Biooooxxx PDF - DNA Structure and Function
Document Details
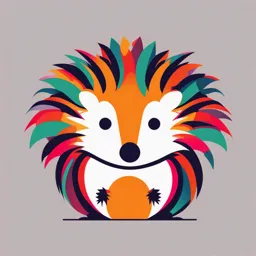
Uploaded by BestNarwhal
Tags
Summary
This document provides a comprehensive overview of DNA structure and function, including genetic information, DNA replication, and its role in protein synthesis. It covers various aspects like the double helix structure, base pairing, and the organization of DNA in eukaryotes. The text also discusses different levels of DNA packaging, chromatin remodeling, and its dynamic nature. Suitable for undergraduate-level biology study and research.
Full Transcript
SUMMARY 1- Genetic Information: DNA carries genetic information in the form of a linear sequence of nucleotides (building blocks). These nucleotides determine the genetic code. Double Helix Structure: DNA exists as a double helix, formed by two long strands of nucleotides that twist around...
SUMMARY 1- Genetic Information: DNA carries genetic information in the form of a linear sequence of nucleotides (building blocks). These nucleotides determine the genetic code. Double Helix Structure: DNA exists as a double helix, formed by two long strands of nucleotides that twist around each other. These strands are complementary and antiparallel, meaning they run in opposite directions. Base Pairing: The two strands are held together by hydrogen bonds between specific pairs of nucleotides. Guanine (G) always pairs with cytosine (C), and adenine (A) pairs with thymine (T). Antiparallel Nature: The two DNA strands are antiparallel, meaning one strand runs in the 5’ to 3’ direction, while the other runs in the 3’ to 5’ direction. This orientation is crucial for DNA replication and function. DNA Replication: During DNA duplication, one strand of DNA serves as a template for synthesizing a complementary strand. This ensures that genetic information is accurately passed on during cell division. Genome: The complete set of genetic information in an organism’s DNA is referred to as its genome. This genome contains all the instructions required for the organism to synthesize every RNA molecule and protein it will need throughout its life. Role of DNA in Protein Synthesis: The DNA genome provides the blueprint for creating RNA molecules, which are then translated into proteins, essential for cellular functions and development. Location in Eukaryotes: In eukaryotic cells (cells with a nucleus), the DNA is enclosed within the nucleus, a membrane-bound compartment. This compartmentalization protects the DNA and regulates the processes of gene expression, replication, and repair. Nuclear Function: The nucleus serves as the control center for managing genetic information, allowing access to specific genes when needed for protein production or during DNA replication while safeguarding the overall integrity of the genome. SUMMARY 2- Gene Definition: A gene is a sequence of nucleotides in a DNA molecule that functions as a unit for producing proteins, structural RNA, or catalytic/regulatory RNA molecules. Protein-Coding Genes in Eukaryotes: In eukaryotes, genes that code for proteins typically consist of alternating introns (non-coding regions) and exons (coding regions), along with regulatory DNA regions that control gene expression. Chromosome Structure: A chromosome consists of a single, extremely long DNA molecule that contains a linear array of many genes. These genes are tightly bound to various proteins to form chromosomal structure. Human Genome: The human genome is composed of 3.2 billion (3.2 × 10^9) DNA nucleotide pairs, organized into 22 autosomes (present in two copies each) and 2 sex chromosomes. Only a small fraction of this DNA codes for proteins or functional RNA molecules. Non-Coding DNA Functions: Apart from coding regions, chromosomal DNA contains important sequences for replication and cell division: Replication Origins: Sites where DNA replication begins. Telomeres: Protective sequences at the ends of chromosomes that facilitate complete replication. Centromeres: Attach the sister chromatids to the mitotic spindle during cell division, ensuring accurate segregation to daughter cells. DNA Packaging with Histones: In eukaryotes, DNA is tightly bound to histone proteins, which together form repeated DNA–protein particles called nucleosomes. This binding ensures efficient packing and protection of the genetic material. Nucleosome Structure: Nucleosomes consist of an octameric core of histone proteins around which the DNA double helix is wrapped. These nucleosomes are spaced about 200 nucleotide pairs apart, creating a repeating structure along the DNA. Chromatin Fiber Formation: Nucleosomes are further packed into a more compact 30-nm chromatin fiber, aided by histone H1 molecules. This compaction helps in the organization of large amounts of DNA into the nucleus. Chromatin Dynamics: Despite being compact, chromatin must remain dynamic to allow gene expression, replication, and repair. Spontaneous unwrapping and rewrapping of DNA within nucleosomes occur, allowing temporary access to specific DNA regions. Chromatin Remodeling Complexes: Cells use ATP-driven chromatin remodeling complexes to actively change the structure of chromatin. These complexes target specific regions of the chromatin and work at appropriate times to control access to the DNA. Role of Histone Chaperones: Remodeling complexes collaborate with histone chaperones to reposition, replace, or remove nucleosome cores, allowing exposure of underlying DNA for transcription, replication, or repair. Regulation of DNA Accessibility: This highly dynamic system of chromatin remodeling allows cells to regulate access to genetic material, facilitating controlled gene expression and cell function in response to environmental or developmental signals. SUMMARY 3- Uniform DNA Assembly into Nucleosomes: In