BMS 200 Week 6 Vessel Physiology - PDF
Document Details
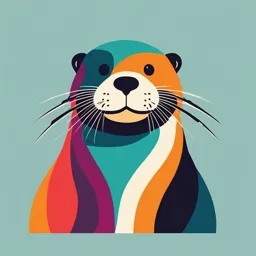
Uploaded by UserReplaceableQuail
SFU
Tags
Summary
This document details the physiology of blood vessels, from the macro- to microcirculation. It covers the histology, function, and regulation of different types of blood vessels, including arteries, arterioles, capillaries, venules, and veins. The document also reviews Poiseuille's Law and discusses the role of the autonomic nervous system and the renin-angiotensin-aldosterone system in regulating blood pressure.
Full Transcript
BMS 200 – Macrocirculatory and Microcirculatory Physiology Objectives Describe the relationship between venous return, cardiac output, mean arterial pressure, pulse pressure, systolic pressure, diastolic pressure, and systemic vascular resistance Relate the following characteristics of elastic arte...
BMS 200 – Macrocirculatory and Microcirculatory Physiology Objectives Describe the relationship between venous return, cardiac output, mean arterial pressure, pulse pressure, systolic pressure, diastolic pressure, and systemic vascular resistance Relate the following characteristics of elastic arteries, muscular arteries, arterioles, capillaries, venules, and veins to their physiologic role Compliance and elasticity Pressure and fluid velocity Cross-sectional area and distribution of total blood volume Describe the histological structure of elastic arteries, muscular arteries, arterioles, capillaries, venules, and veins Describe the mechanisms of venous return and relate it to cardiac preload Describe the cellular physiology of the major baroreceptors and relate it to the overall function of the baroreceptor reflex Apply Poiseuille’s law to the regulation of blood pressure Compare turbulent to laminar flow and describe situations when each would occur Objectives Explain why arterioles are the major site of blood pressure regulation Relate the histological structures of the microcirculation to their physiologic function Contrast the locations as well as the functional and structural differences between continuous, fenestrated, and sinusoidal capillaries Describe the how Starling forces determine bulk flow across the capillary endothelium and relate filtration to the development of edema and inflammation Describe the major mechanisms of autoregulation in the microcirculation and how it suffices to meet the needs of the tissue Describe the role of the autonomic nervous system and the renin- angiotensin-aldosterone system in the homeostatic control of blood pressure Contrast the regulation of blood flow in the following vascular beds: Cerebral, pulmonary, coronary, renal, skeletal muscle, cutaneous Simulating hemorrhage You can simulate hemorrhage by placing a patient in a chamber that applies negative pressure to the lower body ▪ Why is this system a smart experimental system? ▪ What would be the impact on the cardiac system once the vacuum is turned on? ▪ Think about this for end of lecture https://www.sfu.ca/aerospacelab/research-areas/ orthostatic-intolerance/lower-body-negative-pressure.html Blood Vessel Histology – General Concepts The walls of arteries and veins contain 3 distinct layers: ▪ Tunica intima ▪ Tunica media ▪ Tunica externa Blood Vessel Histology – General Concepts Tunica Intima: continuous sheet of simple squamous endothelial cells (endothelium) lining the lumen various amounts of subendothelial connective tissue (CT) Internal elastic lamina, a thin layer of elastic fibers, forms the outermost boundary of the tunica intima Blood Vessel Histology – General Concepts Tunica Media: Thickest layer in arteries Circularly arranged smooth muscle cells and fibroelastic CT ▪ elastic content increases greatly with the size of the vessel External elastic lamina, an elastic fiber-rich layer, forms the outermost boundary of the tunica media Blood Vessel Histology – General Concepts (Externa) Tunica Adventitia: outermost layer of the vessel wall, consisting of dense irregular CT In larger vessels, the tunica adventitia houses vasa vasorum ▪ small blood vessels that supply the tunica adventitia and media * thickest of the three layers in veins * Arterial Vessels – General Characteristics Points of Note ▪ Elastic arteries have a greater number of elastic - membranes for dealing with high-pressure blood flow me item from the heart. ▪ Muscular arteries have more smooth muscle layers for - - regulating blood flow to various regions of the body. ▪ Arterioles control the flow into capillary beds and regulate blood pressure through constriction or dilation. ▪ Metarterioles act as transitional vessels between arterioles and capillaries, controlling blood flow into - capillaries. - Arteries – Elastic and Muscular Elastic arteries: - More elastic fibres ▪ relatively thin external elastic lamina ▪ Very pulsatile, and are the major pressure reservoirs of the circulation ▪ Designed to handle high-pressure blood flow near the heart Muscular arteries: - More smooth muscle ▪ Most of the arteries of the body that are named are muscular arteries ▪ Still pulsatile, but do not serve a major pressure reservoir function ▪ Although a large layer of smooth muscle, cannot completely vasoconstrict and “cut off” blood flow, and not the major source of -peripheral resistance - ▪ Distribute blood to various organs and tissues, controlling flow through smooth muscle contraction Arteries – Elastic and Muscular Elastic Arteries (Conducting Arteries): · Aorta 3 Pulmonary arteries (pulmonary trunk) Common carotid arteries Subclavian arteries Common iliac arteries Arteries – Elastic and Muscular Muscular Arteries (Distributing Arteries): 53 Radial artery Femoral artery Brachial artery Coronary arteries Popliteal artery Muscular artery & accompanying vein Arterioles & Metarterioles Arterioles Control systemic blood pressure and direct blood flow into capillary beds through smooth muscle constriction and dilation. Metarterioles Serve as a microvascular control point, regulating blood flow into individual capillaries through precapillary sphincters, fine-tuning the supply to meet local tissue demands. Arterioles & Metarterioles Major sites of regulation in the cardiovascular system ▪ Arterioles can constrict significantly and restrict blood flow to a capillary bed ▪ Pre-capillary sphincters at the junction of metarterioles and capillaries can greatly reduce flow to a capillary bed AND metarterioles can shunt blood directly to venules Types of capillaries Continuous capillaries: Least permeable, found in tissues that need tight control over what passes through Fenestrated capillaries: Moderately permeable, allowing larger molecules to pass, found in * organs involved in filtration and absorption Sinusoidal capillaries: Highly permeable, allowing large particles like proteins and cells to pass, found in specialized organs Types of capillaries Continuous – vast majority of capillaries ▪ Intercellular junctions allow movement of water-soluble substances Fenestrated – for areas that require higher filtration or increased delivery of soluble substances ▪ Glomerulus in the kidney ▪ Endocrine glands, lamina propria of the intestines ▪ Fenestrae are covered/altered by proteins that form a sort of diaphragm whose properties can be altered Types of capillaries Sinusoidal ▪ Discontinuous basal lamina ▪ Larger diameter ▪ Massive gaps between the intercellular junctions ▪ Specialized for allowing cells to move in and out of them ▪ Locations: liver, spleen, lymph nodes bone marrow Capillary Overview Overview of Veins Large Veins Examples: Vena cava, pulmonary veins, portal vein. Structure: Tunica Intima: Well-developed, with endothelium and a prominent subendothelial layer. Tunica Media: Thin, containing relatively fewer smooth muscle cells compared to arteries. Tunica Adventitia: Thickest layer, composed of dense connective tissue, collagen, and elastic fibers. Contains vasa vasorum (small blood vessels) to nourish the vein wall. Function: Large veins return deoxygenated blood to the heart from systemic circulation. They are able to accommodate large blood volumes. Overview of Veins Medium Veins Examples: Femoral vein, renal vein, brachial vein. Structure: Tunica Intima: Thin, with endothelium and a thin subendothelial layer. Tunica Media: Thinner than arteries, with scattered smooth muscle cells. Tunica Adventitia: The thickest layer, with collagen and elastic fibers. May have some vasa vasorum. Valves: Present, especially in the limbs, to prevent backflow of blood due to low pressure. Function: These veins drain blood from organs and limbs, using valves to direct blood flow toward the heart. Overview of Veins Small Veins (Venules) Examples: Postcapillary venules, collecting venules. Structure: Tunica Intima: Endothelial cells with a thin basal lamina. Tunica Media: Very few layers of smooth muscle cells or absent in the smallest venules. Tunica Adventitia: Thin layer of connective tissue. Function: Venules collect blood from capillaries and begin the process of returning it to larger veins. Postcapillary venules also play a role in inflammatory responses, allowing white blood cells to exit the bloodstream and enter tissues. Overview of Veins Overview of Veins Many large and medium veins have valves that are formed from reflections of the tunica intima ▪ More notable in the lower extremities than upper Lumen of veins is much larger and much less likely to be constricted than that of an artery ▪ Therefore systemic veins contain close to 2/3 of the body’s blood volume ▪ Large and medium veins can constrict somewhat in response to increased secretion of catecholamines Properties of Large Vessels Hemodynamics = study of how blood moves in the vascular compartments ▪ Impacted by properties of the vessels, properties of the