Blood Physiology Erythropoiesis PDF
Document Details
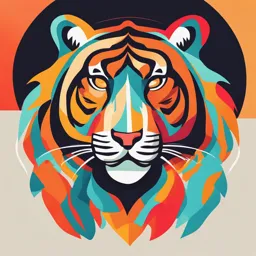
Uploaded by HumourousLouvreMuseum
كلية الطب جامعة ذي قار
Tags
Summary
This document discusses blood physiology, specifically erythropoiesis. It details the functions of red blood cells and the process of their formation. The text also touches upon the regulation and factors affecting erythropoiesis.
Full Transcript
Blood Physiology Erythropoiesis objectives: - RBCs orgin. - regulation of erythropoiesis - Erythrocytes metabolism - Types of anemias - Causes of Polycythemia )1( Red blood cells (erythrocytes): The primary functions of red blood cells are to transport oxygen from the l...
Blood Physiology Erythropoiesis objectives: - RBCs orgin. - regulation of erythropoiesis - Erythrocytes metabolism - Types of anemias - Causes of Polycythemia )1( Red blood cells (erythrocytes): The primary functions of red blood cells are to transport oxygen from the lungs to the various tissues of the body, and to transport carbon dioxide from the tissues to the lungs. Red blood cells (RBCs) are non-nucleated, biconcave discs. Because they lack of mitochondria and ribosomes, RBCs are incapable of aerobic respiration. This prevents them from consuming the oxygen they are meant to transport to other tissues. The red cell membrane is flexible and exhibits a remarkable deformability; the RBC being able to change its shape as it passes through narrow capillaries and then return, undistorted, to its original biconcave shape. The biconcave shape of the RBC, which provides a high surface to volume ratio, allows for maximum surface area (which facilitates gas transport) and greatest deformability. Cytoskeletal proteins (spectrin and actin) give membrane durability and flexibility to withstand the stretch and bend as squeezed through small capillaries Circulating erythrocytes live for about 120 days. As an RBC ages and its membrane proteins (especially spectrin) deteriorate, the membrane grows increasingly fragile. Without a nucleus or ribosomes, an RBC cannot synthesize new spectrin. Many RBCs die in the spleen, which has been called the "erythrocyte graveyard." The spleen has channels as narrow as 3 μm that severely test the ability of old, fragile RBCs to squeeze through the organ. Old cells become trapped, broken up, and destroyed. An enlarged and tender spleen may indicate diseases in which RBCs are rapidly breaking down. The average normal RBC count in adult male is 5,200,000 ± 300,000 per microliter of blood, and in adult female is 4,700,000 ± 300,000 per microliter of blood. At birth, the average RBC count is about 5,700,000 per microliter of blood. The number of RBCs varies with age, sex, and altitude. Each RBC has a mean diameter of about 7.5 micrometers and a thickness of 2.5 micrometers at the thickest point and 1 micrometer or less in the center (figure 2). The surface area of the RBC is about 140 square micrometers. The main constituent of the RBC is hemoglobin. Erythropoiesis: It is the process of erythrocyte formation or production. Erythropoiesis occurs at different anatomical sites during the course of development from embryonic to adult life, and as mentioned above. Stages of Erythropoiesis (Stages of differentiation of RBCs): Maturation and differentiation of RBC is shown in figure 1. Maturation proceeds with hemoglobin formation in the cytoplasm. After the cytoplasm of late normoblast is filled with hemoglobin and the nucleus is extruded from the cell and the endoplasmic reticulum is reabsorbed, at this stage the cell is called reticulocyte. During the reticulocyte stage, the cell passes to the blood and after 1-2 days in blood, it becomes mature erythrocyte. The concentration of reticulocytes among all the red cells of the blood is normally 0.5% -1.5% in adults. Reticulocyte count is used as a clinical measurement of erythropoietic activity. The basic substances needed for normal RBC and hemoglobin production are amino acids (proteins), iron, vitamin B12, folic acid, and vitamin B6. Regulation of erythropoiesis: The main factor stimulating RBC production is hypoxia (O2 deficiency inside the cells). Any condition that causes the quantity of O2 transported to the tissues (O2 carrying capacity of the blood) to decrease (decreased tissue oxygenation), increases the rate of RBC production. Examples on factors that decrease tissue oxygenation are: 1. At very high altitudes, O2 quantity in air is greatly decreased, and insufficient O2 is transported to the (2) tissues. 2. Diseases of the heart and lungs. 