Biomembranes & Cell Surface PDF
Document Details
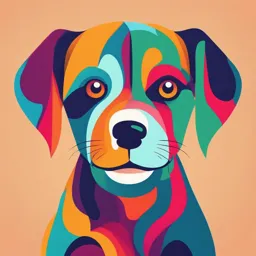
Uploaded by WellBredSmokyQuartz7678
Ramnarain Ruia Autonomous College
Tags
Summary
This document provides an overview of biomembranes and cell surfaces, covering topics such as the fluid mosaic model, chemical composition of membranes (including lipids, proteins, and carbohydrates), and various transport mechanisms. It also discusses the role of cholesterol and lipid rafts in membrane fluidity and their relevance in different biological contexts.
Full Transcript
RUSBCH501 :-Membrane Biochemistry & Cancer Biology Unit:1 Biomembrane’s & Cell Surface SYLLABUS :- 1.1 Overview of membrane functions 1.2 Fluid mosaic model 1.3 Chemical Composition of Membranes 1.3.1 Membrane lipids (Phosph...
RUSBCH501 :-Membrane Biochemistry & Cancer Biology Unit:1 Biomembrane’s & Cell Surface SYLLABUS :- 1.1 Overview of membrane functions 1.2 Fluid mosaic model 1.3 Chemical Composition of Membranes 1.3.1 Membrane lipids (Phospholipids, Glycolipids, sterols (Cholesterol), Lipid rafts 1.3.2 Membrane proteins - Classification- Peripheral Proteins, Integral Membrane Proteins and Lipid- Anchored proteins Peripheral Proteins- Spectrin on RBC Integral Membrane Proteins- Glycophorin A on RBC Lipid-Anchored proteins- Role of GPI anchored protein in blood grouping 1.3.3 Membrane carbohydrate – Role of membrane glycoproteins WHAT IS CELL MEMBRANE ? The cell membrane (also called the plasma membrane or plasmalemma) is a biological membrane separating the interior of a cell from the outside environment It appears in thin sections with the electron microscope as a triple-layered structure about 7.5–10 nanometers thick The cell membrane is flexible and allows a unicellular organism to move FUNCTION OF CELL MEMBRANE :- Maintain a steady environment: Homeostasis Protective barrier Regulate transport in & out of cell (selectively permeable) Small lipid-soluble molecules, e.g. oxygen and carbon dioxide can pass easily Water can freely move cross the membrane Ions and large molecules cannot cross without assistance Allow cell recognition Provide anchoring sites for filaments of cytoskeleton Provide a binding site for enzymes Interlocking surfaces bind cells together (junctions) Contains the cytoplasm (fluid in cell) TYPE OF TRANSPORT :- Passive transport: Simple diffusion Facilitated diffusion: channel, carrier Osmosis Active transport: Pump Bulk membrane transport: Endocytosis: pinocytosis, phagocytosis, receptor mediated Exocytosis FLUID MOSAIC MODEL :- In 1972 SJ Singer and GL Nicolson proposed fluid mosaic model. Fluid – Individual phospholipids and some proteins can move sideways (laterally) in each layer- therefore FLUID Mosaic – Range of different proteins resting on the surface on through the phospholipid layer gives it a mosaic appearance. CHEMICAL COMPOSITION OF PLASMA MEMBRANE :- ❖ POINTS TO THINK… Carnitine shuttle Represent a mechanism by which long chain fatty acids, which are impermeable to the mitochondrial membrane are transported into the mitochondrial matrix for the purpose Beta- oxidation and energy production. Exocytosis (Ab production) In exocytosis, membrane-bound secretory vesicles are carried to the cell membrane, where they dock and fuse at porosomes and their contents (i.e. water soluble molecules) are secreted into the extracellular environment. This secretion is possible because the vesicle transiently fuses with the plasma membrane. Why these oligosaccharide are called Blood Group Ag? The type of oligosaccharides present on the surface of the red blood cell determine a person blood type if only O-type antigen is present, the blood type is O, if only Antigen A or B is found, the blood A or B, respectively, and if both A and B antigens are the blood type is AB Chemical Composition of Membranes Chemical Composition Of Plasma Membrane Lipids and proteins are the two major components of all membrane. Carbohydrates are present in outer leaflets. But in inner leaflets, do not have a significant role. Carbohydrates bound either to proteins as constituents of glycoproteins or bound to lipids as constituents of glycolipids. Lipids are the Major Components:- 3 major components of eukaryotic membrane are: Glycerophospholipids Sphingolipids Cholesterol Membrane Lipids :- ❖ Glycerophospholipids :- Glycerophospholipids have a glycerol molecule with a phosphate esterified at the ɑ-carbon. Two long chain fatty acids esterifies to the remaining carbon atoms. Structure of major alcohols esterified to phosphatidic acid to form the glycerophosphospholipid. For ex:- if alcohol is choline, molecules is called phosphotidylcholine and if serine, then is called phosphotidylserine. Phosphotidyl group Glycerophospholipids contain two fatty acyl groups esterified to C 1 and C 2 of glycerol. A saturated fatty acid is usually found on C 1 and unsaturated fatty acid on C 2. The presence of unsaturated fatty acids has a marked effect on the fluidity of the membrane. Phosphotidylcholine and phosphotidylethanolamine