Personal Strategies to Mitigate Air Pollution PDF
Document Details
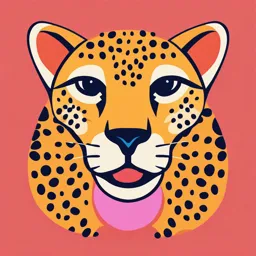
Uploaded by HopefulCalcium
School of Kinesiology
Andy Hung, Sarah Koch, Valerie Bougault, Cameron Marshall Gee, Romulo Bertuzzi, Malindi Elmore, Paddy McCluskey, Laura Hidalgo, Judith Garcia-Aymerich, Michael Stephen Koehle
Tags
Summary
This document is a consensus statement on personal strategies to mitigate the effects of air pollution exposure during sport and exercise. It reviews the current state of evidence and provides recommendations to prevent and mitigate adverse health and performance effects. The review is from the Canadian Academy of Sport and Exercise Medicine and the Canadian Society for Exercise Physiology.
Full Transcript
Personal strategies to mitigate the effects of air pollution exposure during sport and exercise: a narrative review and position statement by the Canadian Academy of Sport and Exercise Medicine and the Canadian Society for Exercise Physiology Andy Hung ,1 Sarah Koch ,2,3 Valerie Bougault ,4...
Personal strategies to mitigate the effects of air pollution exposure during sport and exercise: a narrative review and position statement by the Canadian Academy of Sport and Exercise Medicine and the Canadian Society for Exercise Physiology Andy Hung ,1 Sarah Koch ,2,3 Valerie Bougault ,4 Cameron Marshall Gee ,5,6 Romulo Bertuzzi,7 Malindi Elmore,6 Paddy McCluskey,6,8 Laura Hidalgo,2,3 Judith Garcia-Aymerich,2,3 Michael Stephen Koehle 1,9 ► Additional supplemental material is published online only. To view, please visit the journal online (http://d x.doi. org/1 0.1136/bjsports-2022- 106161). For numbered affiliations see end of article. Correspondence to Dr Michael Stephen Koehle, School of Kinesiology, The University of British Columbia, Vancouver, Canada; m ichael.koehle@u bc.ca Accepted 17 November 2022 ABSTRACT Air pollution is among the leading environmental threats to health around the world today, particularly in the context of sports and exercise. With the effects of air pollution, pollution episodes (eg, wildfire conflagrations) and climate change becoming increasingly apparent to the general population, so have their impacts on sport and exercise. As such, there has been growing interest in the sporting community (ie, athletes, coaches, and sports science and medicine team members) in practical personal-level actions to reduce the exposure to and risk of air pollution. Limited evidence suggests the following strategies may be employed: minimising all exposures by time and distance, monitoring air pollution conditions for locations of interest, limiting outdoor exercise, using acclimation protocols, wearing N95 face masks and using antioxidant supplementation. The overarching purpose of this position statement by the Canadian Academy of Sport and Exercise Medicine and the Canadian Society for Exercise Physiology is to detail the current state of evidence and provide recommendations on implementing these personal strategies in preventing and mitigating the adverse health and performance effects of air pollution exposure during exercise while recognising the limited evidence base. INTRODUCTION © Author(s) (or their employer(s)) 2023. No commercial re-use. See rights and permissions. Published by BMJ. To cite: Hung A, Koch S, Bougault V, et al. Br J Sports Med Epub ahead of print: [please include Day Month Year]. doi:10.1136/ bjsports-2022-106161 Health benefits and physiological responses to exercise and sport are well known.1 Climate change, characterised by more extreme, more frequent and longer- lasting exposures to environmental threats, has the potential to alter typical physiological responses to, and known health benefits of exercise.2 For example, exercise-induced elevations in ventilation, core temperature, blood flow and an upregulation of the metabolism may catalyse harmful effects of inhaled pollutants when exposed to poor air quality. Increased inhaled doses, deposition of pollutants in the respiratory tract and faster circulation of absorbed particles and gases within the systemic circulation can affect nearly all organs, impact exercise performance, and, in the worst case, harm athletes’ health.3–6 Joint investigations in the USA (Medicare), Canada (MAPLE) and Europe (ELAPSE) show that air pollution exposure at all levels, even low concentrations, affects health.7–9 As a result, in September 2021, the WHO released drastically reduced new thresholds for key ambient pollutants.10 More research is needed to fully describe the health risks and possibly modified benefits of exercise in air pollution and their effects on athletic performance.4–6 As training and competitions at venues with increased air pollution exposures are becoming more common,11 strategies to protect athletes’ health and to enable them to achieve their best possible athletic performance need to be developed. The purpose of this position statement is to provide evidence- informed recommendations on personal strategies to prevent or mitigate adverse health and performance effects of air pollution exposure during exercise. AIR POLLUTION COMPONENTS, SOURCES AND HEALTH EFFECTS Air pollution is a complex mixture of gases and particles.10 12 Athletes’ health effects attributed to air pollution mixtures are heterogeneous and influenced by the toxicity of the respective individual components, which vary over space and time. Below, biological, chemical and physical characteristics of particulate matter (PM), ozone (O3), nitrogen oxides (NOx), sulfur oxides (SOx), carbon monoxide (CO) and volatile organic compounds (VOCs), the pollutants with the largest public health relevance, are described, and their most common sources listed. For each pollutant, the best- understood effects on physiological systems relevant for athletic performance are briefly summarised. However, although individual pollutants are discussed in this article, synergies between individual components may exist for which there are little data. Common air pollutant abbreviations are listed in table 1 for reference and the WHO’s air quality guidelines are presented in figure 1. Particulate matter PM is a complex mixture of solid particles and liquid droplets comprising various acids, organic chemicals, metals, pollen and small particles of soil, dust, or carbon.13 Particles can be directly emitted Hung A, et al. Br J Sports Med 2023;0:1–10. doi:10.1136/bjsports-2022-106161 1 Br J Sports Med: first published as 10.1136/bjsports-2022-106161 on 9 January 2023. Downloaded from http://bjsm.bmj.com/ on January 9, 2023 at The University of British Columbia Library. Protected by copyright. Consensus statement Table 1 Air pollutant abbreviations Abbreviation Meaning CO Carbon monoxide DE Diesel exhaust NO2 Nitrogen dioxide NOx Nitrogen oxides O3 Ozone PM Particulate matter PM10 Thoracic particles (PM with nominal mean aerodynamic diameter≤10 µm) PM2.5 Fine particles (PM with nominal mean aerodynamic diameter≤2.5 µm) SO2 Sulfur dioxide SOx Sulfur oxides TRAP Traffic-related air pollution UFP (or PM0.1) Ultrafine particles (PM with nominal mean aerodynamic diameter≤0.1 µm) VOCs Volatile organic compounds Ozone O3 is a highly reactive form of oxygen (O2).14 Ground-level O3 is created by chemical reactions between NOx and VOCs in the presence of sunlight. Industrial facilities and electric utilities, motor vehicle exhaust, gasoline vapours and chemical solvents are major sources of NOx and VOCs.14 Due to its association with UV