3. Anemia. On the other hand, when the rate of O2 transport to the tissues rises above normal, the rate of RBC production is depressed. Hypoxia increases the rate of RBC production by stimulating the secretion of the important regulating hormone "erythropoietin". So hypoxia does not act directly on bone marrow, but it causes marked increase in erythropoietin production and the erythropoietin stimulates RBC production until tissue hypoxia is relieved. In the normal person, about 90% of all erythropoietin is formed in the kidneys, and the remainder is formed in other tissues, mainly the liver. It has a half-life of hours and is broken down in the liver. When both kidneys are removed from a person or when the kidneys are destroyed by renal disease, the person invariably becomes very anemic, because only 10% of the normal erythropoietin formed in other tissues (mainly in the liver) which are insufficient to form RBC needed by the body. Other factors stimulating erythropoietin production include androgens, cobalt salts, epinephrine and norepinephrine, and several of the prostaglandins. Androgens (male sex hormones) can also stimulate erythropoietin production, and it is for this reason that RBC count in male is more than in female. Effect of erythropoietin on erythropoiesis: Erythropoietin is a glycoprotein. It stimulates formation of proerythroblasts from committed stem cells (CFU-E) in bone marrow, and once these proerythroblasts are formed, the erythropoietin causes these cells to pass more rapidly through the different erythroblastic stages than normally, further speeding up the production of new cells. The rapid production of cells continues as long as the person remains in the low oxygen state or until enough red blood cells are produced to carry adequate amount of O2 to the tissues despite the low oxygen. At this time, the rate of erythropoietin production decreases to a level that will maintain the required number of red cells but not an excess. IL-1, IL-3, IL-6, and GM-CSF also play part in erythropoiesis by their role in the development of the CFU-E stem cells (as explained earlier). Human erythropoietin can be produced by recombinant deoxyribonucleic acid (DNA) technology. It is used for management of anemia in cases of chronic renal failure, for treatment of chemotherapy- induced anemia in persons with malignancies, and treatment of anemia in persons with human immune deficiency virus (HIV) infection who are being treated with zidovudine. Erythrocyte metabolism: The RBC anaerobic glycolysis (figure 3B) is importance for the following reasons: 1. Provide energy, in terms of ATP molecules, to various biological activities of RBCs through anaerobic (3) Embden-Meyerhof glycolysis pathway. 2. Provide Nicotinamide adenine dinucleotide phosphate (NADPH) against oxidative stress. NADPH is the reduced form of NADP+. The only source of NADPH in RBCs is via the Hexose monophosphate shunt. Erythrocytes require NADPH to maintain normal levels of reduced glutathione (GSH) that is required to counteract against oxidative stress. This is because oxygen is toxic and without reduced glutathione, peroxides spontaneously formed from molecular oxygen would oxidize the lipid components of the red blood cell membranes. Oxidized membranes are significantly less flexible than normal membranes, and result in damage to the red blood cells when the cells attempt to transit capillaries. In addition, peroxides tend to damage hemoglobin, resulting in precipitation of the protein. Insoluble aggregates of hemoglobin have severely impaired oxygen carrying capacity, and insoluble protein aggregates tend to be inflexible enough to prevent the normal deformations of the red blood cell. 3. Provides nicotinamide adenine dinucleotide (NAD+) in red blood cells is required to keep the Hb in ferrous state. Oxygen tends to oxidize the hemoglobin iron from +2 to the more stable +3 oxidation state (resulting in methemoglobin). This is a problem: the +3 state of heme iron binds oxygen very poorly. NAD+ is used to supply reducing equivalents to methemoglobin reductase, the enzyme that returns the hemoglobin to the +2 oxidation state. 4. To produce 2,3 diphosphoglycerate through Rapaport-Luebering pathway for regulation of Hb affinity to O2. )4( Anemia: It is defined as a reduction in blood Hb level and/or in RBC count below the normal range for the patient's age and sex. Classification of anemia: Anemia can be classified, according to the cause, into anemia due to: 1. Inadequate production of normal RBCs by the bone marrow. 2. Excessive destruction of RBCs (hemolysis). 3. Blood loss (hemorrhage). Inadequate production of normal RBCs by the bone marrow: Examples: 1. Due to deficiency of essential factors (iron, vitamin B12 and folic acid). 