blood, activity of the heart, and the presence of gravity (and position of the patient) ▪ How do you think we get blood back up to the heart from the legs when standing? Calf muscles = second heart! Properties of Large Vessels Review: Poiseuille’s Law ▪ Describes the flow of an incompressible and Newtonian fluid (like blood in small vessels) through a cylindrical pipe or tube. ▪ It relates fluid flow rate to the vessel's dimensions and pressure differences. ▪ Small Radius = Higher Resistance: According to the law, smaller vessels have higher resistance. This is crucial in regulating blood flow, especially in smaller The expression arteries and arterioles. above could be ▪ Vessel Length and Viscosity: Increased length and viscosity also increase considered resistance. For instance, conditions like conductance to dehydration can increase blood viscosity, flow… the inverse leading to higher resistance and decreased flow. would be resistance Properties of Large Vessels Review: Poiseuille’s Law ▪ Q = flow ▪ (P1 – P2) = pressure gradient ▪ r = radius of the vessel ▪ n = viscosity ▪ l = length of the vessel Which parameters will increase flow The expression as they increase? Decrease flow? above could be Flow increases with increased considered pressure and vessel radius. conductance to Flow decreases with increased viscosity and vessel length. flow… the inverse would be resistance Properties of Large Vessels Poiseuille’s law only “works” if flow is not turbulent within the arteries – i.e., flow is laminar Laminar flow - where fluid moves in parallel layers, and each layer flows smoothly without mixing with adjacent layers. Properties of Large Vessels If flow becomes turbulent, then flow is proportional to the square root of the pressure gradient ▪ turbulent flow is less “energy efficient” ▪ In turbulent flow, fluid movement is irregular, with eddies, vortices, and chaotic mixing between layers. ▪ This typically occurs at high flow velocities or in areas with sharp changes in vessel diameter (e.g., bifurcations, atherosclerotic plaques). ▪ In turbulent flow, Poiseuille’s law does not apply because the law assumes a constant resistance to flow. However, in turbulent flow, the resistance increases unpredictably due to chaotic fluid motion and energy dissipation. Properties of Large Vessels The Reynold’s number predicts when turbulent flow will occur ▪ When it is > 2,300, flow will be turbulent Reynold’s number calculation circled below ▪ p = fluid density, D = diameter of the vessel, v = velocity of the fluid ▪ n = viscosity What are pathological situations that could increase the likelihood Re ( (widensity mer) = of turbulent flow? Properties of Large Vessels What are pathological situations that could increase the likelihood of turbulent flow? · 3 Atherosclerosis Stenosis Hypertension Aneurysms Valvular heart disease, All create conditions that disturb the smooth, laminar flow of blood, increasing the likelihood of turbulence. Turbulent flow is more likely when blood velocity increases, vessel diameter is irregular or constricted, or viscosity decreases. Properties of Large Vessels Bernoulli Principle: The concept that pressure is constant in a system, regardless of velocity If forward velocity increases in a blood vessel, that increases “forward pressure” ▪ What is the impact on the force that is exerted against the wall of the vessel? Hemodynamic Characteristics of Different Vessels Look carefully at this diagram: Can you explain the changes in pressure and velocity in the ▪ arteries? ▪ capillaries? ▪ veins? Hemodynamic Characteristics of Different Vessels Summary of Changes: From Arteries to Capillaries: Pressure: Decreases significantly. Velocity: Decreases significantly (slowest in capillaries). From Capillaries to Veins: Pressure: Continues to decrease but at a slower rate. Velocity: Increases as blood moves back toward the heart. Hemodynamic Characteristics of Different Vessels Arteries: Pressure: Blood pressure is highest in the aorta and large arteries (around 120 mm Hg during systole). As blood travels through smaller arteries, the pressure gradually decreases but remains relatively high compared to other vessels. Velocity: Blood velocity is high in the aorta due to the forceful contraction of the heart. As blood moves into smaller arteries and arterioles, the velocity starts to decrease slightly due to the increased cross-sectional area. Capillaries: Pressure: Pressure drops significantly in the capillaries, usually around 30 mm Hg or lower. This reduction in pressure is crucial for facilitating the exchange of nutrients and waste between the blood and surrounding tissues. Velocity: Blood velocity is lowest in the capillaries. This is essential because slower flow allows more time for the exchange processes to occur. The vast network of capillaries (huge total cross-sectional area) contributes to this reduction in