Blood Flow Regulation-Notes.pdf
Document Details
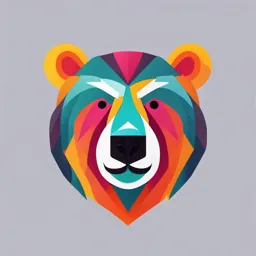
Uploaded by FruitfulIntegral
Wayne State University
Tags
Full Transcript
Donal S. O'Leary, Ph.D. Cardiovascular Physiology BLOOD FLOW REGULATION Learning Objectives: 1. Understand the concepts of local control including: reactive hyperemia active hyperemia autoregulation mechanical (tissue pressure) effects. 2....
Donal S. O'Leary, Ph.D. Cardiovascular Physiology BLOOD FLOW REGULATION Learning Objectives: 1. Understand the concepts of local control including: reactive hyperemia active hyperemia autoregulation mechanical (tissue pressure) effects. 2. Understand the concepts of remote control, effect of activation of alpha, beta, V1, and AII receptors. Lecture Outline: 1. Active vs. reactive hyperemia 2. Autoregulation a. Myogenic hypothesis b. Metabolic hypothesis 3. Mechanical effects (Tissue Pressure) 4. Remote Control of the Circulation a. Autonomic Nervous system b. Circulating hormones 5. Local – Remote Interaction 1 Donal S. O'Leary, Ph.D. Cardiovascular Physiology Regulation of blood flow can be divided into two general areas, local control and remote control. LOCAL CONTROL: Control of blood flow by factors intrinsic to that tissue or organ. ENDOTHELIUM: Initially thought to be simply structural in nature, now it is known that the endothelium is actively involved in the regulation of vascular smooth muscle activity by the release of various factors which can cause both vasodilation and vasoconstriction. Nitric Oxide: Under basal conditions, the endothelial cells are constantly producing Nitric Oxide (NO). NO is a gas formed via activation of the enzyme Nitric Oxide Synthase (NOS) which converts L-Arginine to L- Citrulline + NO. NO then diffuses out of the endothelium cell and enters the vascular smooth muscle where it stimulates soluble guanylate cyclase with subsequent formation of cyclic-GMP. cGMP activates protein kinase G which elicits vasodilation by lowering free Ca2+ levels as well as activating K+ channels causing hyperpolarization. cGMP is broken down to inert GMP by cGMP-dependent phosphodiesterase which then terminates the effects of NO. Under normal, basal conditions in blood vessels, NO is continually being produced by cNOS and is calcium dependent. Two pathways exist to stimulate cNOS, both are activated by release of calcium ions. 2 Donal S. O'Leary, Ph.D. Cardiovascular Physiology 1: Blood flow shearing forces causes the release of calcium and subsequent cNOS activation, thus NO production and vasodilation – termed flow-dependent vasodilation. 2: Endothelial receptors for a variety of substances stimulate calcium release and subsequent NO production termed receptor-stimulated NO formation. Included are receptors for acetylcholine (muscarinic), bradykinin, substance-P, adenosine amongst many other vasoactive substances. The level of tonic release of NO was revealed by the observation that MAP rises about 20mmHg when cNOS is inhibited in experimental animals. A second isoform of endothelial NOS is iNOS which is calcium independent and the tonic activity is very low. However once stimulated during inflammation by bacterial endotoxins (e.g., lipopolysaccharide) and cytokines such as tumor necrosis factor (TNF) and interleukins the amount of NO released much greater than that produced by cNOS. There is a third type of NOS, neuronal NOS (nNOS) which exists in the central nervous system in areas which use NO as a neurotransmitter/neuromodulator and in specialized peripheral nerves controlling vasodilation in select vascular beds. There are many disease states wherein both the tonic ability to produce NO as well the ability to stimulate the release of NO can be markedly impaired including, heart failure, hypertension, diabetes, elevated cholesterol and triglyceride levels. Drugs have been developed which are cGMP-dependent phosphodiesterase antagonists. These prolong and potentiate the effects of released NO by blocking the breakdown of cGMP. These include Viagra as penile erection is caused by stimulation of parasympathetic nerves to the penis, releasing acetylcholine and activating NO formation thereby causing vasodilation. Endothelin (ET-1) is a 21 amino acid peptide that is produced by the vascular endothelium from a 39 amino acid precursor, big ET-1, through the actions of an endothelin converting enzyme (ECE) found on the endothelial cell membrane. ET-1 formation and release are stimulated by angiotensin II (AII), antidiuretic hormone (ADH), thrombin, cytokines, reactive oxygen species, and shearing forces acting on the vascular endothelium. ET-1 release is inhibited by prostacyclin and atrial natriuretic peptide as well as by nitric oxide. Released ET binds to receptors on the vascular smooth muscle and causes 3 Donal S. O'Leary, Ph.D. Cardiovascular Physiology increased calcium release from the sarcoplasmic reticulum which promotes smooth muscle contraction. Endothelin is one of the most potent vasoconstrictors known. Reactive Hyperemia = ↑ blood flow to an organ after a period of occlusion Tissue hypoxia Build-up of vasoactive metabolites Figures 