radiation, O3 levels are often increased during hot, sunny weather. As such, high O3 and high ambient temperatures often coincide. In recent years, global exposure to O3 has increased,15 reflecting an increased emission of O3 precursors, such as NOx, coupled with warmer temperatures, resulting from climate change. O3 is associated with respiratory disease independent of PM exposure.14 Symptoms provoked by O3 exposure include cough, sore or scratchy throat, pain on deep inspiration and difficulty breathing.14 In those with pre-existing respiratory disease who are sensitive to O3, symptoms may be dramatically worsened, and the frequency of asthma attacks increased, with a decrease in asthma control with regular medication.14 Nitrogen oxides from a source, such as construction sites, unpaved roads, fields, smokestacks or fires. However, the majority of PM forms in the atmosphere in complex reactions from chemicals such as sulfur dioxide (SO2) and NOx, pollutants emitted from power plants, industrial zones and motorised transport. The composition, size, shape and concentration of particles define their potential for health threats.13 The smaller the particle, the greater the likelihood that it will penetrate more deeply into the airways, deposit in the respiratory tract and impair health.13 Three categories are typically used to classify PM based on their diameter: PM≤10 µm (PM10), PM≤2.5 µm (PM2.5) and PM≤0.1 µm (PM0.1), which are often referred to as ultrafine particles (UFP). Exposure to PM is associated with negative effects on respiratory and cardiovascular health, including alterations in respiratory symptoms (eg, wheeze), lung function, oxidative stress, inflammation, heart rate (HR), blood pressure (BP) and cognition.13 NOx are highly reactive chemicals that contribute to the generation of smog, a brown haze often seen above cities when NOx and VOCs react with sunlight, and acid rain, the product of NOx, SO2, water and O2.16 17 NOx is formed primarily from the release of nitrogen contained in fuel during combustion or through natural events such as forest fires or volcanic eruptions.16 Combined with other compounds in the atmosphere, such as ammonia, nitrate becomes an important contributor to the secondary formation of PM2.5 and O3.13 14 Exposures to high NO2 concentrations are associated with aggravated respiratory symptoms including coughing, wheezing and difficulty breathing.16 Longer exposures to elevated NO2 concentrations may contribute to the pathogenesis of asthma and increase the susceptibility to respiratory infections.16 Sulfur oxides SO2 belongs to the family of SOx gases.18 Over 90% of SOx emissions are released in the form of SO2, a colourless gas with a distinct sulfurous pungent odour. It originates from the sulfur contained in raw materials such as coal, oil and metal-containing ores during combustion and refining processes.19 When dissolved in water vapour in the air, SO2 forms acids (sulfuric acid and sulfate) and interacts with other gases and particles to form sulfates that can be harmful, particularly to the respiratory tract.18 SO2 irritates the eyes, mucous membranes, skin and respiratory tract.19 Asthmatics appear to be particularly affected by SOx, with significant decreases in forced expiratory volume in 1 s (FEV1) and forced vital capacity (FVC), which can be aggravated further by exercise.20 Carbon monoxide Figure 1 2 2021 WHO global air quality guidelines. CO is a product of incomplete combustion of hydrocarbon- based fuels and presents as a colourless, odourless, tasteless, but poisonous gas.21 The most common sources of CO emissions are vehicles, wood industry, residential wood heating and forest fires. Following the inhalation of CO, it rapidly diffuses across the alveolar membrane and binds reversibly to haemoglobin with a 200-fold greater affinity than O2 to form carboxyhaemoglobin.16 Carboxyhaemoglobin inhibits the blood’s capacity to carry O2 to organs and tissues, resulting in tissue hypoxia and impaired exercise performance.22 Organs primarily targeted by CO poisoning are the central nervous system and the heart. Maximum cardiac output and maximal arteriovenous difference are lowered Hung A, et al. Br J Sports Med 2023;0:1–10. doi:10.1136/bjsports-2022-106161 Br J Sports Med: first published as 10.1136/bjsports-2022-106161 on 9 January 2023. Downloaded from http://bjsm.bmj.com/ on January 9, 2023 at The University of British Columbia Library. Protected by copyright. Consensus statement with CO in the bloodstream, decreasing maximum oxygen uptake (V̇ O2max) and work output.23 The relationship between CO exposures and respiratory health outcomes is unknown. Common symptoms of CO poisoning are confusion, nausea, headache, dizziness, fatigue and drowsiness. CO poisoning can impact the ability to perform complex tasks and reduce exercise capacity, visual perception and manual dexterity.16 24 Volatile organic compounds VOCs refer to all compounds with high vapour pressure and low water solubility and are often components of petroleum fuels, hydraulic fluids, paint thinners and dry-cleaning agents.25 They are also emitted from diesel exhaust (DE). When combined with NOx, they react to form ground-level O3. In the outdoors, VOCs create smog under certain conditions. The health effects of VOCs depend on the nature of the VOCs, the concentration and duration of exposure, respectively. Benzene and formaldehyde, two better-researched VOCs, are listed as human carcinogens.26 Long-term exposure to VOCs can cause damage to the liver, kidneys and central nervous system. Short-term exposure to VOCs can cause eye and respiratory tract irritation, headaches, dizziness, visual disorders, fatigue, loss of coordination, allergic skin reactions and nausea.26 INTERSECTION OF AIR POLLUTION EXPOSURE AND EXERCISE Exposure pathways Exposure pathways of humans to air pollutants include inhalation, ingestion, dermal absorption and non-conventional routes, such as absorption through the eyes.27 The primary route of exposure for most vapours, gases and particles is inhalation. Once inhaled, chemicals are either exhaled or deposited in the respiratory tract. If deposited, damage can occur through direct contact with tissue or diffusion through the lung–blood interface into the bloodstream on a systemic level. Once in contact with tissue in the upper respiratory tract or lungs, chemicals may induce effects ranging from simple irritation to severe tissue damage. Substances absorbed into the blood are circulated and distributed to organs, where they have the potential to cause further impairment. In the context of exercise, exposure to air pollution via inhalation is of particular interest. Increases in minute ventilation (V̇ E), airway airflow patterns and transitions from nasal to oral breathing are considered to increase the dose of air pollutants reaching and depositing into more distal parts of the respiratory tract.28 29 Indeed, Daigle et al30 reported a 4.5- fold higher deposition of UFPs in the respiratory tract following four 15 min long exercise bouts (V̇ E=38.1±9.5 L/min) with 15 min long rest breaks in between compared with rest only (V̇ E=9.0±1.3 L/min). Furthermore, para-athletes may have individual factors that affect their air pollution exposure. For example, in high-level spinal cord injury,31 expiratory muscle weakness and larger residual volumes may put these athletes at a greater risk for PM accumulation due to larger dead space ventilation (i.e. diminished airflow