Algae - Botany PDF
Document Details
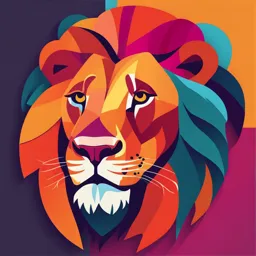
Uploaded by EnviousQuasimodo8732
Tags
Summary
This document provides detailed information about algae, including their classification, characteristics, and use in animal and human diets, and in aquaculture. It also examines the environmental factors affecting algal growth.
Full Transcript
1. Algae Algae have been used in animal and human diets since very early times. Filamentous algae are usually considered as ‘macrophytes’ since they often form floating masses that can be easily harvested, although many consist of microscopic, individual filaments of algal cells. Algae also form...
1. Algae Algae have been used in animal and human diets since very early times. Filamentous algae are usually considered as ‘macrophytes’ since they often form floating masses that can be easily harvested, although many consist of microscopic, individual filaments of algal cells. Algae also form a component of periphyton, which not only provides natural food for fish and other aquatic animals but is actively promoted by fishers and aquaculturists as a means of increasing productivity. This topic is not dealt with in this section, since periphyton is not solely comprised of algae and certainly cannot be regarded as macroalgae. However, some ancillary information on this topic is provided in Annex 2 to assist with further reading. Marine ‘seaweeds’ are macro-algae that have defined and characteristic structures. Microalgal biotechnology only really began to develop in the middle of the last century but it has numerous commercial applications. Algal products can be used to enhance the nutritional value of food and animal feed owing to their chemical composition; they play a crucial role in aquaculture. Macroscopic marine algae (seaweeds) for human consumption, especially nori (Porphyra spp.), wakame (Undaria pinnatifida), and kombu (Laminaria japonica), are widely cultivated algal crops. The most widespread application of microalgal culture has been in artificial food chains supporting the husbandry of marine animals, including finfish, crustaceans, and molluscs. The culture of seaweed is a growing worldwide industry, producing 14.5 million tonnes (wet weight) worth US$7.54 billion in 2007 (FAO, 2009). The use of aquatic macrophytes in treating sewage effluents has also shown potential. In recent years, macroalgae have been increasingly used as animal fodder supplements and for the production of alginate, which is used as a binder in feeds for farm animals. Laboratory investigations have also been carried out to evaluate both algae and macroalgae as possible alternative protein sources for farmed fish because of their high protein content and productivity. Microalgae and macroalgae are also used as components in polyculture systems and in remediation; although these topics are not covered in this paper, information on bioremediation is contained in many publications, including Msuya and Neori (2002), Zhou et al. (2006) and Marinho-Soriano (2007). Red seaweed (Gracilaria spp.) and green seaweed (Ulva spp.) have been found to suitable species for bioremediation. The use of algae in integrated aquaculture has also been recently reviewed by Turan (2009). 1.1 Classification The classification of algae is complex and somewhat controversial, especially concerning the blue-green algae (Cyanobacteria), which are sometimes known as blue-green bacteria or Cyanophyta and sometimes included in the Chlorophyta. These topics are not covered in detail this document. However, the following provides a taxonomical outline of algae. Archaeplastida Chlorophyta (green algae) Rhodophyta (red algae) Glaucophyta Rhizaria, Excavata Chlorarachniophytes Use of algae and aquatic macrophytes as feed in small-scale aquaculture – A review Euglenids Chromista, Alveolata Heterokonts Bacillariophyceae (diatoms) Axodine Bolidomonas Eustigmatophyceae Phaeophyceae (brown algae) Chrysophyceae (golden algae) Raphidophyceae Synurophyceae Xanthophyceae (yellow-green algae) Cryptophyta Dinoflagellates Haptophyta The following sections discuss the characteristics and use of both ‘true’ algae and the Cyanophyta, hereinafter referred to as ‘blue-green algae’). 1.2 Characteristics Filamentous algae and seaweeds have an extremely wide panorama of environmental requirements, which vary according to species and location. Ecologically, algae are the most widespread of the photosynthetic plants, constituting the bulk of carbon assimilation through microscopic cells in marine and freshwater. The environmental requirements of algae are not discussed in detail in this document. However, the most important parameters regulating algal growth are nutrient quantity and quality, light, pH, turbulence, salinity and temperature. Macronutrients (nitrate, phosphate and silicate) and micronutrients (various trace metals and the vitamins thiamine (B1), cyanocobalamin (B12) and biotin) are required for algal growth (Reddy et al., 2005). Light intensity plays an important role, but the requirements greatly vary with the