression and cell attract signaling molecules, including STATs and other signaling proteins containing activation, proliferation, and differentiation (Reddy et al., 2019; SH2 domains (only STAT is shown). Bound STATs become phosphorylated, after which Xin et al., 2020; Awasthi et al., they dissociate from the receptor and dimerize via their phosphorylation sites and the 2021). SH2 domains. The STAT dimers rapidly enter the nucleus and activate transcription of specific target genes. PROTEIN PHOSPHORYLATION, ACETYLATION, AND METHYLATION Reversible acetylation of lysine side chains. Acetyl transferase enzymes catalyzed the transfer of an acetyl group from acetylCoA to the amino group of lysine (for histones, acetyl transferases are denoted HATs). The acetyl group can be removed in two different ways by deacetylases (for histones, deacetylases are denoted HDACs) or by NAD- dependent sirtuins. PROTEIN PHOSPHORYLATION, ACETYLATION, AND METHYLATION Reversible methylation of lysine residues. Lysine may be mono-, di-, or tri-methylated by methyl transferases. Mono- and di-methylated lysines can be demethylated by amino oxidases. The methyl donor is S-adenosylmethionine (SAM) resulting in the production of S-adenosylhomocysteine (SAH). PROTEIN PHOSPHORYLATION, ACETYLATION, AND METHYLATION Reversible acetylation of arginine residues. Unmethylated and mono-methylated arginine can be converted to citrulline or methylated citrulline by the peptidyl arginine deaminase PADI4. PROTEIN PHOSPHORYLATION, ACETYLATION, AND METHYLATION Direct chemical modification of some amino acids can have both regulatory and detrimental effects. Sulfur-containing amino acids are particularly sensitive to various forms of oxidation. Proteins can be modified by reaction with nitric oxide. Examples of amino acid nitration. Formation of S- nitrosylcysteine and O-nitrated tyrosine. PROTEIN DEGRADATION UBIQUITINATION OF PROTEINS Covalent modifications by ubiquitin and related proteins are required for a variety of cellular functions. → Ubiquitin was initially identified as the modification that marked proteins for proteolytic degradation in the cell by a large multiprotein complex known as the proteasome Ubiquitin attachment and chain elongation occur through a series of enzymatic Ubiquitination. steps. (a) Isopeptide bond between ubiquitin and a lysine side Different types of ubiquitin chain. (b) Three-dimensional structure of ubiquitin. modifications have distinct functional consequences UBIQUITINATION OF PROTEINS Steps in protein ubiquitination. Protein ubiquitination begins with the ATPdependent covalent attachment of the C- terminus of the 76-residue ubiquitin protein to the ubiquitin-activating enzyme (E1), which then transfers the ubiquitin to one of a family of ubiquitin-conjugating enzymes (E2). The E2 then collaborates with a target-specific ubiquitinprotein ligase (E3) to catalyze the formation of a peptide bond between the activated C-terminal glycine of ubiquitin and a lysine side chain on the target protein. Additional ubiquitins can then be added to other lysines on the target or on ubiquitin itself, sometimes resulting in multiple ubiquitin polymers on the protein surface. UBIQUITINATION OF PROTEINS Functional consequences of different ubiquitin modifications. Each type of polyubiquitin chain is classified according to the lysine side chain in ubiquitin to which the C- terminus of the next ubiquitin is covalently attached. Each type of linkage is denoted by a different color, as indicated atop each bar. Post-Translational Modifications Summary Post-Translational Modifications (PTMs) are chemical changes that proteins experience as a result of their covalent attachment to functional groups or proteins and the cleavage of their peptide bonds. These modifications alter the structure of individual proteins and therefore, potentially affect their activity, stability, localization and/or interacting partner molecules. Chemical alterations that usually occur during the post translational modification of proteins include phosphorylation, methylation, acetylation, ubiquitination, nitrosylation, glycosylation, and lipidation. Since these modifications constitute a central mechanism that regulates protein levels and function allowing cells to rapidly respond to developmental or environmental stimuli, PTMs are important determinants of cell biology including processes like signal transduction, development, cell structure, mitosis, DNA modification, cancer, neurodegeneration, and others. https://www.youtube.com/watch?v=AeVDoDp3llI END OF THIS TOPIC