are the most common glycerophospholipids. Ether phospholipids contain an alkyl group (alkyl acyl glycerophospholipid ) termed as plasmlogens. Plasmlogens containing ethanolamine or choline plasmlogens esterified to the phosphate are abundant in nervous tissue and heart. High levels of ether linked lipids in plasma membrane of every metastatic cancer cells have been reported. Suggested a role for the lipids in the invasive properties of these cells. ❖ Sphingolipids :- The amino alcohols sphingosine and dihydrosphingosine are the basis for the sphingolipids. Ceramide have a saturated or unsaturated long chain fatty acyl group in amide linkage with the amino group of sphingosine. The sphingomyelins, the most abundant sphingolipids in mammalian tissues, have phosphorylcholine esterified at C1. Glycosphingolipids contain a sugar linked by a ẞ-glycosidic bond to C1OH group of a ceramide. A subgroup of cerebroside which contain either glucose or galactose. Galactocerebroside predominate in brain and nervous tissue, whereas glucocerebroside occur in small quantities of non neuronal tissues. The plasma membrane of animal cells contains 4 major phospholipids, such as –phosphotidylcholine, phosphotidylserine, phosphotidylethanolamine and sphingomyelin. At neutral pH, the polar head group may have no net charge, or it may have net negative charge. ❖ Cholesterol :- Cholesterol is the third major lipid in membrane Cholesterol is a compact rigid hydrophobic molecule. It has a polar hydroxyl group at C3. Cholesterol alters the fluidity of membranes Mobility of lipid components in membrane :- Lipid bi-layer not rigid and static structure. Lipid molecules can rotate freely around their long axis and diffuse laterally within each leaflets. All phospholipids of plasma membrane are mobile, they are not fixed. They are moving laterally. The transition of a lipid molecule from one leaflet to the other is called transverse diffusion. Abnormalities of Cell Membrane Fluidity in Disease :- A major factor in the fluidity of the membrane in higher organisms and mammals in the presence of cholesterol. In case of spur cell anemia, erythrocyte membrane of individuals have increased cholesterol content & a spiny shaped. Cells are destroyed prematurely in the spleen. This condition occurs in severe liver disease such as alcoholic cirrhosis. Increase intracellular membrane cholesterol which affect their fluidity. The intoxicating effect of ethanol on the nervous system is probably due to modification of membrane fluidity, altering membrane receptors and ions channels. Lipid Rafts :- Simons and van Meer (1988) suggested existence of microdomains or “rafts” in plasma membrane of epithelial cells Original concept of rafts was used to explain transport of cholesterol from the trans Golgi network to the plasma membrane. Jacobson & Dietrich, 1999 discussed the existence of rafts and classified these into three, viz caveolae, glycosphingolipid enriched membranes (GEM),and polyphospho inositol rich rafts. At the 2006 Keystone Symposium of Lipid Rafts and Cell Function, lipid rafts were defined as "small (10-200nm), heterogeneous, highly dynamic, sterol- and sphingolipid-enriched domains that compartmentalize cellular processes" Lipid rafts are small (10-200nm), heterogeneous, highly dynamic, sterol and sphingolipid-enriched domains that compartmentalize cellular processes. Lipid rafts are membrane micro-domains enriched in sphingolipids,cholesterol and certain lipid- linked proteins. Outer leaflet : ceramide and glycosphogilipids with long chain fatty acids → thicker Inner leaflet ↑ saturated fatty acids → closed packing Micro-domains known as lipid rafts contain distinctly organized bilayer structures Enriched in sphingolipids and cholesterols Biological membranes are actually mosaic of different microdomains Structure of Rafts :- The fatty-acid chains of lipids within the rafts tend to be extended and so more tightly packed, creating domains with higher order. It is therefore thought that rafts exist in a separate ordered phase that floats in a sea of poorly ordered lipids. Glycosphingolipids, and other lipids with long, straight acyl chains are preferentially incorporated into the rafts. Two types of lipid rafts :- (1) Planar lipid rafts (non-caveolar, or glycolipid, rafts): Planar rafts are continuous with plane of the plasma membrane: Planar rafts contain flotillin proteins and are found in neurons where caveolae are absent. Both types have similar lipid composition (enriched in cholesterol and sphingolipids). (2) Caveolae: Caveolae are flask shaped invaginations of the plasma membrane that contain caveolin proteins: Caveolins are widely expressed in the brain, micro-vessels of the nervous system, endothelial cells, astrocytes, oligodendrocytes, Schwann cells, dorsal root ganglia and hippocampal neurons. Both flotillins and caveolins have the ability to recruit signaling molecules into lipid rafts, thus playing an important role in neurotransmitter signal transduction. Caveoline