depth and density of the algal culture. The pH range for most cultured algal species is between 7 and 9; the optimum range is 8.2–8.7. Marine phytoplankton are extremely tolerant to changes in salinity. In artificial culture, most grow best at a salinity that is lower than that of their native habitat. Salinities of 20–24 ppt are found to be optimal. Lapointe and Connell (1989) suggested that the growth of the green filamentous alga Cladophora was limited by both nitrogen and phosphorus, but the former was the primary factor. Hall and Payne (1997) found that another green filamentous alga, Hydrodictyon reticulatum, had a relatively low requirement for dissolved inorganic nitrogen in comparison with other species. Rafiqul, Jalal and Alam (2005) found that the optimum environment for Spirulina platensis under laboratory conditions was 32 ºC, 2 500 lux and pH 9.0. Further information on the environmental requirements of algae cultured for use in aquaculture hatcheries is contained in Lavens and Sorgeloos (1996). The environmental requirements of cultured seaweeds are discussed by McHugh (2002, 2003). A brief description of some of the filamentous algae and seaweeds that have been used for feeding fish, as listed in Tables 1.1–1.3, is provided in the following subsections. 1.2.1 Filamentous algae Filamentous algae are commonly referred to as ‘pond scum’ or ‘pond moss’ and form greenish mats upon the water surface. These stringy, fast-growing algae can cover a pond with slimy, lime-green clumps or mats in a short period of time, usually beginning their growth along the edges or bottom of the pond and ‘mushrooming’ to the surface. Individual filaments are a series of cells joined end to end which give the Algae thread-like appearance. They also form fur-like growths on submerged logs, rocks and even on animals. Some forms of filamentous algae are commonly referred to as ‘frog spittle’ or ‘water net’. Spirulina, which is a genus of cyanobacteria that is also considered to be a filamentous blue-green algae, is cultivated around the world and used as a human dietary supplement, as well as a whole food. It is also used as a feed supplement in the aquaculture, aquarium, and poultry industries (Figure 1.1). Figure 1.1 Figure 1.2 Spirulina sp. Spirogyra sp. Source: scienceblogs.com/energy/2008/08/big_ Source: Wim van Egmont© energy_fr... Spirogyra, one of the commonest green filamentous algae (Figure 1.2), is named because of the helical or spiral arrangement of the chloroplasts. There are more than 400 species of Spirogyra in the world. This genus is photosynthetic, with long bright grass-green filaments having spiral-shaped chloroplasts. It is bright green in the spring, when it is most abundant, but deteriorates to yellow. In nature, Spirogyra grows in running streams of cool freshwater, and secretes a coating of mucous that makes it feel slippery. This freshwater alga is found in shallow ponds, ditches and amongst vegetation at the edges of large lakes. Under favourable conditions, Spirogyra forms dense mats that float on or just beneath the surface of the water. Blooms cause a grassy odour and clog filters, especially at water treatment facilities. Cladophora (Figure 1.3) is a green filamentous algae that is a member of the Ulvophyceae and is thus related to the sea lettuce (Ulva spp.). The genus Cladophora has one of the largest number of species within the macroscopic green algae and is also among the most difficult to classify taxonomically. This is mainly due to the great variations in appearance, which are significantly affected by habitat, age and environmental conditions. These algae, unlike Spirogyra, do not conjugate (form bridges between cells) but simply branch. Figure 1.3 Figure 1.4 Cladophora sp. Water net (Hydrodictyon sp.) Source: Biopix.dk© Source: silicasecchidisk.conncoll.edu Use of algae and aquatic macrophytes as feed in small-scale aquaculture – A review Another green filamentous alga, Hydrodictyon, commonly known as ‘water net’, belongs to the family Hydrodictyaceae and prefers clean, eutrophic water. Its name refers to its shape, which looks like a netlike hollow sack (Figure 1.4) and can grow up to several decimetres. 1.2.2 Seaweeds Ulva are thin flat green algae growing from a discoid holdfast that may reach 18 cm or more in length, though generally much less, and up to 30 cm across. The membrane is two cells thick, soft and translucent and grows attached (without a stipe) to rocks by a small disc-shaped holdfast. The water lettuce (Ulva lactuca) is green to dark green in colour (Figure 1.5). There are other species of Ulva that are similar and difficult to differentiate. Figure 1.5 Figure 1.6 Sea lettuce (Ulva lactuca) Eucheuma cottonii Source: Mandy Lindeberg (www.seaweedsofalaska.com) Source: www.actsinc.biz/seaweed.html It is important to recognize that the genera Eucheuma and Kappaphycus are normally grouped together; their taxonomical classification is contentious. These are red seaweeds and are often very large macroalgae that grow rapidly. The systematics and taxonomy of Kappaphycus