Cable Television Broadcast Review PDF
Document Details
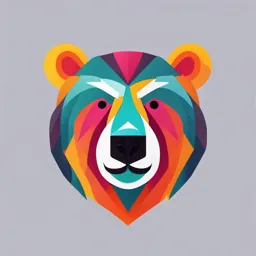
Uploaded by InnovativeAppleTree599
Saint Louis University
Tags
Summary
This document details cable television systems, covering topics like cable frequencies, adjacent channels, midband and superband channels, and tuning to cable channels. It also discusses cable radiation and other related topics, providing an overview of cable TV technologies.
Full Transcript
Cable television started as a means of providing signals to communities that could not receive broadcast stations, either because of distance or shadow areas in which the signal was too weak. Then a community antenna was used at a remote location to feed TV signals to receivers in the area. Today, c...
Cable television started as a means of providing signals to communities that could not receive broadcast stations, either because of distance or shadow areas in which the signal was too weak. Then a community antenna was used at a remote location to feed TV signals to receivers in the area. Today, cable TV has developed far beyond that into huge systems that cover large areas, even for locations having good reception. The reason is that cable TV does not have the restriction of channel allocations for broadcasting. The cable systems offer up to 36 channels. A cable converter box permits selection of the desired channel. Premium pay services such as Home Box Office, Spotlight, Prism, Cinemax, and other also offer current movies and sports event not available on broadcast television. Cable Frequencies Many older cable systems distribute TV signals on the same VHF channel frequencies that are used for broadcasting. The UHF channels are converted to VHF channels for distribution because cable losses are too high in the UHF band. This method is a 12-channel system, including the lowband and highband VHF channels 2 to 13. Subscribers in the system do not need a converter. Direct cable connections are made to the TV receiver, where the RF tuner can be used to select the desired channel. Adjacent Cable Channels with a 12-channel system, some receivers may have adjacent channel interference, since all the VHF channels are used. The ability to reject adjacent- channel frequencies depends on the IF selectivity of the receiver. The interference produces a windshield-wiper or venetian-blind effect in the picture. In the cable system, interference is minimized by balancing the signals for all channels at a common level. Also, compared with TV broadcast signals, the sound carrier level is usually much lower than the picture carrier signal. Midband and Superband Cable Channels Since the cable signal is not radiated, at least not intentionally, the cable system can use frequencies that are assigned to other radio services without interference. Therefore, the midband cable channels are used in the gap between VHF channels 6 and 7. These frequencies from 88 to 174 MHz include 88 to 108 MHz for the FM radio broadcast band plus various marine and aircraft communications services. However, the FM radio band generally is not used for TV cable channels. As listed in table 15-1, the midband cable channels start with number 14 or letter designation A for 120 to 126 MHz, with the video or picture carrier frequency set at 121.25 MHz. Although not listed, the sound carrier frequency is automatically 4.5 MHz higher, or 125.75 MHz. Included are channel numbers 14 to 22 or letters A to I. Additional midband channels are number 00, 01, and 54 to 59. Channels 00 and 01 are above the FM radio band. Double digits are used for all cable channel numbers to allow for a digital control board for tuning. Channels 54 to 59 occupy spot frequencies in gaps of the regular midband channel allotments. Superband just means cable TV channels above the VHF broadcast channel 13. This band starts with cable channel letter J or number 23. The letters continue to Z and numbers to 53. The use of VHF broadcast channels 2 to 13 and cable channels 14 to 37 provides 12 + 24 = 36 channels in a typical large cable TV system. These frequencies are up to approximately 300 MHz. Systems using the higher cable channels up to 400 MHz are more sophisticated. They require special cable and better amplifiers with closer spacing to offset greater losses at higher frequencies. Tuning to the Cable Channels In conventional TV receivers, the RF tuner usually is not made to select the midband and superband cable channels. Therefore, the cable operator provides a separate converter unit. It converts all cable frequencies to a designated VHF channel, such as channel 2, 3, or 4. The subscriber keeps the receiver tuned to the specified channel, and all channel selection is done at the converter. Cable-Ready TV Receivers Many late-model receivers offer a tuner that can select the midband and superband cable channels directly without the need for a converter. However, there is another practical problem. The premium pay services usually have a signal that is scramble electronically. The circuits required for descrambling are build into the converter or attached to it. As a result, the system-oriented converter would be needed anyway to watch the scrambled- signal premium channels. Cable Radiation The cable operator must be especially careful that the system does not radiate TV signals. Radiation can occur if cables are open, short-circuited, or even partially mismatched at their termination. Damage to the cables may result from strong winds, storms, or other accidents. To detect radiation, a selected midband channel may be used just for an FM tone-modulated indicator signal. Then a simple portable FM radio can be used as a “sniffer” to locate any radiation, just by riding along the cable route in the service truck. Some midband channels are particularly sensitive to the radiation problem. For instance, the band for channel A or 14 includes the aircraft distress frequency at 121.5 MHz. Some cable operations may not use that channel when the possibility of interference exists. Harmonically Related Channels Cable systems have the option of operating slightly off the frequencies assigned for TV broadcasting, but close enough to allow TV receivers and converters to tune to the frequencies. One such choice is harmonically related channels (HRCs), where all the picture carrier frequencies are an integral multiple of 6 MHz. These are listed in table 15-2. Note that channel 04 with 66-MHz harmonic picture carrier is only 1.25 MHz away from