cholesterol binding integral membrane protein Forces bilayer to curve inwards forming caveolae Functions : membrane trafficking, signal transduction How do rafts function? Membrane is able to laterally segregate its constituents. This capability is based on dynamic liquid-liquid immiscibility and underlies the raft concept of membrane subcompartmentalization. Example in order to segregate and concentrate specific protein and to facilitate their activity, proteins are activated when several rafts fuse together or ligands binding occurs which favors fusion of rafts Lipid rafts are fluctuating nanoscale assemblies of sphingolipid, cholesterol, and proteins that can be stabilized to coalesce, forming platforms that function in membrane signaling and trafficking. Role of Cholesterol in Membranes and Rafts :- Abundant component of the plasma membranes of eukaryotic cells Plays an essential role in maintaining membrane integrity and fluidity. Critical for liquid-ordered raft/caveolae formation by serving as a spacer between the hydrocarbon chains of sphingolipids. Alterations in the its content in cells modifies the properties of these domains. Depletion of cholesterol from the plasma membrane causes disruption of rafts/caveolae and release of raft/caveolae constituents into a nonraft/caveola membrane, which renders them nonfunctional. Cholesterol is crucial for maintaining intact raft/caveola structure and function Key in Neural Functioning Lipid rafts are cholesterol-rich plasma membrane microdomains that regulate a diverse range of cellular functions. Rich in cholesterol and sphingolipids (high melting lipids). Important for neuronal cell adhesion, axon guidance and synaptic transmission. Crucial for neural development / function. Many diseases such as Alzheimer's, Huntington's, Parkinson's disease,AIDS etc are related to lipid rafts. Membrane Carbohydrate :- Carbohydrates are the third major component of plasma membranes. The extracellular surface of the cell membrane is decorated with carbohydrate groups attached to lipids and proteins. These carbohydrate chains may consist of 2-60 monosaccharide units and can be either straight or branched. Carbohydrates are added to lipids and proteins by a process called glycosylation, and are called glycolipids or glycoproteins These short carbohydrates or oligosaccharides are usually chains of 15 or fewer sugar molecules. Oligosaccharides give a cell identity (i.e., distinguishing "self" from "nonself") and are the distinguishing factor in human blood types and transplant rejection. Along with membrane proteins, these carbohydrates form distinctive cellular markers, that allow cells to recognize each other. These markers are very important in the immune system, allowing immune cells to differentiate between body cells, which they shouldn’t attack, and foreign cells or tissues, which they should. Distribution Of Membrane Carbohydrate :- The distribution of carbohydrates in the cell membrane is asymmetric. The extracellular face of the membrane is in contact with the extracellular fluid. The extracellular side of the membrane contains oligosaccharides that distinguish the cell as "self." It also contains the end of integral proteins that interact with signals from other cells and sense the extracellular environment. The inner membrane is in contact with the contents of the cell and tends to have few carbohydrates. Instead, this intracellular side of the membrane anchors to the cytoskeleton and contains the end of integral proteins that relay signals received on the external side. Role of Membrane Carbohydrates Cell-cell recognition is a cell’s ability to distinguish one type of neighboring cells from another. Cells recognize other cells by binding to molecules, often containing carbohydrates, on the extracellular surface of the plasma membrane. Membrane carbohydrate usually plays an important role in cell-cell recognition. They are usually short, branched chains of fewer than 15 sugar units. Some are covalently bonded to lipids, forming molecules called glycolipids. Most are covalently bonded to proteins, which are thereby glycoproteins. The carbohydrates on the extracellular side of the plasma membrane vary from species to species, among individuals of the same species, and even from one cell type to another in a single individual. Blood groups are determined by cell surface carbohydrates of erythrocytes, and they also have the ability to trigger immunological responses. They are used to identify a type of cell, if exploited by virus, then virus gains the entry. After an infection, endothelial cells near the injured tissue expose a type of proteins, known as selectins, in their plasma membranes. They recognize and bind carbohydrates of the plasma membrane of lymphocytes that go through the bloodstream. In this way, lymphocytes get attached to the blood vessel walls, can cross the endothelium and move to the infection focus. Carbohydrates as recognition molecules are also important during embryonic