and exchange). Effects on general health Long-term effects appear to vary by pollutant, with O3 having the most convincing interaction with exercise.4 For example, long-term physical activity in high O3 environments results in an increased risk of asthma in children.32 However, in adults, a large study from Denmark with relatively low exposures to NO2 reported no interaction effects between air pollution exposure and physical activity levels on mortality, the incidence of asthma, Hung A, et al. Br J Sports Med 2023;0:1–10. doi:10.1136/bjsports-2022-106161 chronic obstructive pulmonary disease and myocardial infarction.33–35 Two other studies in adults showed a protective effect of physical activity against adverse health effects of air pollution on premature mortality and impaired lung function.36 37 In summary, a recent mapping review confirmed previous findings that the health benefits of physical activity generally outweigh the health risks of air pollution in healthy individuals5; however, insufficient evidence is available for athletes whose training volumes are very high, making them a population group that needs to be studied further. In this position statement, we propose evidence-informed strategies to mitigate the deleterious health and performance effects of air pollution during training and competition. METHODS A steering committee (MSK, AH and SK) initially convened and determined the objectives of the position statement. The committee then identified and invited an expanded panel of experts with clinical and/or academic experience in exercise and air pollution. The panel, consisting of an elite athlete and experts in sports medicine, family medicine, pulmonology, epidemiology, exercise physiology and parasport, was carefully selected to ensure a diverse and balanced perspective. MEDLINE, Embase, Cochrane Central Register of Controlled Trials, SPORTDiscus and the Agricultural and Environmental Science Database were searched for peer-reviewed publications related to air pollution, exercise and strategies preventing or mitigating the effects of air pollution. We excluded publications focusing on people with respiratory or cardiovascular conditions, with the exception of asthma and exercise-induced bronchoconstriction (EIB) given their prevalence in athletic populations.38 No date restrictions were applied, and a final search was conducted on 1 March 2022. The reference lists of the included studies were handsearched for potentially missed articles. An example search strategy is available in online supplemental appendix 1. Given the limited evidence base identified in a preliminary search, as well as the necessity to incorporate expert experiential perspectives, a narrative review was selected in lieu of a systematic review. Due to the paucity of sufficient published evidence, Grading of Recommendations, Assessment, Development and Evaluations39 and other consensus methodologies were not applied. The steering committee appraised the literature and compiled the initial draft. The draft was subsequently reviewed by the extended panel of experts. The final version of the position statement was approved by all authors and the Canadian Academy of Sport and Exercise Medicine and Canadian Society for Exercise Physiology board of directors. A summary of the results and recommendations is provided (figures 2 and 3). EXERCISE INTENSITY The inhaled dose of air pollution (i.e. concentration × ventilation × time) generally increases with exercise intensity due to a proportional increase in V̇ E.40 As such, given the known dose–response relationship between air pollution and health outcomes,10 an increase in exercise intensity could theoretically augment the health and performance effects of air pollution. Indeed, public health agencies generally recommend the curtailment or complete avoidance of intense activities during periods of poor air quality.41 Nevertheless, limited evidence suggests the contrary. To our knowledge, only one study has investigated the differential responses to low- intensity and high- intensity exercise performed at high levels of PM. In a double-blinded crossover 3 Br J Sports Med: first published as 10.1136/bjsports-2022-106161 on 9 January 2023. Downloaded from http://bjsm.bmj.com/ on January 9, 2023 at The University of British Columbia Library. Protected by copyright. Consensus statement runners: a moderate 1-hour continuous exercise bout at V̇ E=80 L/min and one with a succession of 30 min warm-up and 30 min competition intensity (V̇ E=112 L/min).46 The decrement in lung function was comparable between bouts, indicating that the higher intensity did not exacerbate the O3 effect. Taken together, the results of these laboratory studies do not support the premise that increased exercise intensities potentiate the adverse effects of air pollution. While further research is warranted, the goal at present is not to specifically avoid high-intensity efforts per se, but rather to reduce the total inhaled dose over the course of the bout. Summary ► Aim to minimise the total inhaled dose (i.e. the product of air pollution concentrations, ventilation rate and duration of the exercise bout) of air pollution during an exercise bout. REDUCING EXPOSURE BY TIME AND DISTANCE Figure 2 Infographic summary of personal strategies to mitigate the effects of air pollution exposure during exercise. trial, 18 recreationally active males performed two 30 min exercise bouts while exposed to high concentrations of DE (300 µg/ m3 of PM2.5): once at a low-intensity (30% V̇ O2peak) and once at a high-intensity (60% V̇ O2peak).42–44 Interestingly, low-intensity and high-intensity cycling in high levels of DE did not produce differences in respiratory function or inflammation, autonomic function, norepinephrine, circulating NOx and systemic inflammation.42–44 Even more surprisingly, the authors found that even though low-intensity exercise under DE exposure significantly increased V̇ E, V̇ O2, carbon dioxide production, and the O2 cost of exercise compared with filtered air, no exposure effect was found for the high-intensity cycling bout.45 One relevant study has been conducted comparing two modalities of exercise designed to deliver similar inhaled doses of O3 in 10 competitive male Figure 3 Summary of key recommendations to minimise effects of air pollution during exercise. 4 A fundamental strategy is to distance oneself from air pollution by time and distance. Pollution exposure varies considerably across all time frames from hours to months. Within-day variations can be large and are affected by local factors such as weather (e.g. wind, ambient temperature, precipitation), traffic patterns, topography and the built environment.47 Since ground- level O3 is generated in response to ultraviolet light, O3 levels typically peak in the afternoon, whereas particulate levels seem to peak in the evening, returning to baseline levels by early the next morning.13 48 Thus, in the absence of extraordinary factors, early in the morning is usually a low pollution time of day. At the other end of the timescale, there are seasonal variations in pollution as well. For example, certain regions of the world have strong seasonal variations in pollution, such as the wildfire season in Western North America and the haze season in Southeast Asia. Although these seasonal pollution events can last days or weeks, changes in wind, weather and geography can lead to local variations in exposure which can be used to choose