eukaryotic chromosomes, DNA is consistently assembled into nucleosomes, which are fundamental units of chromatin structure. Despite this uniformity, chromatin can adopt a variety of structural forms based on chemical modifications to histones. Histone Modifications: A diverse range of reversible covalent modifications to the four core histones in nucleosomes creates this variety in chromatin structure. These modifications include: Mono-, di-, and trimethylation of lysine side chains on histones. Acetylation of lysines, which is mutually exclusive with methylation on the same lysine residues. These chemical changes alter the interactions between nucleosomes and various chromatin-associated proteins. Modification Combinations as Chromatin Marks: Specific combinations of histone modifications, also known as “marks,” are applied to nucleosomes. These marks serve as signals that govern nucleosome interactions with proteins, regulating chromatin function and gene expression. Reader Proteins and Chromatin Interaction: Specialized proteins called “reader” proteins, which are part of larger protein complexes, bind to the modified nucleosomes. These reader proteins recognize the specific histone marks and recruit additional proteins that carry out various tasks, such as remodeling chromatin, regulating gene expression, or influencing DNA repair. Reader-Writer Complexes: Some of these reader protein complexes also contain enzymes known as “writers” that add further histone modifications. For example, a histone lysine methylase can recognize and methylate specific lysines, propagating the same mark the reader protein recognizes. This reader-writer system can facilitate the spread of particular chromatin structures along a chromosome. Formation of Heterochromatin: This reader-writer mechanism is thought to contribute to the formation of condensed chromatin, specifically heterochromatin. Heterochromatin is tightly packed and gene-poor, often silencing any genes within it. It is typically located around centromeres, telomeres, and other specific chromosomal regions. Role of Heterochromatin in Gene Silencing: The compact structure of heterochromatin prevents access to the DNA within, effectively silencing genes located in these regions. This silencing is important for maintaining genome stability and regulating gene expression. Position Effect Variegation: This phenomenon provides strong evidence that condensed chromatin states, such as heterochromatin, can be inherited across cell generations. In position effect variegation, a gene’s expression is affected by its proximity to heterochromatin, demonstrating that chromatin structure can spread and be maintained across divisions. Centromeric Chromatin Maintenance: A similar inheritance mechanism preserves the specialized chromatin structures at centromeres, which are critical for proper chromosome segregation during cell division. The maintenance of this specialized chromatin ensures centromeres remain functional across generations. Epigenetic Cell Memory: The propagation of specific chromatin structures across cell generations enables an epigenetic memory system. This epigenetic mechanism allows cells to “remember” their specific states (e.g., differentiation states) without altering the underlying DNA sequence. This is crucial for complex multicellular organisms, where different cell types must retain their identity and function over time. Role in Multicellular Organisms: This epigenetic memory system is essential for maintaining distinct cell states and functions, allowing multicellular organisms to develop and sustain complex tissues and organs by preserving the specialized functions of different cell types across generations. SUMMARY 4- Nucleotide Sequence Comparisons in Genomes: Comparing the nucleotide sequences of modern genomes has greatly enhanced our understanding of gene and genome evolution, revealing patterns of genetic change over time. High Fidelity of DNA Replication and Repair: DNA replication and repair processes are highly accurate, allowing only about one nucleotide out of a thousand to change every million years in a typical eukaryotic lineage. This low mutation rate means that evolutionary changes in nucleotide sequences occur very slowly over time. Human and Chimpanzee Genome Comparison: Humans and chimpanzees diverged around 6 million years ago, and comparisons of their genomes reveal very few differences. Their genes are nearly identical, and the order of genes on their chromosomes is also almost the same. Although some segmental duplications (DNA duplications) and segmental deletions (DNA losses) have occurred, even the positions of transposable elements (noncoding DNA that moves around in the genome) have remained largely unchanged. Human and Mouse Genome Comparison: Comparing genomes of more distantly related species, such as humans and mice (separated by about 80 million years), shows far more changes. Essential nucleotide sequences, especially in regulatory regions and coding sequences (exons), have been preserved through purifying selection (the elimination of harmful mutations), highlighting the evolutionary pressure to maintain critical functions. In contrast, nonessential sequences, like much of the DNA in introns, have diverged so much that their shared ancestry is no longer recognizable. Role of Purifying Selection: The action of purifying selection makes comparing the genomes of multiple related species a powerful tool for identifying DNA sequences with important functions. By maintaining essential sequences over time, purifying selection highlights regions of the genome that play critical roles in biology. Conserved DNA in the Human Genome: Approximately 5% of the human genome has been conserved due to purifying selection. Despite being conserved across species, much of this