the 67.25-MHz broadcast carrier frequency. The advantage of using the HRC system in cable systems is simplification of the frequency synthesis circuits used for tuning at the head end and in the converter. Coaxial Cable for CATV The conduit used for distributing the CATV signals at RF channel frequencies is coaxial cable. It is an efficient wideband transmission line that has the advantage of shielding. There are several types of coaxial line, generally called coax, but all are constructed as in fig. 15-1. A central wire is surrounded by a cylindrical or tubular conductor, and two conductors are separated by an insulator. The type of cable generally used in a main signal route, called a trunk line is shown in figure 15.2. It consists of a heavy central aluminum conductor that is copper-clad, meaning that it is coated with copper. The outer conductor or shield is also aluminum and is shaped in a solid tube. A polyethylene foam fills the internal space and supports the inner conductor exactly at the center. The cable diameter is about ¾ in. (19.1mm). Some other types of trunk cable are hollow, with the inner conductor supported by plastic beads at regular spacings. The larger the cable diameter, the less the attenuation. However, large cables are not flexible and are difficult to install. For installations with a long, unsupported span of cable, a steel messenger cable is encased within the outer jacket. The steel wire actually supports the weight of the cable. Trunk cables also are made with a waterproof polyethylene jacket for use underground or underwater. In addition, armored cables with spiral layer of steel are used. In some systems, two cables are combined in a single outer jacket. These are called Siamese cables. They can be used in two-cable systems in which each cable carries different programs in the range of channel 2 to channel 13. Then, the system has a capacity of 24 channels without the need for a cable converter. A simple two-position A-B switch can be used by the subscribers to choose one cable or the other, for 12 channels on each. The cable used in the branch lines from the main trunk are similar to that in Fig. 15.2 but smaller in diameter. Thinner cable can be used because the run is not too long. The line from a branch to the subscriber is called a drop line. The drop line is generally RG- 59U coaxial cable, as shown in Fig. 15.3. This cable is flexible because a copper braid is used for the outer shield. Its diameter is ¼ in (6.35 mm), including the outer polyethylene jacket to make the cable weatherproof. The F connector at end Is standard for CATV use. Characteristic Impedance Coaxial cable is one type of transmission line, which means a line with uniform distance between the two conductors. Any transmission line has a characteristic impedance, or surge impedance, because of the constant conductor spacing. The symbol for the surge impedance is Zo. Different types of line have different values of Zo, but for any one type Zo is the same for any length of line. The coaxial cable used for CATV in general, and the RG-59U specifically, has a characteristic impedance of 72 to 75 ohms. Generally, 75 ohms is considered as the nominal value. Note that 50 ohms coaxial cable also is common in applications for communications equipment such as CB radio. Also, for the flat twin-lead cable generally used for antenna input to TV receivers Zo=300 ohms. Although not coaxial cable, the twin-lead cable is a transmission line. The Zo is resistive, without a reactive component, but it is an ac value that cannot be measured with the ohmmeter. However, you can check the low dc resistance for continuity to see whether the line is open. What is the meaning of Zo? As the name says, Zo, is a characteristic for a particular type of line. Its value depends on the size of conductors, their spacing, and the type of insulator between them. In general, wider spacing with the same conductors provides a higher Zo. One definition of Zo is the impedance the line would have as a load connected to an ac voltage source at one end and infinitely long at the other end. The line must be considered infinitely long so that no energy can be reflected from the end to interfere with the energy supplied by the source to the line. It may seem impractical to have an infinitely long line, but the same effect can be produced by terminating the end with a resistance equal to Zo Consider the equivalent circuit of a transmission line shown in figure. This circuit is a lumped-constant model, for L and C represent the concentrated values of inductance and capacitance for any unit length of the line, corresponding to the L and C distributed over that length. The battery in figure 15.4 represents a dc source just to apply energy, and the meter give readings of the voltage and current. When the switch S is closed, each of the capacitances C is charged by the voltage source. Since C is uncharged initially, the capacitance is like a short circuit as it takes a high charging current. However, the series L opposes the increase in current. So, a finite time is taken to charge C1 in the model. As C1 charges to some value of V, it serves as a source to charge the next section with L2 and C2. Since the L and C values are extremely small, the charging process propagates down the line very rapidly. The velocity is close to the speed of light in space. Still, the voltage across the two conductors travels as a wavefront out to the end of the line. Assume now, that the line in fig 15.4 is infinitely long. If such a line could be made, it would go on charging forever, toward the open end. At the input end connected to the source, though, the voltage and current readings settle at specific values of V and I. This ratio of V/I is the characteristic impedance of that line. Terminating the Line in Zo Suppose that we cut the infinitely long line at a point 10ft (3m) from the switch and then connect across the open end of the line a resistor having the same value as the characteristic impedance of the line. Now, when the line capacitance is fully charged, the resistor at the end will produce the same meter readings for V and I. The line terminated with R equal to Zo acts just like an infinitely long line. For the source that feeds the line, the load impedance is the same. Most important ac signals, all the energy in the line is used at the termination, and no signal is reflected from the end. This factor explains why the distribution lines in CATV must always