velocity. Hemodynamic Characteristics of Different Vessels Veins: Pressure: After passing through the capillaries, pressure continues to drop as blood enters the venules and larger veins, eventually reaching around 5-10 mm Hg near the vena cava. The pressure in veins is significantly lower than in arteries. Velocity: Blood velocity begins to increase as it returns to the heart through the venules and larger veins, even though the pressure is low. The cumulative effect of the return flow through the veins results in a higher velocity as it approaches the heart, despite the low pressure. Vascular Systems in Series: Series Circulation: In a series arrangement, blood flows through one vessel, then directly into the next, one after the other. This is less common in the body. Example: The arrangement of blood flow from the heart through the aorta and then through various arteries to a single capillary bed is somewhat sequential but isn't truly series in a broad physiological sense. Implication: In a series circuit, the total resistance to blood flow is the sum of the resistances of each individual vessel. This can lead to higher overall resistance and potentially less efficient blood flow. Vascular Systems in Parallel: * Parallel Circulation: In a parallel arrangement, blood can flow through multiple vessels simultaneously. This is the predominant arrangement in the body. Example: The systemic circulation is characterized by many capillary beds in parallel. For instance, when blood reaches an organ, it can be distributed through multiple arterioles leading into many capillaries, allowing different tissues to receive blood concurrently. Implication: In a parallel circuit, the total resistance is less than the resistance of any individual vessel. This is because each additional pathway provides an alternative route for blood flow, which reduces the overall resistance and enhances perfusion. This allows for efficient distribution of blood to various tissues and organs Series vs. Parallel Vascular Circuits There are very few vascular systems in the body that are in series – most are in parallel ▪ Using the diagram below, can you describe why the cumulative resistance of the systemic capillary beds are so low, even though the radius of the vessels are so small (Poiseuille’s law says small vessel = higher resistance)? It is because the blood is being evenly distributed through multiple vessels, reducing resistance Series vs. Parallel Vascular Circuits Despite individual capillaries having high resistance due to their small radius their parallel arrangement results in low cumulative resistance, enabling effective blood flow and nutrient exchange in the systemic circulation. Central vs. Peripheral Blood Volume Total blood volume in an “average-sized” adult male is ~ 5 L ▪ 80% in the systemic circulation * ▪ 60% in systemic veins ▪ Small arteries and capillaries – 20% of blood volume 3 Veins are very compliant – they’re “floppy” and are easy to distend (up to a point) ▪ If you give someone 500 mL of normal saline, 80% of that stays in the systemic circulation, and most of it (95%) locates in the veins… Thus having a modest effect on arterial blood pressure Compliance Compliance describes how much pressure is required to change the diameter (volume) of a structure… in this case a blood vessel the ability of a blood vessel to expand and accommodate changes in blood volume or pressure. High compliance: small amount of pressure ! large change in volume ▪ In general, veins are more compliant than arteries ▪ Compliance of a vessel – especially larger arteries - decreases with age; vessels become “stiffer” Atherosclerosis Calcification E Compliance decreases with contraction of smooth muscle within blood vessels Compliance Arterial Compliance Definition: Arterial compliance is the ability of arteries to stretch and expand when blood pressure increases during systole (heart contraction). Characteristics: Low Compliance: Arteries have relatively low compliance, meaning they do not stretch easily. This is due to their thick muscular walls and elastic fibers, which allow them to withstand high pressure from the blood being pumped by the heart. Function: The low compliance helps maintain high blood pressure, ensuring efficient blood flow to various organs. Arteries can also dampen the pressure fluctuations caused by the heartbeat. Compliance Venous Compliance Definition: Venous compliance is the ability of veins to expand and hold more blood without a significant increase in pressure. Characteristics: High Compliance: Veins have high compliance, allowing them to accommodate larger volumes of blood at lower pressures. Their walls are thinner and less muscular compared to arteries. Function: This high compliance serves as a blood reservoir. During periods of rest or when blood volume increases, veins can expand to store extra blood without a drastic