a training location that minimises exposure. Location is also key when considering air pollution exposure. In general, urban sites have poorer air quality than rural sites, although significant environmental events (e.g. wildfires) can alter this relationship. Within urban areas, pollution exposure is extremely variable and small changes in location by individuals can lead to significant reductions in air pollution exposure. For example, it is clear that levels drop significantly within a small distance from major traffic arteries and many pollutants (e.g. CO, PM and NOx) reach background levels by 400 m from a major road49; that is, the levels of air pollution that would occur in the absence of anthropogenic emissions. Of course, concentration–distance relationships vary with air pollutants, with some pollutants (e.g. O3) exhibiting less spatial variability in urban environments.14 Nonetheless, individuals are encouraged to physically distance themselves as much as possible from significant sources of air pollution. For example, cycle or walking commuters should consider using smaller streets and greenways when safe to do so, whereas outdoor workouts should plan to take place towards the centre of green spaces. It must be considered, however, that for some exercisers of differing abilities (e.g. wheelchair athletes), relocating an exercise session and accessing green spaces on short notice may pose additional challenges. Summary ► While highly variable, air pollution levels are generally lowest early in the morning. Athletes may also consider Hung A, et al. Br J Sports Med 2023;0:1–10. doi:10.1136/bjsports-2022-106161 Br J Sports Med: first published as 10.1136/bjsports-2022-106161 on 9 January 2023. Downloaded from http://bjsm.bmj.com/ on January 9, 2023 at The University of British Columbia Library. Protected by copyright. Consensus statement participating in events when local seasonal events (e.g. wildfires, haze) are less likely. ► Athletes are encouraged to physically distance themselves from sources of air pollution, such as major roads and highways, and towards green spaces. MONITORING AIR POLLUTION LEVELS To optimise the timing and location of exercise, a good understanding of current and forecasted pollution levels is key. This can be achieved by using services that provide current and future air pollution conditions for locations of interest. Local government websites and apps provide trustable data. For example, in Canada, the following government site provides good quality real- time data with reasonable granularity in terms of location: https://weather.gc.ca/airquality/pages/index_e.html. These websites and apps even have utility when planning the location for outdoor exercise. In larger urban centres, conditions are typically monitored at multiple locations, so users can compare different locations within an urban agglomeration to determine where the pollution is lowest. Once the general region for activity is determined, then the individual can choose the precise location of activity based on local factors (e.g. towards the centre of green spaces and away from local sources of pollution). Air quality indices, aggregate measures characterising air pollution concentrations present at a given moment in time, and their associated health risks are reported by many public health authorities to raise awareness of current local air pollution levels.50 However, different indices may be used between countries, reflecting different pollutants of interest, air quality standards and an overall lack of international consensus. Consequently, caution is warranted in directly comparing different air quality indices. In Canada, the reported Air Quality Health Index (AQHI) was developed to reflect the additive effects of NO2, O3 and PM2.5.50 In older adults exercising outdoors in rural and urban settings, the AQHI has been demonstrated to be predictive of acute subclinical adverse cardiorespiratory effects (e.g. decrease in HR variability, increased oxidative stress, decrease in FEV1).51–53 It must be acknowledged that most websites and indices generally rely on networks of fixed-site monitoring stations or remote sensing techniques that are designed to estimate population-wide exposures for regulatory purposes.54 While capable of providing accurate, long-term air quality data, monitoring stations have high construction costs and consequently are sparsely distributed and rarely situated near locations of interest in respect to exercise and sport. In other words, the data may be nonspecific to an individual athlete’s environmental context and incapable of providing actionable, hyperlocal, time-resolved data. World Athletics has begun important work in this area, deploying air pollution sensors inside the main athletics stadia to provide local measurements.54 55 Alternatively, low-cost wearable air pollution sensors present a potentially promising method for determining exposure levels.56 These sensors can theoretically overcome the spatial and temporal limitations of fixed-site station-derived air pollution estimates, providing athletes with the ability to independently acquire immediate and hyperlocal exposure data to quickly modify behaviour before or during a workout. While there has been a rapid proliferation of such devices in recent years, many devices still lack sufficient third-party validation. An objective sensor evaluation is provided by the South Coast Air Quality Management District in California (http://www.aqmd. gov/aq-spec/sensors). This group evaluates the performance of low- cost sensors against gold- standard reference instruments Hung A, et al. Br J Sports Med 2023;0:1–10. doi:10.1136/bjsports-2022-106161 and publishes their findings online. We do not recommend or endorse any specific sensors at this time. Summary ► Minimise air pollution exposure by monitoring current and forecasted levels of air pollution for locations of interest using trusted sources (e.g. local government websites). ► Affordable, wearable air pollution sensors theoretically allow for the timely assessment of personal air pollution levels. However, the performance of such sensors may be highly variable. INDOOR EXERCISE AS AN ALTERNATIVE Moving an exercise bout indoors may be an alternative to reduce exposure when ambient pollution is high. However, the unintended consequence may be increased exposure to indoor air pollutants. Consequently, the effectiveness of this strategy is dependent on the ratio of indoor-outdoor air pollution concentrations. In the discussion below, we will focus on several factors that influence this ratio, including the types and sources of air pollutants, degree of infiltration and available building ventilation.57 Indoor types and sources of air pollutants have been shown to vary greatly depending on the type of sporting facility. For example, while PM, CO and NO2 levels may be of particular concern in ice hockey arenas or skating rinks due to the use of propane-powered or gasoline-powered ice resurfacing machines (e.g. Zambonis), VOC concentrations may be of special concern in fitness centres given the frequent use of disinfectants.3 58 In general, some common sources of air pollution in sporting environments include ice resurfacing machines, dust, moulds, cleaning agents, paints, disinfectants, air fresheners, candles, climbing chalk and snowboard/ski wax. For a comprehensive overview of the relevant types and sources of air pollutants in indoor sporting environments, we refer