be terminated in their characteristic impedance, which is generally 75 ohms. Mismatch with an Open or Short-Circuited Line When the line is not terminated in Zo, an impedance mismatch results. Ine extreme case is a line open at the end, as shown in Fig 15.5a. the driving source here is an ac generator to supply signal on the line. Note that the last lumped capacitor on the line, indicated as C 3 has a signal voltage of the same polarity as the source. Therefore, C 3 acts as a source to send a voltage wave back from the end toward the input source. Capacitor C3 sends back a wave of discharge current, in the opposite direction from the incident charging current. These effects are illustrated by the standing-wave patterns of V and I in fig 15.5b. In the line with an open end, therefore, standing waves of V and I are set up along the line. A standing wave means that different points along the line have a specific V and I as an ac signal in either peak or root mean square values. A maximum on the standing wave occurs where the incident and reflected values have the same phase, with respect to distance along the line. A node occurs where the incident and reflected values are out of phase and so cancel. With respect to time, however, the ac values of V and I are continuously changing. For a specific frequency of ac signal on the line, the distances can be considered in terms of the wavelength. One-quarter wave back from the open end, the voltage waves are 180 out of phase, and a voltage null results. The current is additive here, though, which makes I double the value of a matched line. At this point on the line, therefore, it is equivalent to a series resonant circuit. The reason is that I is high and V is low for a specific frequency. The relative values of V and I reverse at quarter-wave distances back to the source. At each peak or null on the standing wave, the V/I ratio for Z is resistive and has a maximum or minimum value. At the intermediate points, the line is reactive and has intermediate values of impedance. The conclusion is then, that an open coaxial cable acts as a tuned circuit. Such a resonant response means different results for different frequencies. Furthermore, all the energy supplied to a lossless line would be reflected to the source at the input. When a long line runs are used, the resulting time delay in the reflected signals can produce ghosts in the TV picture. Note that id the driving source does not have a 75 ohms impedance, then the signal reflected from the open end is also reflected again from the source, which creates multiple ghosts. The example of a line short-circuited at the end is shown in Fig 15.5c. The last component on the line is inductance L3. Since C3 is bypassed by the short circuit at the end. After a current peak has been produced by the driving source, the inductance tends to keep the current flowing. Therefore, energy is reflected from the end of the line. This time, though, the reflected current is in the same direction as the incident current. Also, the polarity of the self- induced voltage is reversed. The resulting standing waves of V and I are shown in Fig 15.5d. They correspond to the standing waves in 15.5b for the open line, but the V and I patterns are reversed in terms of peaks and nulls. In summary, an open end always has high V and low I because of the capacitance of an open circuit. A quarter-wave back, conditions reverse with a peak of I and null of V. For the opposite case, a short-circuited end always has high I and low V because of the short circuit. Conditions reverse with a peak of V and null of I a quarter-wave back. The standing-wave pattern continues the reversals for every quarter-wave. Remember that high I and low V for a specific frequency create the same effect as series resonance. Also, high V and low I mean high Z for the effect of a parallel resonant circuit. These effects illustrate why quarter-wave and half-wave sections of transmission lines can be used as low-loss tuned circuits, particularly for wave traps, at frequeheadncies in the VHF and UHF bands. Furthermore, the fact that a mismatched line has standing waves means that the amount of signal at the end depends on where you cut the line. A foot more or less can make a big difference. If a line is correctly terminated in its Zo, however, its length is not critical. Voltage Standing-Wave Ratio The examples of an open, or short-circuited lines are the extremes of mismatch, in which almost all the energy is reflected from the end. However, reflection also occurs with a poor termination not equal to the characteristic impedance Zo. Then part of the energy is reflected. As a result, the voltage is less than double at the peaks in the standing-wave pattern, and the nulls are more than zero. For this reason, the degree of cable match can be expressed as the ratio of voltage at the peaks to voltage at the nulls. The proportion is the voltage standing-wave ratio. When the line is perfectly matched with a termination equal to its own Zo, there are no standing waves. Then Vmax and Vmin are equal, and the VSWR=1. Any other termination however yields a VSWR greater than 1. Cable Losses In our imaginary lossless line, all energy sent down the line from the source is consumed in the terminating load. With practical lines, though some energy is dissipated in the line itself. The result is attenuation of the signal. There are three causes of attenuation: 1. I2R losses produced by current in the conductors. 2. Dielectric losses in the insulator between conductors. Remember that signals are high radio frequencies in the VHF band. 3. Skin effect. The Rf current flows more on the circumference of the conductor than its center. Because of the smaller area for current, the ac resistance of the conductor increases. The aluminum cable in Fig 15-2 has a copper coating around the inside conductor to reduce losses from the skin effect. Losses Increase with Frequency Specifically, the losses increase in proportion to the square root of frequency. For a practical case, compare channel 13 at 210 to 216 MHz which is about four time higher in frequency than channel 2 at 54 to 60 MHz. At 4f, the line losses for channel 13 equal sqrt4, or double the losses for channel 2. The values of cable attenuation for several different type of CATV cable are listed in Table 15-3 for frequencies from 5 to 500 MHz. Cable Distance Designers of cable distribution systems think in terms of signal attenuation per unit distance