and Eucheuma (Figure 1.6) is confused and difficult, due to morphological plasticity, lack of adequate characters to identify species and the use of commercial names of convenience. These taxa are geographically widely dispersed through cultivation (Zuccarello et al., 2006). These red seaweeds are widely cultivated, particularly to provide a source of carageenan, which is used in the manufacture of food, both for humans and other animals. Gracilaria is another genus of red algae (Figure 1.7), most well-known for its economic importance as a source of agar, as well as its use as a food for humans. Figure 1.7 Figure 1.8 Gracilaria sp. Porphyra tenera Source: Eric Moody© (Wikipedia) Source: http://www.fao.org/fishery/species/search/en Algae The red seaweed Porphyra (Figure 1.8) is known by many local names, such as laver or nori, and there are about 100 species. This genus has been cultivated extensively in many Asian countries and is used to wrap the rice and fish that compose the Japanese food sushi, and the Korean food gimbap. It is also used to make the traditional Welsh delicacy, laverbread. 1.3 Production As in the case of their environmental conditions, the methods for culturing filamentous algae and seaweeds vary widely, according to species and location. This topic is not covered in this review but there are many publications available on algal culture generally, such as the FAO manual on the production of live food for aquaculture by Lavens and Sorgeloos (1996). Concerning seaweed culture, the following summary of the techniques used has been has been extracted from another FAO publication (McHugh, 2003) and further reading on seaweed culture can also be found in McHugh (2002). Some seaweeds can be cultivated vegetatively, others only by going through a separate reproductive cycle, involving alternation of generations. In vegetative cultivation, small pieces of seaweed are taken and placed in an environment that will sustain their growth. When they have grown to a suitable size they are harvested, either by removing the entire plant or by removing most of it but leaving a small piece that will grow again. When the whole plant is removed, small pieces are cut from it and used as seedstock for further cultivation. The suitable environment varies among species, but must meet requirements for salinity of the water, nutrients, water movement, water temperature and light. The seaweed can be held in this environment in several ways: pieces of seaweed may be tied to long ropes suspended in the water between wooden stakes, or tied to ropes on a floating wooden framework (a raft); sometimes netting is used instead of ropes; in some cases the seaweed is simply placed on the bottom of a pond and not fixed in any way; in more open waters, one kind of seaweed is either forced into the soft sediment on the sea bottom with a fork-like tool, or held in place on a sandy bottom by attaching it to sand-filled plastic tubes. Cultivation involving a reproductive cycle, with alternation of generations, is necessary for many seaweeds; for these, new plants cannot be grown by taking cuttings from mature ones. This is typical for many of the brown seaweeds, and Laminaria species are a good example; their life cycle involves alternation between a large sporophyte and a microscopic gametophyte-two generations with quite different forms. The sporophyte is what is harvested as seaweed, and to grow a new sporophyte it is necessary to go through a sexual phase involving the gametophytes. The mature sporophyte releases spores that germinate and grow into microscopic gametophytes. The gametophytes become fertile, release sperm and eggs that join to form embryonic sporophytes. These slowly develop into the large sporophytes that we harvest. The principal difficulties in this kind of cultivation lie in the management of the transitions from spore to gametophyte to embryonic sporophyte; these transitions are usually carried out in land-based facilities with careful control of water temperature, nutrients and light. The high costs involved in this can be absorbed if the seaweed is sold as food, but the cost is normally too high for production of raw material for alginate production. Where cultivation is used to produce seaweeds for the hydrocolloid industry (agar and carrageenan), the vegetative method is mostly used, while the principal seaweeds used as food must be taken through the alternation of generations for their cultivation. Use of algae and aquatic macrophytes as feed in small-scale aquaculture – A review 1.4 Chemical composition A summary of the chemical composition of various filamentous algae and seaweeds is presented in Table 1.1. Algae are receiving increasing attention as possible alternative protein sources for farmed fish, particularly in tropical developing countries, because of their high protein content (especially the filamentous blue-green algae). The dry matter basis (DM) analyses reviewed in Table 1.1 show that the protein levels of filamentous blue green algae ranged from 60–74 percent. Those for filamentous