increase in pressure, which helps regulate blood flow and maintain venous return to the heart. Central vs. Peripheral Blood Volume Central blood volume (25%) includes: ▪ vena cavae ▪ heart ▪ pulmonary circulation The central blood volume is important for: ▪ determining preload of the heart (optimal ventricular stretch ! optimal force of contraction) ▪ pathologic situations like pulmonary edema due to congestion We can acutely shift large volumes of blood into or out of the central compartments by varying the amount in the peripheral compartments ▪ Peripheral compartments – veins in the abdominal cavity or limbs Central vs. Peripheral Blood Volume Using this knowledge: ▪ What happens when you go from lying down to standing? Causes blood to shift from central to peripheral du to gravity - decreases cardiac output and BP temporarily ▪ Why is it such a big deal to give someone with CHF an extra litre of IV fluid? CHF causes fluid overload in the body, which can be worsened by extra IV fluid ▪ Why is it advantageous to constrict our arteries and veins when confronted with a serious hemorrhage? Reduces blood loss, maintains BP, prioritize blood flow to vital organs Hemodynamic Physiology Compliant, yet elastic arteries decrease cardiac work ▪ Elasticity = the tendency for a structure to resume it’s original shape once a distending force (pressure) is removed ▪ If the heart was beating into a rigid pipe, no distention would occur and no significant potential energy – elasticity that can be converted to pressure - could be stored in the vessel wall Therefore blood flow would decrease to close to zero during diastole ▪ Every single systole would have to “charge up the pressure” and apply significant kinetic energy to the column of blood Requires more energy usage Hemodynamic Physiology Increasing venous filling pressure increases cardiac output… ▪ due to improved actin-myosin overlap at optimal preload … but at the same time, as cardiac output increases, the central venous blood volume is removed more quickly ▪ due to increased movement of fluid Hemodynami c Physiology The upright posture poses a number of challenges to venous return to the central blood volume ▪ Pressures can be as high as 90 mm Hg in the feet, but 40 mm Hg at the thigh Solution: ▪ Valves in the leg veins of the lower body ▪ Surround the leg veins with skeletal muscle ▪ Breathing in decreases intrathoracic pressure… Which draws blood upwards Mean Arterial Pressure What is the “average” pressure in the arterial system? ▪ Can we average systolic and diastolic pressures together to form a mean arterial pressure (MAP)? ▪ Since 1/3 of the cardiac cycle is spent in systole, then it stands to reason that the average pressure is the diastolic pressure + 1/3 (systolic pressure – diastolic pressure) ▪ Multiple different ways of writing this equation – “easiest” is below Pulse pressure (PP) = systolic pressure (SP) – diastolic pressure (DP) MAP = DP + PP/3 OR MAP = Diastolic Pressure + 1/3(Systolic Pressure - Diastolic Pressure) For many, that’s 90 + 13 = 103 mm Hg The Microcirculation Artery Entering an Organ Branching of Arteries: When an artery enters an organ, it undergoes multiple branches (six to eight times). These arteries are known as distributing arteries, as they distribute blood to different regions of the organ. Arterioles Further Branching: The distributing arteries branch into arterioles, which are smaller blood vessels with a diameter of about 10 to 15 microns. Function: Arterioles typically branch two to five times and serve as the primary site for regulating blood flow into the capillaries. They play a key role in controlling resistance and blood pressure. Metarterioles Formation from Arterioles: As arterioles continue to branch, they lead to metarterioles, which are transitional vessels between arterioles and capillaries. Muscle Layer: Muscular arterioles (the larger arterioles) usually have a single layer of smooth muscle that helps regulate blood flow. Discontinuous Muscle: Metarterioles have a discontinuous layer of smooth muscle along their length, which is not uniform like in the larger arterioles. The Microcirculation Capillaries Structure: Metarterioles lead directly to capillaries, which have an internal diameter ranging from 3 to 9 microns. Function: Capillaries are the sites of nutrient and gas exchange between the blood and surrounding tissues. Their small diameter allows red blood cells to pass through in single file, facilitating this exchange. Pre-Capillary Sphincters Role: At the junction of metarterioles and capillaries are pre-capillary sphincters. These are small bands of smooth muscle that regulate blood flow into the capillary beds. Function: When the sphincters are contracted, blood flow into the capillaries is reduced or halted, directing blood flow to other areas or minimizing flow when tissues are not active. When relaxed, they