of line. For example, suppose that a particular cable has an attenuation of 1dB per 100 ft(30.5m). the loss is 6 dB for 600ft and 20 dB for 2000ft. Incidentally a 6 dB loss in voltage means one-half the signal. Rather than continuously make the conversion from decibel loss to distance, it is more convenient to work in terms of cable loss directly. In out example, the cable 2000ft long is referred to as a 20dB run. It is the function of the trunk amplifiers to make up for the cable losses and restore signal levels to standard values throughout the cable system. Cable Distribution System Refer to Fig 15-6. The starting point for cable signals is called the head end. Here the broadcast signals picked up by the antenna are amplified, adjusted for level, and fed into the trunk lines. The UHF channels are converted to VHF channels. Also included are local- origination signals from a studio. The video and audio signals modulate separate carriers in a VHF channel not being used. The main routes of signal from the head end are the trunk lines. Trunk Amplifiers The trunk amplifiers are inserted at regular intervals along the trunk route to make up for cable losses. For example, in Fig. 15-7, a 20 dB amplifier is placed at the end of a cable run with a loss of 20dB. The decibel unit used for cable signals is dBmV, which means decibels above 1mV. Amplifiers are placed at regular intervals to keep the signal up to the standard level of 1 to 3mV. Figure 15-8 shows a weatherproof housing for a trunk amplifier in an aerial system, which is mounted on a pole. Power is obtained from a tap on electric service line on the same pole. Bridging Amplifiers This type of amplifier is for a branch from the main trunk to feed a particular neighborhood in the cable system. The typical gain is 20 to 40 dB. The output is for the branch lines to individual subscribers. In many cases, the trunk and bridging amplifiers are located in the same weatherproof housing. An additional attenuator may be used at the input to the bridging amplifier to balance the signal levels. Line Amplifiers Long line runs from the bridging amplifier may require that line extender amplifiers be inserted in the branch line to make up for cable losses in that branch. This amplifier extends the number of drop lines that can be used on a branch line. The typical gain for a line amplifier is 20 to 40 dB. Directional Couplers Signal power taken from the trunk must be kept very small so that the line is not loaded by all the branches. The device used to tap off the signal is a directional coupler. Its construction is illustrated in Fig 15-9a, and its symbol is shown in 15-9b. It is a three-terminal device. One terminal is for signal input. Another carries the signal through the trunk line. The third terminal has tapped output signal for a branch. The directional coupler is so named because it feeds a sample of the direct, downstream signal out at the tap but ignores reflected energy in the trunk line. This is accomplished by a small loop placed in the wall of the coaxial assembly, as shown in Fig 15-9a. The loop is terminated with a 75-ohms resistor. The loop acts as both a capacitor and an inductor. Its capacitance charges to the potential difference between the inner and outer conductors at that point on the line. As a one-turn coil, the loop is magnetically coupled to the center conductor to tap off that signal. Directional couplers have a very small insertion loss between the input and output signals on the trunk line. A typical value is -1dB for insertion loss at 300 MHz. The tap loss from input to output at the tap is typically -13dB, but this loss is made up in the bridging amplifier. Power Supplies The cable amplifiers are solid-state and require little power. The power supplies may be placed at long intervals and the cable itself used to carry the dc power. Input for the power supply is 120V ac tapped from the power line on the same pole in an aerial system. The typical dc supply voltage for the cable amplifiers is 24V. The power supply module often is located in the same weatherproof housing that encloses the trunk and bridging amplifiers. In some cases, storage batteries are kept on constant charge with two 12V batteries in series to make 24V. They are switched into service when there is a failure in ac power. Line Taps The final tap on the system feeds the drop line for the subscriber, usually with RG-59U cable. Figure 15-10 shows a multitap with four taps for four houses close to one another. As with directional couplers, the line tap has a low insertion loss but high tap loss. The tap-to-tap loss is made high to provide isolation between the individual subscriber lines. Isolation is necessary so that a misterminated cable at the subscriber’s TV receiver will not setup reflections in the cable system. Two possible terminations are a cable that is not connected or leads that are short-circuited. The tap units available with many values of tap loss, so the signal levels can be balanced for different subscriber drop lines along the branch. Balun Units The word balun is actually an abbreviation of balanced-to-unbalanced connections. A typical unit is shown in Fig 15-11a. Most TV receivers are designed for 300-ohms balanced input at the antenna connections, for twin-lead transmission line with neither side grounded. The coaxial cable in a CATV system, however, is single-ended or unbalanced with one side grounded. The balun is used to match the 75-ohms coaxial cable to the 300-ohms receiver input. As shown in Fig 15-11b, the balun is constructed as two sections of 150-ohms line, usually coiled to make the unit smaller. The lines are connected in parallel at the 75-ohms side and in series at the 300-ohm side. Actually, the balun can match the impedances in either direction. F connector The coaxial connector for the 75-ohm line in Fig 15-11a is the standard F connector used in cable work. Its advantage is that no soldering is required. The solid center wire of the cable is the center pin of the connector. Also, the grounded sleeve slides into the braided shield of the cable. Either the sleeve of the plug is screwed onto the jack, or a press-fit connector can be used. Many TV receivers have an input jack for an F connector so that a direct cable connection can be made without the need for a balun. The dBmV Unit for Losses and Gains Because the cable signal voltages are always measured across the same 75 ohms impedance, it is convenient to express voltage levels