2. Aplastic anemia (bone marrow aplasia). Vitamin B12 and Folic acid are required for DNA synthesis, so they are important for maturation of RBCs. If B12 or folic acid is deficient, DNA synthesis and nuclear maturation is slowed, whereas cytoplasmic maturation (largely dependent on RNA function) is relatively unimpeded. Consequently, erythropoiesis is delayed with production of the erythroblastic cells in the bone marrow, which grow but cannot divide rapidly and become larger than normal (called megaloblasts). The production of larger than normal erythrocytes (called macrocytes) which are abnormal in shape and break easily leading to decreased number of RBCs in blood and anemia develops which is called megaloblastic anemia or maturation failure anemia. B12 deficiency can occur due to lack of B12 in diet or more commonly due to lack of a factor (intrinsic factor) which is secreted by gastric mucosa and is bound to B12 so that to protect it from digestive enzymes and also assists in absorption of vitamin B12 in the ileum. In a condition called "pernicious anemia" there is failure of secretion of intrinsic factor by stomach due to atrophy of gastric mucosa, so megaloblastic anemia develops. In aplastic anemia, bone marrow may be destroyed and become unable to produce blood cells, such as following excessive x-ray exposure or the use of certain drugs that cause bone marrow aplasia (lack of functioning bone marrow). Excessive destruction of RBCs (hemolysis): Hemolytic anemia results from abnormalities of red cell membrane or Hb, or other causes. In which there is excessive destruction of RBCs. Examples are hereditary spherocytosis (a common membrane defect, in which the RBCs are small and spherical in shape, and fragile), sickle cell anemia, deficiency of G6PD (misleadingly also called favism), and erythroblastosis fetalis. Blood loss (hemorrhage): Acute hemorrhage is loss of large volume of blood over a short period. After rapid hemorrhage, the body replaces plasma within 1-3 days, but this leaves a low concentration of RBCs, which will return to normal within 3-4 weeks if no more hemorrhage occurs. Chronic hemorrhage is loss of small volume of blood over long period. Therefore, this person needs continuous formation of new RBCs, so he needs more iron than normal, with time if this person does not receive extra iron, store of iron is going to be depleted, and then the person will suffer from iron deficiency anemia. (5) Anemia has potential consequences: 1. The tissues suffer hypoxia (oxygen deprivation). Severe anemic hypoxia can cause life -threatening necrosis of brain, heart, and kidney tissues. 2. Blood osmolarity is reduced. More fluid is thus transferred from the blood stream to the intercellular spaces, resulting in edema. It is suggested that the low concentration of Hb in patients with anaemia causes a reduced inhibition of basal endothelium-derived relaxing factor (NO) activity and leads to generalised vasodilatation and consequently low blood pressure. The consequent of low blood pressure may be the stimulus for neurohormonal activation and salt and water retention. 3. Blood viscosity is reduced. 4. The heart and lungs also must work harder to compensate for the blood's low capacity to carry oxygen. Because the heart has to work harder to get blood and oxygen to the tissues, anemia, particularly severe anemia, can result in cardiac failure and arrest. Polycythemia: It is an increased concentration of erythrocytes in the circulating blood that is above normal for sex and age. Polycythemia could be: 1. Relative polycythemia is due to reduction in plasma volume. This may occur because of dehydration that occurs in conditions such as diarrhea. 2. Absolute or true polycythemia, which could be; a. Secondary polycythemia that is related to increased erythropoietin production. It is seen for example in those living at high altitudes. b. Primary polycythemia (polycythemia vera) is caused by a gene aberration that occurs in the cell line that produces the blood cells. The blast cells continue producing red cells even when too many cells are already present. This causes excess production of red cells without erythropoietin stimulus, and usually there is excess production of white blood cells and platelets as well. The principal dangers of polycythemia are increased blood volume, blood pressure, and viscosity. Blood volume can double in primary polycythemia and cause the circulatory system to become tremendously engorged. Blood viscosity may rise to three times normal. Circulation is poor, the capillaries are clogged with viscous blood, and the heart is dangerously strained. Factors affecting blood viscosity: The plasma proteins and formed elements (red cells, white cells, and platelets) increase the viscosity of blood. Of these formed elements, red cells have the greatest effect on viscosity. Therefore, viscosity is strongly dependent on Hct, as Hct increases, there is a disproportionate increase in viscosity. Temperature: As temperature decreases, viscosity increases (increases ~ 2% for each °C decrease in temperature) Flow rate of blood: Low flow rates marked increased in viscosity Increased cell-to-cell and protein-to-cell adhesive interactions Erythrocytes adhere to one another (rouleau formation) Vessel diameter: Small vessel diameters (e.g., in arterioles less than 300 microns), there is a paradoxical decrease in blood viscosity (Fahraeus-Lindqvist effect). This occurs because the hemotocrit decreases in small vessels relative to the hemotocrit of large feed arteries. )6(