allow more blood to flow into the capillaries for exchange. Basement Membrane of Capillaries Composition: The basement membrane of capillaries is primarily formed by Type IV collagen. This structure supports the capillary walls and provides a scaffold for endothelial cells, ensuring the integrity and functionality of the capillaries. The Microcirculation Continuous Capillaries Overview Structure and Function: Continuous capillaries are characterized by an uninterrupted endothelial lining, which allows them to maintain a relatively tight barrier between the blood and surrounding tissues. Most continuous capillaries are considered “leaky,” permitting the free movement of small, water-soluble substances (like ions, glucose, and amino acids) while restricting larger molecules and cells. Notable Exceptions 13 Blood-Brain Barrier (BBB) Blood-Testes Barrier The Microcirculation Tight Junctions Composition: In these exceptions, the capillaries have tight junctions formed by proteins called occludins and claudins. These proteins connect adjacent endothelial cells and form a more complete seal compared to regular continuous capillaries. Function of Tight Junctions: The tight junctions in these specialized capillaries significantly reduce paracellular permeability (the movement of substances between cells), ensuring that only specific substances can cross the endothelial barrier. This sealing mechanism is crucial for protecting sensitive environments, like the brain and testes The Microcirculation Pinocytosis Process: Continuous capillaries engage in a process known as pinocytosis, where they continuously endocytose (internalize) small pockets of extracellular fluid from the vascular space. This involves the formation of small vesicles that capture fluid and any dissolved substances. Transport Across the Basement Membrane: Once internalized, these vesicles transport the fluid and its contents across the endothelial cells and release them into the surrounding tissue. This mechanism allows for the regulated exchange of substances between the bloodstream and the tissue, even in areas where tight junctions restrict other forms of transport. The Microcirculation Effect of Inflammation Increased Pinocytosis: During inflammation, the rate of pinocytosis in continuous capillaries is increased. This enhanced fluid uptake can help facilitate the transport of immune cells and substances necessary for the inflammatory response, such as antibodies and nutrients, to the affected area. Implication of Increased Permeability: Inflammatory mediators can also lead to changes in the permeability of these capillaries, allowing larger molecules and immune cells to pass through more readily. This is part of the body’s response to injury or infection, as it helps bring more immune cells to the site of inflammation. Continuous capillaries Caveolae – “small caves” ▪ endocytosis and 43 transcytosis of macromolecules across endothelial cells ▪ vesicles can move slowly through the endothelial cell Serve as important structures in endothelial cells that facilitate the endocytosis and transcytosis of macromolecules. They allow for controlled transport across the endothelial barrier while intercellular clefts provide a selective pathway for small molecules. Continuous capillaries Pinocytic vesicles can coalesce to form vesicular channels - Intercellular clefts ▪ just small enough so albumin doesn’t get through Movement of substances across capillaries Substances move down their concentration gradient (diffusion) except for water ▪ Water moves down its concentration gradient AND down its hydrostatic gradient ▪ Where the equilibrium lies can be calculated using the Starling equation – REVIEW from BMS 100, see next slide The speed with which substances diffuse down their gradient is determined by Fick’s law ▪ REVIEW as well – slide after next Starling forces - simplified **Starling forces govern fluid exchange in the body by balancing the hydrostatic pressure that pushes fluid out of capillaries and the oncotic pressure that pulls fluid back in.** Variables: 𝐋𝐩 = the “leakiness” of the capillary wall to water ▪ The “inverse” of resistance 𝐏 = hydrostatic pressure 𝛑 = osmotic pressure “cap” = the fluid within the capillary “ISF” = the fluid within the interstitial space 𝛔 = how much protein leaks through the capillary wall 𝐅𝐥𝐮𝐱 = 𝐋𝐩[ (𝐏𝐜𝐚𝐩 − 𝐏𝐈𝐒𝐅 ) − 𝛔(𝛑𝐜𝐚𝐩 − 𝛑𝐈𝐒𝐅)] Fick’s law - defined describes the diffusion of substances, particularly the movement of gases or solutes across a barrier, such as cell membranes or capillary walls. F = flow/flux ▪ number of molecules of a substance diffusing from point A to point B over time (𝐶𝐴 − 𝐶𝐵 ) = concentration gradient ▪ Difference in concentration on either side of the membrane 𝑨(𝑪𝑨 − 𝑪𝑩 ) A = surface area of the membrane 𝑭=𝒌 ∙ 𝒌 = a constant that increases 𝒕 when: ▪ The substance is a smaller molecule that dissolves better in