in decibels. The reference used for CATV is 1 mV across 75 ohms. With this reference, the units are indicated as dBmV. This reference is an arbitrary value, but 1 mV happens to be just about the minimum signal voltage measured across 75 ohms that a receiver needs for a noisefree picture. Across 300 ohms, the minimum is 2V. Distortion in the Cable Signal The cable amplifiers should provide maximum signal to make up for the cable losses and still provide a good signal-to-noise ratio for no snow in the picture. However, the signal levels must be held within narrow limits because the amplifiers produce serious distortion with excecssive signal. Harmonic Distortion When an amplifier is overdriven, harmonic distortion appears in the output. The harmonics are multiples of the signal frequencies being amplified. Harmonic distortion is actually a result of amplitude distortion, for changes in the waveform generate new frequencies. Second-order harmonics are not too serious in a 12-channel system because the second harmonic of channels 2 to 6 falls in the range of 110 to 176 MHz. These frequencies are in the midband range between channels 6 and 7. The harmonics can cause interference problems, however, in the cable systems using midband and superband channels. The cable amplifiers are push-pull operation. Second harmonics generated in the push-pull circuit are canceled in the output. The cancellation is effective but not complete because perfectly balanced push-pull circuits are difficult to achieve. Cross Modulation Third-order harmonics and the effects of cross modulation are more serious results of overload distortion. The nonlinear amplifier characteristic causes an overload amplifier stage to operate as a mixer stage, the input signals are detected, and the modulation is extracted. A transfer of the modulation to another carrier frequency can then occur. The effect of cross modulation on the picture is a jumble of lines and the appearance of video from another channel. The interfering picture may be a negative. It shows most clearly when the channel you are watching goes to black between commercials. However, the easiest symptom of cross modulation to recognize is the maximum modulation of the interfering signal, which is sync. This appears as vertical bars in the picture. The bars may be stationary or may drift across the picture, depending on the stability of the sync generator for the interfering channel. Note that the bars are produced by sync and blanking pulses. Spurious Signals Another effect of amplifier overload is the production of spurious frequencies that are not harmonically related to the desired signal. Harmonics of all the signal frequencies, caused by the mixer action with nonlinear operation. Furthermore, these frequencies can beat with each other. The result is a wide range of unwanted signal frequencies. Computer solutions to the complex problem of numerical values for the spurious frequencies have shown that a large number show up on channel 4 at 66 to 72 MHz. Temperature Effects Another problem is that the signal level changes because of the effect of temperature on cable losses. The attenuation increases for higher temperatures at an approximate rate of 1 percent per 10F rise. This loss may not seem significant, but in very long cable runs the variation in signal level could be 100 dB in the expected temperature range for the northeast United States. The double trouble is a reduction in signal-to-noise ratio in hot weather and the probability of amplifier overload when it snows. Automatic Gain Control To compensate for temperature changes and other variable factors, some trunk amplifiers have an AGC circuit to vary the gain. A typical arrangement is shown in the block diagram of a trunk amplifier. The system uses a cw pilot carrier inserted at a frequency of 73 MHz is indicated here, but other frequencies are in the range of 72 to 76, 100 to 120, 160 to 174, or 216 to 230 MHz. The narrowband amplifier at the bottom of Fig is tuned to the pilot frequency. Then the AGC detector produces dc control voltage proportional to the amplitude of the pilot signal. The dc control voltage varies the attenuation of the cable signal into the preamplifier. A manual control is also provided by R1 for the initial adjustment Automatic Slope Control The cable attenuation increases for higher frequencies. Therefore, the overall frequency response of the cable tilts down for higher-frequency channels. To compensate for this effect, the frequency response of the amplifier is made to slope upward for the higher channel frequencies. The desired result is a uniform or flat frequency response for signals in all the channels. The automatic slope control is an AGC system designed to increase the amplifier gain just for higher frequencies. The ASC system uses another pilot carrier with a frequency at or near the top of the channel range. In fig. the ASC pilot frequency is 270 MHz. The narrowband amplifier and ASC detector are tuned to this frequency to provide dc control voltage that varies the gain of the slope-control amplifier. A manual control is provided by R2 for the initial adjustment. The R2 control for ASC and R1 for AGC are set to balance the system to achieve a uniform signal on all channels. Then the automatic control circuits can maintain the balance. Two-Way Cable Systems In addition to downstream signals from the head end to subscribers, many cable systems are designed for upstream service from subscribers to the head end. The same cable is used for both directions. However, separate amplifiers are needed for the upstream signal. Upstream communication is in the band of frequencies between 5 and 30 MHz. For the downstream path at the top, diplexers with a high-pass filter are used for the downstream signal. Filtering is necessary to remove any upstream signal. At the bottom of the diagram, low-pass filters separate the return signal in the 5 to 30 MHz band. Only the upstream signal is used in the return amplifier. The converter unit at the subscriber end supplies the upstream signal. Two-Way Applications Some communities permit home burglar alarm systems to be connected to a central receiving station in the return line of the cable system. Most important, the system provides two-way communications with subscribers for billing, surveys, shop-at-home service, and pay-per-view for special programs. The system acts as an electronic box office for collecting money from all the subscribers. The subscriber