green algae were much lower (16–32 percent). The protein contents of green and red seaweeds were quite variable, ranging from 6–26 percent and 3–29 percent respectively. The levels reported for Eucheuma/ Kappaphycus were very low, ranging from 3–10 percent, but the results for Gracilaria, with one exception, were much higher (16–20 percent). The one analysis for Porphyra indicated that it had a protein level (29 percent) comparable to filamentous green algae. Some information on the amino acid content of various aquatic macrophytes is contained in Annex 1. The lipid levels reported for Spirulina (Table 1.1), with one exception (Olvera- Novoa et al. (1998), were between and 4 and 7 percent. Those for filamentous green algae varied more widely (2–7 percent). The lipid contents of both green (0.3–3.2 percent) and red seaweeds (0.1–1.8 percent) were generally much lower than those of filamentous algae. The ash content of filamentous blue-green algae ranged from 3–11 percent but those of filamentous green algae were generally much higher, ranging from just under 12 percent to one sample of Cladophora that had over 44 percent. The ash contents of green seaweeds ranged from 12–31 percent. Red seaweeds had an extremely wide range of ash contents (4 to nearly 47 percent) and generally had higher levels than the other algae shown in Table 1.1. 1.5 Use as aquafeed Several feeding trials have been carried out to evaluate algae as fish feed. Algae have been used fresh as a whole diet and dried algal meal has been used as a partial or complete replacement of fishmeal protein in pelleted diets. 1.5.1 Algae as major dietary ingredients A summary of the results of selected growth studies on the use of fresh algae or dry algae meals as major dietary ingredients for various fish species and one marine shrimp is presented in Table 1.2. Dietary inclusion levels in these studies varied from 5 to 100 percent. Fishmeal-based dry pellets or moist diets were used as control diets. The results of the earlier growth studies showed that the performances of fish fed diets containing 10–20 percent algae or seaweed meal were similar to those fed fishmeal based standard control diet. The responses were apparently similar for most of the fish species tested. These inclusion levels effectively supplied only about 3–5 percent protein of the control diet. In most cases, these control diets contained about 26–47 percent crude protein. This shows that only about 10–15 percent of dietary protein requirement can be met by algae without compromising growth and food utilization. There was a progressive decrease in fish performance when dietary incorporation of algal meal rose above 15–20 percent. However, although reduced growth responses were recorded with increasing inclusion of algae in the diet, the results of feeding trials with filamentous green algae for O. niloticus and T. zillii indicated that SGR of 60–80 percent of the control diet could be achieved with dietary inclusion levels as high as 50–70 percent. Recent work by Kalla et al. (2008) appears to indicate that the addition of Porphyra spheroplasts to a semi-purified red seabream diet improved SGR. In addition, Valente et al. (2006) recorded improvements in SGR when dried Gracilaria busra-pastonis replaced 5 or 10 percent of a fish protein hydrolysate diet for European seabass. Table 1.1 Chemical analyses of some common algae and seaweeds Algae Algae/ seaweed Moisture Proximate composition1 Minerals1 Reference (percent) (percent DM) (percent DM) CP EE Ash CF NFE Ca P Filamentous blue-green algae Spirulina maxima, spray dried powder 6.0 63.8 5.3 9.6 n.s. n.s. n.s. Henson (1990) Spirulina, commercial dry powder 3-6 60.0 5.0 7.0 7.0 21.0 n.s. n.s. Habib et al. (2008) Spirulina spp., dry powder n.s. 55-70 4-7 3-11 3-7 n.s. n.s. Habib et al. (2008) Spirulina maxima, dry powder, Mexico 10.2 73.7 0.7 10.5 2.1 13.0 n.s. n.s. Olvera-Nova et al. (1998) Filamentous green algae Spirogyra spp., fresh, USA 95.2 17.1 1.8 11.7 10.02 n.s. n.s. Boyd (1968) Cladophora glomerata, meal, Scotland 1.6 31.6 5.2 23.6 11.2 28.4 n.s. n.s. Appler and Jauncey (1983) Cladophora sp., fresh, USA3 90.5 15.8 2.1 44.3 13.3 24.54 n.s. n.s. Pine, Anderson and Hung (1989) 2 Hydrodictyon reticulatum, fresh, USA 96.1 22.8 7.1 11.9 18.1 n.s. n.s. Boyd (1968) Hydrodictyon reticulatum, meal, Belgium 5.7 27.7 1.9 32.6 14.9 22.9 n.s. n.s. Appler (1985) Green seaweeds Ulva reticulata, fresh, Tanzania n.s. 25.7 n.s. 18.3 38.5 n.s. 0.1 Msuya and Neori (2002) Ulvaria oxysperma, dried, Brazil 16-20 6-10 0.5-3.2 17-31 3-12 n.s. n.s. Pádua, Fontoura and Mathias (2004) Ulva lactuca, dried, Brazil 15-18 15-18 1.2-1.8 12-13 9-12 n.s. n.s. Pádua, Fontoura and Mathias (2004) Ulva fascita, dried, Brazil 18-20 13-16 0.3-1.9 17-20 9-11 n.s. n.s. Pádua, Fontoura and Mathias (2004) Red seaweeds Eucheuma cottonii, fresh, Indonesia 91.3 4.9 0.4 43.5 8.4 42.84 0.5 0.2 Tacon et al. (1990) Eucheuma cottonii, dry powder, Malaysia 10.6 9.8 1.1 46.2 5.9 37.04 0.3 n.s. Matanjun et al. (2009) Eucheuma denticulatum, fresh, Tanzania n.s. 7.6 n.s. 46.6 22.3 n.s.