7-4 from Klabunde’s Cardiovascular Physiology Concepts 2nd Ed REACTIVE HYPEREMIA refers to the rise in blood flow above normal levels after release of an occlusion of flow. The extent and duration of the reactive hyperemia is dependent on the duration of the occlusion of blood flow. With normal metabolism, there is a continuous production of of metabolic vasodilators (H+, lactate, K+, CO2, adenosine, prostaglandins, etc.). Even at low metabolic rates, there exists a tonic level of metabolite production. The metabolites are being produced, released into the interstitium and either metabolized by the cells or carried away in the blood. Thus, with reactive hyperemia, when blood flow is arrested, the local concentration of metabolites increases due to the lack of wash out by the normal level of blood flow. With the increased accumulation of metabolites, vasodilation of the arterioles occurs and when the occlusion is removed, blood flow increases markedly due to the low resistance. The increase in blood flow washes away the metabolites and blood flow returns to normal levels. ROLE OF NITRIC OXIDE With the sudden large rise in blood flow, there is an increase in shear stress on the endothelium which is a stimulus for NO production and release. Nitric Oxide blockade lowers basal levels of blood flow and reduces the peak and especially the duration of the reactive hyperemia mediated vasodilation. 4 Donal S. O'Leary, Ph.D. Cardiovascular Physiology ACTIVE HYPEREMIA When metabolic rate increases in an organ, blood flow to that organ also increases - termed Active Hyperemia. The extent of the increase in blood flow is dependent on the magnitude of the increase in metabolic rate. Active Hyperemia Blood flow to an organ is proportional to its metabolic activity Tissue metabolism Production of vasoactive metabolites Figures 7-5 from Klabunde’s Cardiovascular Physiology Concepts 2nd Ed The mechanisms mediating Active Hyperemia are similar to those mediating Reactive Hyperemia. With increased metabolic rate, there is an increased production of metabolic vasodilators including H+, lactate, K+, CO2, adenosine, and prostaglandins. These cause vasodilation of the arterioles in this tissue in proportion to the increase in metabolic rate. 5 Donal S. O'Leary, Ph.D. Cardiovascular Physiology Flow Mediated Vasodilation via release of NO from the endothelium also plays a role as the rise in blood flow increases shear stress on the endothelium which stimulates the release of NO. Virtually all vascular beds will display Active Hyperemia, most notably this is the primary mechanism by which vasodilation occurs in SKELETAL MUSCLE and CARDIAC TISSUE in response to exercise and in the INTESTINES after consumption of a meal. AUTOREGULATION - The ability of an organ or tissue to maintain a relatively constant blood flow despite changes in perfusion pressure. When a sustained increase in perfusion pressure occurs, blood flow initially increases (remember P = F x R, F = P/R, therefore if P increases at constant R, then F increases [P - perfusion pressure, F - flow, R - resistance]). However, over time blood flow decreases back toward the initial value despite a maintained increased perfusion pressure. Panel B shows the relationship between the steady-state blood flow and the arterial pressure (perfusion pressure). There exists a range of arterial pressures over which autoregulation is quite effective (AUTOREGULATORY RANGE). Outside of this range autoregulation is less effective or non-existent and substantial changes in blood flow occur with changes in perfusion pressure. Not all vascular beds display marked autoregulation, however the kidney and brain are two areas in which effective autoregulation has been demonstrated. Two major hypotheses have been proposed to explain autoregulation. METABOLIC HYPOTHESIS: - increased pressure causes an initial increase in blood flow. The increased blood flow "washes out" the metabolic vasodilators and the concentration of these substances decreases. With a decreased concentration of metabolic vasodilators, some vasoconstriction occurs, increasing resistance and decreasing blood flow back towards normal. Opposite responses occur with a decrease in perfusion pressure (transient decrease in flow, buildup of metabolites, vasodilation returning flow back towards normal). MYOGENIC HYPOTHESIS: - based on the observation that when vascular smooth muscle is stretched, it contracts. When the tension on vascular smooth muscle is reduced, it relaxes. Thus, with an increase in perfusion pressure, tension on the smooth muscle increases. In response to the increased tension, constriction of the smooth muscle occurs which increases vascular resistance and thus blood flow decreases towards normal levels. Opposite responses occur with a decrease in perfusion pressure. 