reply can be made with a few pushbuttons on the cable TV converter unit. As many as 99 coded replies are possible by using a calculator type of keypad. Poling Signal The pay-per-view system requires that a poling signal be sent downstream to communicate with only the appropriate converter units. The frequency of the poling signal is in the107 to 119 MHz range. Frequency-shift keying is used with a bit 16-bit word as modulation for the particular address. When poled, the converter replies with data set up at the keypad. For example, punching in 55 can indicate that the subscriber wants to view a special program that evening. The reply to the poling signal is sent back to the head end by means of a small modulator operating in the 5 to 30 MHz range for the upstream signal. An affirmative reply to the head end sends a coded signal back downstream to operate the descrambler in the subscriber’s converter unit on the correct channel at the specified time. Also, data are centered into the cable operator’s business computer for billing purposes. A digital address for each subscriber is set in a read-only memory. Which cannot be changed when each converter unit is installed. If the converter is stolen, electronic address will show up at the wrong location. Although two-way cable systems are basically simple, they are difficult to put into service practically. The main problem is interference from the upstream signal at 5 to 30 MHz. The entire cable system, covering a huge area, acts as an antenna for the return signal. Particular attention must be paid to proper termination of the lines, good grounding, and low-loss cable fittings. Any parts of the system that radiate signals also pick up interfering frequencies. Elaborate digital switching methods are used to isolate any branches that pick-up interference. A particular problem is that the subscriber’s modulator may be stuck in the transmit mode, which can shut down the entire poling system. Provisions are made to switch to another poling frequency when this trouble occurs. Cable TV Converters The converter om top of the TV set, or close to it, is an RF tuner used to select the desired channel. All the cable channels are heterodyned to a specific frequency band chosen to be one of the lowband VHF channels. Usually, channel 3 or 4 is the choice, depending on which is not an active broadcast channel in the area. However, a simple frequency conversion to channel 3 or 4 presents some problems. For channel 3 conversion, the local oscillator in the converter would operate 61.25 MHz above the selected channel. Note that 61.25 MHz is the picture carrier frequency for channel 3. Suppose that channel 4 is selected by the converter. Then the LO operates at 61.25+67.25=128.5 MHz. This LO frequency is inside the cable midband channel B or 15 at 126 to 132 MHz. In addition. There are several other frequency combinations in which the LO signal can be a source of interference-especially since the broadband preselector in the converter is not very selective for RF tuning owing to the wide range of cable channels. The solution to these problems is to use a double-superheterodyne circuit for the cable converter, with a UHF intermediate frequency. Up-Down Converters Refer to the block diagram in Fig. In this double-superheterodyne circuit, first, the cable channels are heterodyned up to the IF value of 374 to 380 MHz in the UHF band. The oscillator frequencies are not in any band for cable TV channels. Second, the IF signal is converted down to the frequency for either channel 3 or 4. Two mixer stages are used in the up-down catv converter. The first mixer with the varactor- tuned local oscillator converts all incoming channels to the IF value of the converter. The IF band here is 374 to 380 MHz, but some converters use 608 to 614 MHz for the IF signal. A bandpass filter in the output circuit of the up converter selects only the IF signal, which is the desired channel tuned in by the UHF local oscillator. The second mixer, with a fixed frequency oscillator, is the down converter. It heterodynes the IF signal at its UHF values down to either channel 3 or 4 for the TV receiver. An adjustment in this oscillator is set for either output channel. The frequency that is used in the second LO for down conversion stays the same for all channels. The IF signal is always in the fixed IF passband of the converter. Each of the selected channels has already been selected by tuning the first LO frequency in the up converter, which heterodynes all the cable channels up to the IF band. Channel Selection Each channel is tuned by varactor control of the local oscillator frequency in the up converter. A varactor is a capacitive diode. Its capacitance decreases with the amount of reverse dc voltage. Since the frequency is varied by dc bias, the circuit is a voltage-controlled oscillator. A typical arrangement for the cable converter is shown in the block diagram. The pushbutton switches S1 to S6, at the right in the diagram, are identified with the channel numbers. When they are pushed in, the switches inset the variable resistors R1 to R6 into the circuit. The dc bias voltage is supplied through the three-position band switch with sections Sa, SB and Sc. Each potentiometer, or pot is preset to provide dc voltage that makes the VCO operate at the frequency needed to tune in a specific channel. The three-position band switch, with 12 pushbutton switches and 12 potentiometers, allows tuning for 36 channels. Frequency Synthesizer The block diagram shows the complete circuit for an up-down converter using frequency synthesis. The synthesizer section illustrates how the frequency is set for the VCO as the first local oscillator for the up converter. An 8 MHz crystal-controlled oscillator is the reference. The advantage of frequency synthesis is that many oscillator frequencies can be supplies with the accuracy of crystal control. The 8 MHz oscillator in the synthesizer is divided by 1014 to produce 7.8125 kHz signal into a phase detector or comparator. The other input to the comparator is a sample of the signal from the VCO in the up converter. This sample is divided first by 256 and then by a factor N that is set in the programmable counter. The idea is to divide by whatever N is needed to provide 7.8125 kHz for comparison with the reference signal in the phase detector. The factor N can take any value between 334 and 454. The actual count is determined by an 8-bit binary code set with the pushbutton