to a recent review by Salonen et al58 Indoor air quality is also influenced by the surrounding outdoor air pollution using natural building ventilation and infiltration (i.e. exchange of air pollutants between indoor and outdoor environments via cracks and leaks in a building).57 For example, Weichenthal et al conducted a crossover study in healthy women and compared the cardiovascular responses to cycling in outdoor or indoor settings.59 Similar PM2.5 between indoor and outdoor environments was reported, attributed to the presence of open windows.59 Similarly, another study found high concentrations of PM2.5 in an elementary school gym in Prague, that authors theorised as a penetration of traffic-related air pollution (TRAP) from a nearby traffic-dense street.60 Thus, in some buildings, the action of closing doors and windows could reduce the exchange of air, and subsequently, lower concentrations of indoor pollutants. However, the trade-off is potentially ‘trapping’ more air pollutants originating from indoor sources. Portable air cleaners (PAC) and central heating, ventilation and air conditioning systems with built- in filters (e.g. high- efficiency particulate arrestance (HEPA) filters) present promising solutions, despite a paucity of evidence on changes in health outcomes from their use.61 Nevertheless, when the ratio of indoor–outdoor air pollutant concentrations is favourable (i.e. indoor air quality exceeds outdoor air quality), limited evidence suggests that exercising indoors may confer physiological benefits. Several studies in healthy adults have shown that compared with indoor environments with air cleaned via a HEPA filter, exercising while 5 Br J Sports Med: first published as 10.1136/bjsports-2022-106161 on 9 January 2023. Downloaded from http://bjsm.bmj.com/ on January 9, 2023 at The University of British Columbia Library. Protected by copyright. Consensus statement breathing ambient air characterised by high levels of TRAP can impair glucose metabolism, increase systemic inflammation and attenuate postexercise hypotension.62–65 In another study of older adults in Brazil, a greater improvement in glycaemia, brain-derived neurotrophic factor and cognitive function was observed following 12 weeks of aerobic training in an indoor environment, relative to an outdoor urban park.66 Summary ► When ambient air pollution concentrations are high, it may be favourable to relocate an exercise bout indoors after considering the indoor air quality and potential coexposures (e.g. indoor temperatures). ► Indoor air quality can also be improved by controlling indoor sources of air pollution, optimising ventilation and using PAC fitted with HEPA filters. ACCLIMATION Acclimating to air pollution may be a viable performance strategy in certain populations (i.e. high-performance athletes). Multiple small laboratory studies involving consecutive daily short-term exposures to O3 during exercise have investigated the acclimation effect with respect to respiratory function and symptom responses. In general, these studies involved healthy adults or those with mild asthma intermittently exercising at light intensities while exposed to high concentrations of O3 (~200–500 ppb) for ~2 hours on 4–5 consecutive days.67 68 In these studies, postexercise FEV1 was worse with the initial 2 days of O3 exposure, before gradually improving with consecutive daily exposures, such that FEV1 on the last days of O3 exposure was similar to levels observed following an exercise bout in filtered air.67 68 However, this acclimation appears to persist for less than a week.68 69 One study examined the potential effects on performance outcomes, finding that after four consecutive days of 350 ppb O3 exposure, performance time and V̇ O2peak were no different than in the filtered air condition70; however, there is likely significant inter-individual variability in response. The O3 acclimation effect may be, in part, concentration- dependent.71 In one modelling study using a dataset of over 650 000 performance outcomes from outdoor collegiate athletes, those who experienced the greatest O3- induced detriment in performance were exposed to the lowest levels of O3 7 days before their event; this supports a potential acclimation effect dependent on exposure over several days.72 The potential for acclimation to other pollutants in relation to exercise is less clear. In one study involving 16 male, collegiate athletes performing two consecutive days of maximal exercise tests while exposed to high levels of PM (~340 000 particles/ cm3), no acclimation effect was observed.73 Only a single study was found to examine the effects of four consecutive daily exposures to NO2 during exercise: decrements in FEV1, FVC and antioxidant status were observed following the first day of exposure, but the magnitude of the decrements decreased following the fourth day.74 Nonetheless, the dose of NO2 used was several times larger than ambient levels. In summary, O3 appears to be the pollutant with the most potential for acclimation. The findings must be interpreted cautiously, given the very high O3 levels, limited endpoints and very low-intensity exercise used. It must therefore be acknowledged that the use of an O3 acclimation protocol remains speculative until the safety and real- world effectiveness of such a strategy can be properly assessed. 6 Summary ► Laboratory studies suggest that exposure to high levels of O3 on multiple, consecutive days may allow athletes to acclimate to the deleterious pulmonary effects of O3. ► Athletes should be aware that the safety and effectiveness of an O3 acclimation protocol, in the context of sport and performance, has yet to be directly tested outside of laboratory settings. EXPOSURE PRIOR TO COMPETITION Exposure to air pollution before an exercise bout may have cardiorespiratory and performance implications, even when the exercise itself is performed in optimal air quality. This is an important practical question, as athletes and exercisers are likely exposed to higher levels of air pollution during their travel to a sporting environment. For example, while bus commuting generally makes up <5% of one’s day, it can contribute between related 11%–70% of one’s daily exposure to certain traffic- exercise’ as the pollutants.75 For discussion, we refer to ‘pre- exposure to air pollutants hours prior to an exercise bout, whereas ‘acclimation’ refers to intentional multi-day protocols involving short-term exposures to high levels of air pollution. Two studies investigating the cardiorespiratory and perforexercise exposure have been conducted mance effects of pre- using controlled laboratory conditions, exposing participants to high levels of DE at 300 µg/m3 of PM2.5. In one study involving eight endurance-trained males, 60 min of resting exposure to DE prior to exercise increased exercise HR, and attenuated exercise-induced bronchodilation. However, performance on a 20 km cycling time trial was not affected.76 In the second study, constant load cycling performed 2.5 hours following a 2-hour resting pre-exercise exposure to DE impaired exercise tolerance, ventilatory responses and dyspnoea in 11 healthy, older adults.77 Minimising pre-exercise air pollution exposure is therefore recommended. For example, for organised sports involving team buses, individuals should avoid meeting in semiclosed transport hubs or leaving the bus running while waiting for the passengers to board. Alternatively, when possible, travel should