DNA has unknown functions. Tens of thousands of multispecies conserved sequences remain mysterious, and future research is expected to reveal their roles in vertebrate biology. Gene Family Expansion and Genetic Complexity: One of the major drivers of genetic complexity in modern organisms has been the expansion of ancient gene families. Through DNA duplication followed by sequence divergence, new genetic functions have evolved, providing a major source of genetic innovation over evolutionary time. Genetic Variation in Humans: On a more recent evolutionary scale, the genomes of individual humans vary from one another due to: Single-nucleotide polymorphisms (SNPs), which are single-base changes in the DNA. Copy number variations (CNVs), which involve inherited gains and losses of DNA sequences that lead to differences in gene copy number between individuals. These variations contribute to genetic diversity in humans and have important implications for human biology and medicine. Impact on Medicine and Human Biology: Understanding the effects of genetic variations like SNPs and CNVs will provide valuable insights into human biology and disease. This knowledge can lead to advancements in medical treatments and a deeper understanding of human health, evolution, and diversity. Future Research: Continued research into conserved sequences and genetic variations will expand our understanding of vertebrate biology and the genetic differences that underlie human traits and diseases. This could lead to breakthroughs in personalized medicine and insights into the evolutionary processes that shape genomes. SUMMARY 5- Interphase Chromosome Decondensation: During interphase, chromosomes are generally decondensed, making their detailed structure difficult to observe. Despite this, specialized chromosomes such as lampbrush chromosomes (in vertebrate oocytes) and polytene chromosomes (in insect secretory cells) serve as exceptions and provide insights into chromatin organization during this phase. Chromatin Loop Domains: Studies on lampbrush and polytene chromosomes suggest that each long DNA molecule in a chromosome is divided into distinct chromatin loop domains. These loops represent sections of the DNA that are compacted through additional folding. When genes within a loop are activated for expression, the loop temporarily unfolds, granting the cell’s transcription machinery access to the DNA. Chromosome Territories in the Nucleus: Interphase chromosomes occupy discrete territories within the cell nucleus, meaning they are not extensively intertwined with each other. This organization prevents entanglement and facilitates proper gene regulation and DNA functions. Euchromatin and Heterochromatin in Interphase: Euchromatin: The majority of interphase chromatin is euchromatin, which consists of relatively loosely packed nucleosomes. Euchromatin is more accessible for transcription machinery when needed, although, when inactive, it remains compacted. Heterochromatin: Within euchromatin, there are stretches of heterochromatin, where nucleosomes are more tightly packed. This compacted state renders the DNA largely inaccessible for gene expression. Heterochromatin plays a critical role in gene silencing and structural chromosome integrity. Forms of Heterochromatin: Heterochromatin exists in various forms, including large blocks around centromeres and near telomeres. These regions are essential for maintaining chromosomal stability and for the regulation of gene expression. Heterochromatin can also be found at other chromosomal positions, where it helps regulate developmentally important genes, serving as a mechanism for controlling gene activity during different stages of development. Dynamic Nuclear Organization: The interior of the nucleus is highly dynamic. Heterochromatin is often positioned near the nuclear envelope, while chromatin loops can extend out of their defined chromosome territories when genes are highly expressed. This movement and organization reflect the presence of nuclear subcompartments, specialized regions within the nucleus where specific biochemical reactions occur. These regions enhance the efficiency of certain reactions by concentrating relevant proteins and RNAs. Formation of Nuclear Subcompartments: Nuclear subcompartments, such as the nucleolus and Cajal bodies, are formed through self-assembly of their components. These discrete nuclear structures help coordinate specific cellular processes such as ribosome synthesis and RNA splicing. In some cases, components of nuclear subcompartments can be tethered to fixed structures like the nuclear envelope, adding to the organization and functionality of the nucleus. Chromatin Condensation During Mitosis: During mitosis, gene expression is largely shut down, and all chromosomes undergo a significant condensation process. This transition begins in early M phase to ensure the efficient packaging of the two DNA molecules in each replicated chromosome as two separately folded chromatids. The condensation process involves histone modifications, which facilitate the tighter packing of chromatin. These modifications play a role in reorganizing the DNA into a more condensed state. Further Chromosome Condensation: In addition to histone modifications, additional proteins are required for the full condensation of chromosomes. This process reduces the overall length of the DNA by a factor of ten compared to its interphase state, ensuring chromosomes are compact enough for proper segregation during cell division. Orderly Chromosome Segregation: The full condensation and folding of chromatids during mitosis is critical for the orderly segregation of chromosomes into daughter cells. This highly regulated process ensures that each daughter cell receives the correct number of chromosomes during division.