switches on either the counter or a remote unit. Remote operation is provided with a long wire to the converter for convenience of the subscriber. Or a wireless infrared control system can be used, much ass the remote tuner is used for TV receiver. We can calculate the frequencies needed in the converter for a specific example. Superband channel 36 has a video carrier frequency of 295.25 MHz, as listed in the Table. The video IF carrier frequency is 612.75 MHz for the converter. Thus the required VCO frequency, beating above the mixer input signal is. Wave Traps and Scrambling Methods Cable systems offer for a minimum fee the so-called basic service, which include the local TV broadcast channels, some out-of-town stations and local-origination programs. In addition, premium services are offered, such as Home Box Office, spotlight, cinemax, rainbow and others. They feature special sports events and movies uncut and without commercial interruptions. However, these premium channels require a fee to be paid that is added to the basic charge. To service only those subscribers who pay for the service, two techniques are used. One method is to insert a wave trap that attenuates the pay channel. The trap is in the feed line to each subscriber who does not have premium service. The trap method is not used much anymore, though because it requires work on the pole to change the service. Also, the wave traps can be bypassed by illegal tampering at the subscriber tap on the feed line. Today, the preferred method of security is to scramble the signal. The picture is not intelligible unless it is descrambled with a unit supplied by the cable operator Scrambling The most common method of scrambling the signal is known as syn suppression. Sync is compered only in the RF modulation envelope of the video carrier in the cable channel. Then the receiver cannot lock in with the sync-suppressed signal. The picture is usually out of sync, both vertically and horizontally, as evidenced by rolling and diagonal bars. In addition, the loss of sync upsets the receiver AGC circuit and produces the effects of AGC overload distortion. The picture is dark, possibly reversed in white and black values, like a negative, and out of sync. Descrambling The descrambler unit reverses the effect of the scramble at the head end by restoring sync to the RF signal. Sync is restored by means of a keyed RF attenuator bypassed with a diode switch, indicated as Ra and S in the figure. In this method, the pulses needed for the switched attenuator are sent to the descrambler unit by a separate route. A pilot carrier signal having a frequency below that of the channel is used. An example of the pilot carrier frequency is 114 MHz for midband channel A at 120 to 126 MHz. Other choices are possible, though and cable operators choose their own pilot frequencies for security reasons. The descrambler contains a narrowband receiver tuned to the assigned pilot frequency. The receiver has an amplitude detector and pulse-shaping circuits to drive the diode switch. The decoding pulse in the pilot signal is the sync needed for descrambling. As a result, sync is restored in the RF signal for the TV receiver. In-Band Descrambles A more recent method uses the same idea of a pilot signal for descrambling, but the decoding pulses are sent inside the passband of the scrambled channel. These descrambler units are used with the cable converter, connected as shown in the block diagram. For an in- band system, the FM sound carrier signal in a scrambled channel is amplitude-modulated with the decoding pulses. Keeping the percentage of modulation low prevents interference. Since the converter changes all cable channels to a single channel such as 3 or 4, the descrambler unit contains a narrowband receiver tuned just for the sound carrier frequency of the designated channel in the converter output. Other than that, the descrambler operates just as the pilot carrier system. The advantage of the in-band method is that it allows as many scrambled channels as the cable operator wants to offer. Systems with four and more scrambled channels are common. Note that a change in the designated channel output of the converter, from 3 to 4 or vice versa requires a different descrambler. Both the converter and the descrambler must operate on the same channel. Long-Distance Links Large cable systems often cover long distances which could results in prohibitive cable attenuation. Example include the distance from a remote antenna tower to the head end and that from the head end to major hubs, which are the distribution points for local service. The methods used by the cable operator to reduce losses for long-distances links include super trunks, microwave links, and fiber-optic links using light waves. Supertrunks In this method, larger cables are used and the cable channels are heterodyned down to lower frequencies. Both techniques reduce the cable losses. As shown in the figure, the cable channels are changed to 6 to 78 MHz in the down converter. This band has space for 12 channels. Cable losses in the supertrunk are reduced for the lower frequencies, in proportion to the square root of the frequency change. For instance, 54 MHz when reduced by a factor of 9 to 6 MHz, would have one-third the cable losses. Note that a second heterodyne circuit is needed as an up converter in figure, to provide the cable channels at their standard frequencies. From the hub, channels 2 to 12 are fed to the cable distribution system. Microwave Links Frequency allocations by the FCC permit operation in the band of 12.7 to 13.2 GHz. Relay stations of this type are called community antenna relay services. Note that 1 GHz=1000MHz=1x109 Hz. Frequencies in the range of 0.3 to 300 GHz are called the microwave band because the short wavelengths are a fraction of 1m. The advantage of microwave transmission is that parabolic reflector dish antennas can be used to provide very high gain with a very narrow beam. In effect, the energy travels in a pencil-like beam from the transmitter dish antenna to the receiver dish. The line-of-sight transmission requires that no physical obstructions be in the path between transmitter and receiver. Microwave propagation is similar to light radiation, since the microwave frequencies are not much lower. However, microwaves are not visible. Some typical signal levels can be calculated for a microwave link operating at 13 GHz. The transmitter output is 1W. With a 1 mW reference for dBmW