6 Donal S. O'Leary, Ph.D. Cardiovascular Physiology Autoregulation of Blood Flow Autoregulation is the intrinsic ability of an organ to maintain a constant blood flow despite changes in perfusion pressure. Autoregulation Figure 7-3 from Klabunde’s Cardiovascular Physiology Concepts 2nd Ed 7 Donal S. O'Leary, Ph.D. Cardiovascular Physiology MECHANICAL EFFECTS (TISSUE PRESSURE) Perfusion Pressure (PP) is the energy gradient for driving blood flow and is created by the pumping of the heart. PP is calculated as the DIFFERENCE in pressure between two points. For the entire body, PP = MAP – CVP. Coronary Blood Flow Cycle Figure 24-4 from Boron and Boulpaep’s Medical Physiology 2n For a given vascular bed PP is normally MAP-Venous Pressure. So, for the kidney, perfusion pressure = MAP – Renal Venous Pressure. However, when tissue pressure (TP) exceeds venous pressure then perfusion pressure = MAP – TP. In areas such as skeletal and cardiac muscle substantial changes in tissue pressure can occur which can markedly affect blood flow. NOTE that during systole blood flow in the left coronary artery (perfusion the left ventricle) initially falls to zero despite an increase in arterial pressure. This can be explained by the large increase in tissue pressure within the ventricle during each contraction. This mechanical force compresses the blood vessels and thus decreases the effective perfusion pressure. 8 Donal S. O'Leary, Ph.D. Cardiovascular Physiology Tissue Pressure in skeletal muscle during rhythmic contractions acts as a pump forcing venous blood back toward the heart. This is one of the mechanisms allowing cardiac output to rise during exercise as this aids in filling of the ventricle during the shortened filling time at high heart rate with strenuous workloads. With strong static contractions tissue pressure can rise so high that arterial inflow is blocked (similar to systole in the left heart). Thus, skeletal blood flow is reduced and the ability to maintain the strong contraction is limited by the ischemia. A similar effect of Tissue Pressure can occur when edema or hemorrhage occur in encapsulated areas (kidney, cranium). Tissue pressure will increase thus decreasing effective perfusion pressure and blood flow will fall. 9 Donal S. O'Leary, Ph.D. Cardiovascular Physiology REMOTE CONTROL - control by factors extrinsic to the tissue or organ studied. NEURAL CONTROL - control by the autonomic nervous system, primarily the sympathetic nervous system. Only the blood vessels in the heart, genitals and brain are innervated by the parasympathetic nervous system. The sympathetic post-ganglionic neurons use norepinephrine (NE) as the primary neurotransmitter (however several other co-transmitters/neuromodulators also exist). NE released from the sympathetic nerve binds primarily to alpha adrenergic receptors causing vasoconstriction (arterioles) and venoconstriction (venules and veins). Every vascular bed contains alpha adrenergic receptors. Activation of some sympathetic nerves also causes vasodilation (sympathetic active vasodilator nerves). The principle tissues are skeletal muscle and skin. Activation of these nerves is responsible for the rapid, brief increase in muscle blood flow during emotional arousal ("fight or flight response"), the neurotransmitter may be acetylcholine. In skin, active vasodilation is responsible for ~ 90% of the increase in skin blood flow in non-apical areas during hyperthermia. The neurotransmitter is unknown. 10 Donal S. O'Leary, Ph.D. Cardiovascular Physiology The parasympathetic nervous system innervates blood vessels in the genitals, heart and brain. Activation of these nerves releases acetylcholine (Ach). Ach binds to muscarinic receptors on the endothelium causing generation and release of nitric oxide. CIRCULATING FACTORS: Renin - angiotensin II system: In response to a decrease in arterial pressure at the kidney, an increase in renal sympathetic nerve activity, and/or a decrease in Na+ load (Na+ concentration x tubular flow) the enzyme renin is released by the juxtaglomerular cells from the kidneys into the blood. In the blood, renin acts on a protein angiotensinogen (which is released from the liver) and converts it to angiotensin I. Angiotensin I has little biological activity. Angiotensin I is converted by angiotensin converting enzyme (present many places but in high concentration in the lungs) to angiotensin II. Circulating angiotensin II activates vascular AII receptors and causes vasoconstriction. Angiotensin II also acts centrally to increase sympathetic activity. Vasopressin (antidiuretic hormone) Released from the pituitary activates V1 receptors on vascular smooth muscle causing vasoconstriction. NOREPINEPHRINE AND EPINEPHRINE: Released by the adrenal medulla in response to sympathetic stimulation. Epinephrine acts primarily on vascular beta 2 adrenergic receptors and causes vasodilation. Skeletal muscle is one vascular bed with a rich supply of 11 Donal S. O'Leary, Ph.D. Cardiovascular Physiology beta 2 receptors. However, in high concentrations or in vascular beds which lack beta 2 receptors epinephrine can bind to alpha adrenergic receptors and induce vasoconstriction. Epinephrine also acts on cardiac beta1 receptors to increase heart rate and contractility. Norepinephrine acts on vascular alpha receptors and cardiac beta1 receptors. -----------------------------------NOTES-------------------------------------- 12