be avoided altogether. While en route to training or competition, athletes can also minimise air pollution exposures by closing vehicle windows, turning on air conditioning and using cabin air filters. Outside of training or competition in high particulate air pollution, a mask that effectively filters particles may be beneficial. Summary ► Minimise exposure to air pollution during transportation to exercise facilities by closing vehicle windows, turning on air conditioning and using cabin air filters. RESPIRATORS AND FACE MASKS When exposures cannot be avoided, a face mask that reduces the inhalation of air pollutants can be considered. The effectiveness of a face mask is dependent on the pollutant, type of filter or adsorbent material, ability to correctly don the mask and quality of face seal. Not all users will be able to achieve an optimal seal with a single mask given varying facial characteristics (e.g. masks certified for adults may not fit children properly). The protection conferred by the cloth and surgical masks that have become commonplace during the COVID-19 pandemic is inconsistent given the inability to form a tight seal, allowing pollutants to flow through the path of least resistance via gaps in the face seal, rather than the filter itself.78 Hung A, et al. Br J Sports Med 2023;0:1–10. doi:10.1136/bjsports-2022-106161 Br J Sports Med: first published as 10.1136/bjsports-2022-106161 on 9 January 2023. Downloaded from http://bjsm.bmj.com/ on January 9, 2023 at The University of British Columbia Library. Protected by copyright. Consensus statement Surprisingly, research into the health and performance effects of masks in the context of air pollution is lacking. Langrish et al found a beneficial BP and autonomic effect from wearing an N95 face mask during a 2-hour walk. However, participants wore masks for 24 hours on the day before and the day of the study (for a total of 48 hours), limiting generalisability.79 In a recent double-blinded, randomised study, wearing an N95 face mask during a 2-hour walk had beneficial effects on lung function, airway oxidative stress and systemic inflammation, relative to a sham mask.80 Among children, wearing an N95 mask designed for paediatric populations while brisk walking was found to be comfortable and safe.81 In the only study investigating the use of carbon-infused masks to protect against O3, improvements in lung function were observed compared with a sham mask, but discomfort and breathing difficulties were also reported.82 Although recent meta-analyses found wearing an N95 face mask during exercise had minimal physiological and performance effects,83 they are not universally tolerated during exercise. Therefore, given the paucity of studies investigating the health and performance effects of wearing a face mask during moderate-to-vigorous intensity exercise in a highly polluted environment, we do not specifically recommend wearing a face mask during moderate-to-vigorous exercise. Nonetheless, athletes in high PM environments may consider the use of a properly fitted N95 face mask prior to and following training and competition to minimise the potential adverse effects of pre-exposure. Summary ► Athletes in environments characterised by high levels of PM may consider wearing a face mask that has been verified to remove ≥95% of airborne particles (i.e. N95, KN95, FFP2) when outside of training or competition. Cloth and surgical masks are not recommended given the variability in forming tight face seals, undermining their effectiveness. ► Face mask efficacy is dependent on: (1) wearing it correctly, (2) ensuring proper fit via a user seal check and (3) maintaining and replacing the mask after saturation. MEDICATIONS EIB is a respiratory disorder that is particularly prevalent in athletic populations, which is of concern, given that asthmatics may be prone to acute exacerbations brought on by air pollution.38 Common first-line and second-line medications include inhaled β2-agonists (e.g. salbutamol/albuterol, salmeterol), inhaled corticosteroids, anticholinergics and leukotriene receptor antagonists (e.g. montelukast).38 As air pollution contributes to EIB by exacerbating oxidative stress and inflammatory pathways, it has been hypothesised that these medications may be effective prophylactic medications. However, the available research is limited, and each medication likely has different effects and may work differently according to the pollutant. On the contrary, concerns have also been raised over asthma medications potentially proving counterproductive by facilitating the deposition of pollutants further down the bronchial tree via bronchodilation. Indeed, recent work in mice has demonstrated exacerbations in O3-induced respiratory inflammation with combined long-acting β2-agonists and glucocorticoid use.84 In two double- blinded, placebo- controlled trials involving competitive, non-asthmatic athletes exposed to O3, salbutamol did not attenuate the effects of O3 on lung function, symptoms or exercise performance.85 86 In a recent study exposing young adults with EIB to high DE concentrations, pre-exercise salbutamol use induced bronchodilation, reduced the work of breathing but did Hung A, et al. Br J Sports Med 2023;0:1–10. doi:10.1136/bjsports-2022-106161 not affect dyspnoea, microvascular or macrovascular function, or HR when compared with filtered air.87 88 Use of the long- acting β2-agonist salmeterol in adults with asthma exposed to SO2 improved decrements in pre-exercise to postexercise change in FEV1 and symptoms.89 Some studies do suggest that montelukast use, at least under well-controlled conditions, may be effective at attenuating SO2- and PM-induced bronchoconstriction, respiratory inflammation and endothelial dysfunction.90–92 Taken together, there is currently no evidence to suggest that asthma medications can aggravate the acute effects of air pollution exposure during exercise. Likewise, there is also no support asthmatics or increasing for using these medications in non- the dose in asthmatics exercising in high pollution conditions. Limited evidence suggests montelukast or salmeterol use may have vascular and respiratory benefits under certain conditions, but there is no evidence that these changes translate into ergogenic benefits. However, prior to the prescription of montelukast, a shared decision-making process must be undertaken to evaluate the risk-benefit trade-off, given montelukast’s risk for serious adverse neuropsychiatric events.93 In summary, patients with asthma or EIB should continue to use asthma medications as prescribed and avoid increasing medication doses prior to exercising in a polluted environment. As always, athletes must doping regulations remain in compliance with current anti- regarding any medication use (https://www.wada-ama.org/en/ prohibited-list). Summary ► Asthma medications do not appear to mitigate or aggravate the effects of air pollution exposure during exercise. ► Athletes with asthma and/or EIB should continue to phar- macologically control their conditions, with the dose and frequency as prescribed. ► Practitioners must remain aware and up to date with organisation-specific antidoping regulations. SUPPLEMENTS Much research examines the interaction between supplements and pollution, given that multiple air pollutants (e.g. PM, O3) exert their adverse cardiorespiratory effects by augmenting oxidative stress levels.94 However, relatively few studies have explored the potential