units, this power is 30 dBmW. Antenna gain in decibel units for a dish with 6 ft diameter is 43, in either decibels or dBmW. The antenna gain applies at the transmitter and receiver for a total of 85 dBmW. Attenuation of the microwave signal for a 20 mi distance can be taken as -146 dBmW. This value is the approximate free-space loss at 13 GHz, as listed in reference tables. The resulting signal at the receiver is -30 dBmW. The signal power at -30 dBmW below the reference of 1mW is equal to 0.001 mW. In terms of voltatge acoss 75 ohms, V=0.115mV of signal. Special low- noise amplifiers are required for the receiver in the microwave link. Note that individual transmitter-receiver combinations are used for the different cable channels. Either a frequency-modulation link or an amplitude-modulation link can be used. The FML generally has a better signal-to-noise ratio, especially of preemphasis and deemphasis are used for the baseband video signal. However, the AML can actually have superior performance compared with an FM system using a narrow frequency deviation. Fiber Optics The latest type of communications link uses a cable made with thin glass fibers that serve as a conduit for light over long distances with little losses. In this system with a fiber-optic link, the full cable-channel bandwidth can be used for amplitude modulation of the light sources. For transmission, a modulated light beam is the source that introduces light into the glass fibers. At the receiving end, a photoelectric detector converts the variations in light amplitude back to the cable signals. The light serves as a super carrier wave for the entire cable passband. There are important advantages to using fiber-optic cable as a long- distance link. The cable is not as heavy as copper conductors, making it convenient for installation. Attenuation of the light is much less than the losses with conduction or radiation of an RF carrier wave. A big factor is that the exceptionally high frequency for light makes it possible to have modulation that include a tremendous range of frequencies. Refraction and Internal Reflection of Light The reason for the low attenuation is the internal reflection of light inside the fiber cable. Thus, no light can escape and the losses are extremely small. To analyze this effect, the basic laws of refraction of light are illustrated, and internal reflection is shown in figure. Light rays are shown going from air into a slab of glass. The velocity of light is reduced in the glass because it is a denser medium. Light rays enter at an angle from the normal line. This direction is perpendicular to the interface where light enters or leaves the glass. Going into the denser medium, the light rays do not continue in their original angular direction. Instead, they are bent to an angle closer to the normal line because of the reduced velocity of the rays. As each wavefront of light reaches the air-glass interface, the effect is like a squad of people abreast marching in a line. The first one to hit the interface begins going slower, but the last continues with the original speed up to the interface. As a result, the line of the wavefront turns to its right, and the light beam bends toward the normal. At the bottom of the glass slab, the light going out is bent away from the normal. This direction is opposite to the bending of the incident light. The reason is that the light goes to a less dense medium that allows it to travel at higher velocity. The bending of the light is called refraction. How much the light bends when it meets a different medium is determined by the index of refraction, whose symbol is n. Typical values of n are 1 for air or vacuum, 1.8 for glass, 2.4 for diamond, and 1.3 for water. Now consider a light source actually inserted in the slab of glass. The light radiates in all directions. The light ray marked A approaches the interface at a right angle. Such rays along the normal are not refracted. Ray B is refracted but still leaves the glass. Note that refraction bends the light away from the normal line. For ray C, however, the refraction is just enough to make the light follow along the glass surface. At D an smaller angles for the rays, the light is reflected internally. None of these light rays can leave the glass. The angle at which the internal reflection begins is the critical internal angle. The corresponding action is shown for fiber optic cable. Light entering the conduit at angles less than twice the critical angle will be reflected internally. Then the light is propagated along the cable in zigzag direction, bouncing off the walls but without leaving the glass. This angle that allows complete internal reflection is the acceptance angle. All incident light with a smaller angle, or along the central axis, is transmitted in the optic cable. Modal Dispersion Light going into the optical cable or the central axis takes the shortest route. At other angles within the acceptance angle, the light must travel a longer path because of the internal reflections. The time difference between the direct and reflection paths is called the modal dispersion. This factor limits the bandwidth of the cable. To minimize the modal dispersion, practical optical cable is made with fine fibers of small diameter. In addition, the fiber is encased in a cladding material that has a high index of refraction in order to increase internal reflections. In effect, all the fibers are in parallel to provide a cable with very low losses. Optic Transmitter The light source is often a special light-emitting diode, operating in the infrared part of the spectrum, where the wavelength is greater than that for visible light. In construction, the LED is at the bottom of a conical pit to produce light in the cable. The LED current and its light output can be modulated with the full passband of all the cable channels. Actually, the LED is the limiting factor. The bandwidth of the cable itself is far greater than the bandwidth of the LED modulation circuits. Another method of transmitting the optic signal uses an injection laser diode as the light source. This system can accept modulating frequencies well into the UHF range. Cable Connectors One of the problems with fiber optics is splicing, or joining cables. Care must be taken to make precise optical alignment at the connection, or excessive light loss will result. However, special connectors are available to join the cables and still keep light losses within tolerable limits.