the barrier ▪ The permeability of the barrier to the substance increases t = thickness of the membrane Movement of substances across capillaries Movement of substances across capillary walls is necessary for life ▪ However, too much results in edema Factors that keep water in the capillary: ▪ albumin and other plasma proteins a small amount leaks through ! a small oncotic pressure in the interstitial space Factors that reduce excessive edema in tissue ▪ glycosaminoglycans that absorb water like a “sponge” (lots of CHO moieties) ▪ lymphatics in most tissue We’ll discuss edema more next day Regulation of blood flow at the level of the capillary Autoregulation – refers to the intrinsic ability of a capillary bed to regulate its own blood flow based on local tissue factors, rather than relying on systemic factors like overall blood pressure. This ensures that tissues receive an adequate blood supply that meets their metabolic needs, even when systemic blood pressure varies. Myogenic regulation ▪ Maintains a relatively constant rate of tissue flow despite changes in MAP Higher pressures in the vessel (smaller vessels, i.e. arterioles) ! smooth muscle stretching → increased constriction (stretch-activated calcium channels) Decreased pressure ! decreased stretch → dilation ▪ Likely has very little physiologic significance by itself – metabolic autoregulation seems to be much more important However, some hormonal signals work by changing the sensitivity of myogenic constriction/dilation Autoregulation at work The safe range for blood flow is about 80% to 125% of normal usually occurs at arterial pressures of 60 to 160 mm Hg At pressures above about 160 mm Hg, vascular resistance decreases because the pressure forces dilation to occur at pressures below 60 mm Hg, the vessels are fully dilated, and resistance cannot be appreciably decreased further. Regulation of blood flow at the level of the capillary Autoregulation – metabolic factors ▪ O2, CO2, H+, lactate, adenosine, K+ accumulate and lead to vasodilation Can you explain why these substances change their local tissue concentrations in metabolically active tissue? Important exception – pulmonary microcirculation – see later slides ▪ increased blood flow leads to washout of the same metabolites ▪ Can lead to reactive hyperemia – after a period of vasoconstriction, metabolites build up and lead to a period of vasodilation and greatly increased perfusion ▪ Interestingly, mitochondria will produce H2S in hypoxic situations – may be the major metabolic autoregulatory mediator in some tissues Metabolic effects on arterioles Hormonal factors in regulating tissue flow Vasodilation and nitric oxide NO is produced by endothelial cells - shear stress causes its release from the endothelium ▪ Shear stress = the force placed on a blood vessel along the axis of blood flow (increases with pressure, velocity of flow, turbulence) ▪ Detected by mechanoreceptors that modulate nitric oxide synthase (which produces NO from L-arginine) NO diffuses to nearby vascular smooth muscle ▪ NO activates soluble guanylyl cyclase ! cGMP production ! activation of PK G ! relaxation (through dephosphorylation of myosin) of smooth muscle ** Net result – shear stress increases ! nitric oxide production ! vasodilation in arterioles ! a decrease in shear stress Nitric oxide mechanism Hormonal Controls Vasodilation Histamine: vasodilates arterioles, constricts venules → edema Bradykinin: circulating protein, activated by inflammatory signals ▪ Potent vasodilator Prostaglandin E2 and I2 Epinephrine, norepinephrine through beta-2 receptors Hormonal Controls Vasoconstriction Epinephrine, norepinephrine through alpha-1 receptors Serotonin – released in tissue damage → local vasoconstriction ▪ When released by platelets or GI tract, sometimes results in vasodilation Thromboxane A2 and Prostaglandin F constrict Angiotensin II ADH (anti-diuretic hormone) Reaction to damage (platelet plug formation) Hormonal Control - SNS Catecholamines Epinephrine has a much greater effect than norepinephrine on β2 receptors, and they are - roughly equal for α1, α2, and β1 In general, β2 causes vasodilation in skeletal muscle, cardiac muscle and liver, and and α receptors cause vasoconstriction in all tissues including muscle Comparison of Tissue Vascular Beds Skeletal Muscle Compression of Capillaries: During muscle contractions, the skeletal muscle fibers shorten and exert pressure on the capillaries that supply blood to the muscle. This compression can temporarily restrict blood flow within the capillary bed during contraction. Emptying the Venous Tree: When skeletal muscles contract, they help pump blood back to the heart by compressing veins. This is particularly effective in emptying the venous tree, which can increase the pressure gradient driving blood flow from arteries to veins. Increased Blood Flow During Exercise Increased Flow