role of antioxidant supplementation in modulating the health and performance effects of air pollution exposure during exercise; exercise is known to improve cardiorespiratory fitness levels by favourably influencing one’s antioxidant capacity, which may potentially offset the pro-oxidative effects of air pollution.94 In two ecological studies following healthy, amateur Dutch cyclists during the summer, antioxidant supplementation had a prophylactic effect against acute O3-induced decrements in lung function. In the first trial, ambient O3 levels were negatively associated with post-exercise FVC, FEV, and peak expiratory flow; 12 weeks of daily β-carotene, vitamin E and vitamin C (starting 1 week before measurements) modified this relationship.95 Findings were replicated in a second larger trial involving 38 cyclists: a 100 µg/m3 O3 (~51 ppb) exposure reduced FEV1 and FVC by 95 mL and 125 mL, respectively, and daily antioxidant supplementation attenuated these reductions.96 However, variability exists between studies, with one double-blinded trial finding no improvements in O3- induced respiratory inflammation or symptoms, despite a significant attenuation in lung function,97 and another reporting no protection whatsoever in terms of lung function and inflammation.98 In the only study 7 Br J Sports Med: first published as 10.1136/bjsports-2022-106161 on 9 January 2023. Downloaded from http://bjsm.bmj.com/ on January 9, 2023 at The University of British Columbia Library. Protected by copyright. Consensus statement examining performance effects, using antioxidant supplementation in trained runners did not affect average speed, HR, perceived exertion, or total time on an 8 km time trial performed in 100 ppb O3.99 The benefits of antioxidant supplementation are less clear in the context of TRAP. A one-time red-orange juice supplementation 2.5 hours prior to a Yo-Yo intermittent recovery test blunted TRAP-induced increases in postexercise HR, systolic BP, skeletal muscle damage and lipid peroxidation.100 However, despite authors reporting reductions in V̇ O2peak from TRAP exposure, no effect modification from the red-orange juice supplementation was observed.100 In summary, while recognising significant variability between studies, it appears that antioxidant dietary supplementation with 250–650 mg of vitamin C and 75–100 mg of vitamin E, and 25 mg of β-carotene for at least 1 week prior to exercising in high O3 environment may reduce O3 effects, especially in those with inadequate dietary intake. Summary ► For at least 1 week prior to exercising in an environment with high O3 levels, athletes may consider consuming 250–650 mg of vitamin C and 75–100 mg of vitamin E, and 25 mg of β-carotene. CONCLUSION Air pollution is of particular importance in the context of sport, with athletes generally inhaling larger doses of air pollutants in both acute and chronic settings. While the health, well-being and performance effects of exercising in air pollution have yet to be fully elucidated, the health effects of air pollution are well documented and substantial. Changes in national policies are necessary to create long-lasting changes, but evidence-informed and practical personal-level strategies offer athletes a major tool in reducing the exposure to and effects of air pollution. Based on the reviewed literature and the perspectives of the panel of authors, athletes may minimise air pollution exposures outside of training and competition by monitoring concentrations, exercising in the morning or in locations when seasonal events are less likely, minimising pre-exercise and intratransport exposures, wearing face masks and optimising antioxidant consumption. Athletes with asthma and/or EIB should continue to use medications as prescribed by their physicians. Consecutive multiday exposures to O3 prior to competition may also attenuate the pulmonary effects of O3 pollution. During the exercise, athletes should aim to minimise the total inhaled dose of air pollution and maximise distances from significant sources of air pollution (e.g. major traffic arteries). The contents of this statement should be interpreted in the context of its limitations. Foremost is the uncertainty and limited primary evidence base underscoring the provided recommendations. As expected, the literature search identified relatively few relevant research studies for each strategy, with some completely lacking high-quality, randomised controlled studies designed to specifically investigate their efficacy. Moreover, while systematic methodologies were employed during the literature search, a narrative approach was necessary due to the limited primary evidence base and methodological heterogeneity. To employ a truly personalised approach to mitigating the impact of air pollution in sport and exercise, further research investigating the different complex components of exercise (e.g. frequency, intensity, modality and duration) and air pollution (e.g. type, sources, concentrations and mixtures) is warranted. We did not identify any studies investigating the long- term 8 efficacy of any strategies on ‘hard’ clinical or performance endpoints either. There is also a distinct lack of diversity in the evidence base regarding sport type and competition level—most were performed in young, healthy adults employing a cycling, running or walking model. This statement was intended to raise public awareness of and provide guidance around a growing area of concern in the sport and exercise field. The benefits and harms of each strategy remain, to a degree, uncertain. Future well-designed randomised controlled trials are necessary to further validate the efficacy of each strategy in optimising the health and performance of athletes. In all situations, this statement should not supplant clinical judgement, and a shared, informed decision-making process should be undertaken prior to the adoption of any interventions. Author affiliations 1 School of Kinesiology, The University of British Columbia, Vancouver, British Columbia, Canada 2 Barcelona Institute for Global Health, Barcelona, Catalonia, Spain 3 Universitat Pompeu Fabra, Barcelona, Catalonia, Spain 4 Laboratoire Motricité Humaine Expertise Sport Santé, Université Côte d’Azur, Nice, France 5 International Collaboration on Repair Discoveries, Faculty of Medicine, The University of British Columbia, Vancouver, British Columbia, Canada 6 Athletics Canada, Ottawa, Ontario, Canada 7 Endurance Performance Research Group, School of Physical Education and Sport, University of São Paulo, São Paulo, Brazil 8 Canadian Sport Institute – Pacific, Victoria, British Columbia, Canada 9 Division of Sport & Exercise Medicine, Faculty of Medicine, The University of British Columbia, Vancouver, British Columbia, Canada Twitter Valerie Bougault @VBougault, Cameron Marshall Gee @CameronMGee and Michael Stephen Koehle @mskoehle Funding SK was funded by a Marie Skłodowska-Curie Individual Fellowship by the European Commission (840513). ISGlobal acknowledges support from the Spanish Ministry of Science and Innovation through the ’Centro de Excelencia Severo Ochoa 2019-2023’ Program (CEX2018-000806-S), and the Generalitat de Catalunya through the CERCA Program. Competing interests None declared. Patient consent for publication Not applicable. Provenance and peer review Not commissioned; externally peer reviewed. Supplemental material This content has been supplied by the author(s). It has not been vetted by BMJ Publishing Group Limited (BMJ) and may not have been peer-reviewed. Any opinions or recommendations discussed are solely those of the author(s) and are not endorsed by BMJ. BMJ disclaims all liability and responsibility arising from any reliance placed on the content. Where the content includes any translated material, BMJ does not warrant the accuracy and reliability of the translations (including but not limited to local regulations, clinical guidelines, terminology, drug names and drug dosages), and is not responsible for any error and/or omissions arising from translation and adaptation or otherwise. ORCID iDs Andy Hung http://orcid.org/0000-0002-6905-6323 Sarah Koch http://orcid.org/0000-0001-9461-8407 Valerie Bougault http://orcid.org/0000-0002-2258-6562 Cameron Marshall Gee http://orcid.org/0000-0003-0333-1836 Michael Stephen Koehle http://orcid.org/0000-0001-7026-8422 REFERENCES 1 Bull FC, Al-Ansari SS, Biddle S, et al. World Health organization 2020 guidelines on physical activity and sedentary behaviour. Br J Sports Med 2020;54:1451–62. 2 Bernard P, Chevance G, Kingsbury C, et al. Climate change, physical activity and sport: a systematic review. Sports Med 2021;51:1041–59. 3 Giles LV, Koehle MS. The health effects of exercising in air pollution. Sports Med 2014;44:223–49. 4 Hung A, Nelson H, Koehle MS. The acute effects of exercising in air pollution: a systematic review of randomized controlled trials. Sports Med 2022;52:139–64. 5 Tainio M, Jovanovic Andersen Z, Nieuwenhuijsen MJ, et al. Air pollution, physical activity and health: a mapping review of the evidence. Environ Int 2021;147:105954. Hung A, et al. Br J Sports Med 2023;0:1–10. doi:10.1136/bjsports-2022-106161 Br J Sports Med: first published as 10.1136/bjsports-2022-106161 on 9 January 2023. Downloaded from http://bjsm.bmj.com/ on January 9, 2023 at The University of British Columbia Library. Protected by copyright. Consensus statement 6 DeFlorio-Barker S, Lobdell DT, Stone SL, et al. Acute effects of short-term exposure to air pollution while being physically active, the potential for modification: a review of the literature. Prev Med 2020;139:106195. 7 Dominici F, Schwartz J, Di Q, et al. Assessing adverse health effects of long-term exposure to low levels of ambient air pollution: phase 1, 2019. 8 Brauer M, Brook JR, Christidis T, et al. Mortality–Air pollution associations in Low- Exposure environments (maple): phase 1, 2019. 9 Brunekreef B, Strak M, Chen J, et al. Mortality and morbidity effects of long-term exposure to low-level PM2.5, bc, NO2, and O3: an analysis of European cohorts in the ELAPSE project. Boston, MA, 2021. 10 World Health Organization. Who global air quality guidelines: particulate matter (PM2.5 and PM10), ozone, nitrogen dioxide, sulfur dioxide and carbon monoxide, 2021. 11 Ross WJ, Orr M. Predicting climate impacts to the Olympic Games and FIFA Men’s World Cups from 2022 to 2032. Sport Soc 2022;25:867–88. 12 Dominici F, Peng RD, Barr CD, et al. Protecting human health from air pollution: shifting from a single-pollutant to a multipollutant approach. Epidemiology 2010;21:187–94. 13 U.S. Environmental Protection Agency. Integrated science assessment (Isa) for particulate matter (final report, Dec 2019. Washington, DC, 2019. 14 U.S. Environmental Protection Agency. Integrated science assessment (Isa) for ozone and related photochemical oxidants (final report, Apr 2020, 2020. 15 Health Effects Institute. Ozone exposure | state of global air 2020, 2020. Available: https://www.stateofglobalair.org/air/ozone [Accessed 08 Jun 2022]. 16 Koenig JQ. Health effects of ambient air pollution. 1st ed. Boston, MA: Springer, 2000. 17 U.S. Environmental Protection Agency. Nitrogen oxides (NOX), why and how they are controlled, 1999. 18 Health Canada. Human health risk assessment for sulphur dioxide, 2016. 19 U.S. Agency for Toxic Substances and Disease Registry. Medical management guidelines for sulfur dioxide, 2014. Available: https://wwwn.cdc.gov/TSP/MMG/ MMGDetails.aspx?mmgid=249&toxid=46 [Accessed 7 Feb 2022]. 20 Linn WS, Avol EL, Peng RC, et al. Replicated dose-response study of sulfur dioxide effects in normal, atopic, and asthmatic volunteers. Am Rev Respir Dis 1987;136:1127–35. 21 Environment and Climate Change Canada. Common air pollutants: carbon monoxide, 2016. Available: https://www.canada.ca/en/environment-climate-change/ services/air-pollution/pollutants/common-contaminants/carbon-monoxide.html [Accessed 9 Dec 2021]. 22 Adir Y, Merdler A, Ben Haim S, et al. Effects of exposure to low concentrations of carbon monoxide on exercise performance and myocardial perfusion in young healthy men. Occup Environ Med 1999;56:535–8. 23 Ekblom B, Huot R, Stein EM, et al. Effect of changes in arterial oxygen content on circulation and physical performance. J Appl Physiol 1975;39:71–5. 24 Raub JA, Benignus VA. Carbon monoxide and the nervous system. Neurosci Biobehav Rev 2002;26:925–40. 25 U.S. Environmental Protection Agency. What are volatile organic compounds (VOCs). Available: https://www.epa.gov/indoor-air-quality-iaq/what-are-volatile-organic- compounds-vocs [Accessed 7 Feb 2022]. 26 U.S. Environmental Protection Agency. Volatile Organic Compounds’ Impact on Indoor Air Quality. Available: https://www.epa.gov/indoor-air-quality-iaq/volatile- organic-compounds-impact-indoor-air-quality [Accessed 7 Feb 2022]. 27 Furtaw EJ. An overview of human exposure modeling activities at the USEPA’s national exposure research laboratory. Toxicol Ind Health 2001;17:302–14. 28 Cutrufello PT, Smoliga JM, Rundell KW. Small things make a big difference: particulate matter and exercise. Sports Med 2012;42:1041–58. 29 Kesavanathan J, Swift DL. Human nasal passage particle deposition: the effect of particle size, flow rate, and anatomical factors. Aerosol Science and Technology 1998;28:457–63. 30 Daigle CC, Chalupa DC, Gibb FR, et al. Ultrafine particle deposition in humans during rest and exercise. Inhal Toxicol 2003;15:539–52. 31 Gee CM, Eves ND, Sheel AW, et al. How does cervical spinal cord injury impact the cardiopulmonary response to exercise? Respir Physiol Neurobiol 2021;293:103714. 32 McConnell R, Berhane K, Gilliland F, et al. Asthma in exercising children exposed to ozone: a cohort study. The Lancet 2002;359:386–91. 33 Andersen ZJ, de Nazelle A, Mendez MA, et al. A study of the combined effects of physical activity and air pollution on mortality in elderly urban residents: the Danish diet, cancer, and health cohort. Environ Health Perspect 2015;123:557–63. 34 Fisher JE, Loft S, Ulrik CS, et al. Physical activity, air pollution, and the risk of asthma and chronic obstructive pulmonary disease. Am J Respir Crit Care Med 2016;194:855–65. 35 Kubesch NJ, Therming Jørgensen J, Hoffmann B, et al. Effects of leisure-time and transport-related physical activities on the risk of incident and recurrent myocardial infarction and interaction with traffic-related air pollution: a cohort study. J Am Heart Assoc 2018;7:e009554. , Thach T-Q, et al. Does regular exercise protect against air 36 Wong C-M, Ou C-Q pollution-a ssociated mortality? Prev Med 2007;44:386–92. Hung A, et al. Br J Sports Med 2023;0:1–10. doi:10.1136/bjsports-2022-106161 37 Lamichhane DK, Leem JH, Kim HC. Associations between ambient particulate matter and nitrogen dioxide and chronic obstructive pulmonary diseases in adults and effect modification by demographic and lifestyle factors. Int J Environ Res Public Health 2018;15:363. 38 Parsons JP, Hallstrand TS, Mastronarde JG, et al. A