Through Capillary Beds: After the muscle contractions stop, there is a rapid increase in blood flow to the capillary beds. This increased flow delivers oxygen and nutrients necessary for recovery and continued activity. Vasomotor Control: Alpha-1 (α1) Receptors: These receptors are typically associated with vasoconstriction of vascular smooth muscle (VSM). Activation of α1 receptors in skeletal muscle arterioles can reduce blood flow. Beta-2 (β2) Receptors: In contrast, β2 receptors mediate vasodilation in response to certain stimuli, such as epinephrine (adrenaline) during physical activity, leading to increased blood flow. Comparison of Tissue Vascular Beds Metabolic Factors Overriding Autonomic Nervous System (ANS) Influence Lactate, Potassium (K+), Adenosine: During intense exercise or muscle activity, metabolic byproducts accumulate: Lactate: Produced during anaerobic metabolism, signaling the need for increased blood flow and oxygen delivery. Potassium (K+): Released by muscle cells during activity, causing local vasodilation to increase blood flow. Adenosine: Released as a result of ATP breakdown; acts as a potent vasodilator. * E 35 These metabolites override the vasoconstrictive effects mediated by the ANS, leading to vasodilation and enhanced blood flow to active skeletal muscles. Comparison of Tissue Vascular Beds Rest vs. Exercise: Oxygen Extraction and Blood Flow Resting State: At rest, skeletal muscles extract approximately 25-30% of the available oxygen (O2) from the blood flowing through the capillary bed. Blood flow during rest is relatively low, which matches the lower metabolic demands of the muscle at this time. Exercising State: During exercise, blood flow to skeletal muscles can increase up to 20-fold. This dramatic increase is facilitated by vasodilation and recruitment of additional capillaries. The number of perfused capillaries can increase 3 to 4 times, allowing for greater surface area for gas exchange and nutrient delivery. Oxygen extraction can increase to as much as 90% during maximal exercise, meeting the heightened metabolic demands of the working muscle. Comparison of Tissue Vascular Beds Cerebral pH and adenosine regulate neural blood flow ▪ mostly mediated by local increases in H+/adenosine or by carbon dioxide diffusing across the BBB ▪ Other vasomediators blocked by BBB, so it doesn’t really respond to circulating vasodilatory/constricting influences Brain is also capable of modulating ANS activity, thus it can control blood flow to other tissues ▪ wide range of mechanisms, including baroreceptor input, CO2 concentrations, endocrine feedback loops ↑ intracranial pressure → ↓ perfusion ▪ in response to this, the brain increases mean arterial pressure (Cushing reflex) Comparison of Tissue Vascular Beds Pulmonary Controlled by O2 concentrations ▪ constriction in response to decreased oxygen levels ▪ how does this compare to other vascular beds? Why does this make sense? Skin Sympathetic is most important - alpha1 receptors constrict important for regulating body temperature Comparison of Tissue Vascular Beds Coronary Most sensitive to O2 and adenosine Mechanical compression during systole → poorer blood flow ▪ Can you describe two reasons why the heart is in big trouble if diastole shortens too much? Renal xB of blood being pumped Myogenic and tubuloglomerular feedback also controlled by a variety of hormones (catecholamines, angiotensin II) Simulating hemorrhage You can simulate hemorrhage by placing a patient in a chamber that applies negative pressure to the lower body ▪ Why is this system a smart experimental system? ▪ What would be the impact on the cardiac system once the vacuum is turned on? https://www.sfu.ca/aerospacelab/research-areas/ orthostatic-intolerance/lower-body-negative- pressure.html Simulating hemorrhage Definition: Lower Body Negative Pressure (LBNP) simulates hemorrhage by redistributing blood into the lower extremities. Key Concept: Mimics central hypovolemia without physical blood loss. Why is LBNP a Smart Experimental System? Controlled: Precise pressure adjustments mimic varying hemorrhage levels. Non-invasive: No actual blood loss, safe and reversible. Physiological Realism: Accurately simulates cardiovascular effects of hemorrhage. Real-time Monitoring: Continuous tracking of heart rate, blood pressure, and more. Versatile Applications: Study hemorrhage, orthostatic intolerance, shock, syncope. Simulating hemorrhage Cardiovascular Impact of LBNP Decreased Venous Return: Blood pools in lower extremities, reducing preload. Reduced Stroke Volume & Cardiac Output: Less blood fills the heart, decreasing output. Compensatory Tachycardia: Heart rate increases to maintain blood pressure. Peripheral Vasoconstriction: Sympathetic response causes vessel constriction to maintain pressure. Hypotension Risk: Prolonged LBNP can lead to a dangerous drop in blood pressure.