development. Carbohydrates of the plasma membrane are major recognition and attaching sites for pathogens during infection. The glycocalyx (carbohydrate-enriched coating) also has important functions in humans. It allows cells on the inside of blood vessels to withstand the strong flow of liquid across their surfaces. It protects microvilli in the gut, which absorb nutrients, and the glycocalyx even aids in the breakdown of food for this absorption by holding digestive enzymes in its coat. Certain plasma transport proteins, hormones, and enzymes are glycoproteins, and in these molecules, carbohydrate is important to physiological activity Membrane Proteins :- A membrane protein is a protein molecule that is attached to, or associated with the membrane of a cell or an organelle. It is any protein that is embedded in the plasma membrane of the cell. They do not anchored in one place but tend to float within the phospholipids bilayer. More than half of all proteins interact with membranes. Proteins can be associated with membranes in several different ways Membrane proteins have asymmetric orientations in membranes Proteins have very slow rates of movement –slower than lipids Membrane protein’s soluble form is critical to function (e.g. lipid kinases, lipid phosphatases, coat proteins)…. Lipids help them to stay soluble Main categories Integral membrane proteins which are permanently bound to the lipid bilayer Peripheral membrane proteins that are temporarily associated with lipid bilayer or with integral membrane proteins Lipid-anchored proteins bound to lipid bilayer bound through lipidated amino acid residues Integral membrane proteins Integral membrane proteins are permanently attached to the membrane. They can be defined as those proteins which require a detergent (such as SDS or Triton X-100) or some other non-polar solvent to be displaced. They can be classified according to their relationship with the bilayer: Integral polytopic proteins, also known as transmembrane proteins, are protein that are permanently attached to the lipid membrane and span across the membrane Integral monotopic proteins are proteins that are permanently attached to the lipid membrane from only one side and do not span across the membrane. Properties of integral proteins Penetrate the lipid bi-layer Transmembrane proteins Amphipathic Domain within the membrane – hydrophobic Domains projecting from the lipid bi-layer – hydrophilic Firmly bind to membrane by hydrophobic interactions Not fixed and may move across the membrane Solubilized with detergents Most have one or more membrane spanning domains (e.g. α-helix) Peripheral membrane proteins Peripheral membrane proteins are temporarily attached either to the lipid bilayer or to integral proteins by a combination of hydrophobic, electrostatic, and other non-covalent interactions. Peripheral proteins dissociate following treatment with a polar reagent, such as a solution with an elevated pH or high salt concentrations. Integral and peripheral proteins may be post-translationally modified, with added fatty acid chains which may be anchored in the lipid bilayer. Lipids – anchored proteins Lipid-anchored proteins (also known as lipid-linked proteins) are proteins located on the surface of the cell membrane that are covalently attached to lipids embedded within the cell membrane. These lipids insert and assume a place in the bilayer structure of the membrane alongside the similar fatty acid tails. The lipid-anchored protein can be located on either side of the cell membrane. Thus, the lipid serves to anchor the protein to the cell membrane Membrane protein function Biological membranes consist of a phospholipid bilayer and a variety of proteins that accomplish vital biological functions. Structural proteins are attached to microfilaments in the cytoskeleton which ensures stability of the cell. Cell adhesion molecules allow cells to identify each other and interact. Membrane enzymes produce a variety of substances essential for cell function. Membrane receptor proteins serve as connection between the cell's internal and external environments. Transport proteins play an important role in the maintenance of concentrations of ions. Various ways proteins associate with lipid bilayer Transmembrane proteins (Integral):cross bilayer in various ways; 1. as a single α helix 2. as multiple α helices, or 3. as a rolled-up β sheet (β-barrel) 4. membrane proteins exposed to cytosolic side but anchored into cytosolic monolayer an amphiphilic α helix Transmembrane Lipid Anchored Proteins: Some “single-pass” and “multipass” proteins covalently attached via fatty acid chain inserted in cytosolic lipid monolayer Lipid Anchored Proteins: attached solely by a covalently bound lipid chain; 5. a fatty acid chain or a prenyl group in cytosolic monolayer or 6. an oligosaccharide linker to phosphatidylinositol in noncytosolic monolayer Membrane-associated proteins (Peripheral): attached on outer surface of membrane by non-covalent interactions with 7. transmembrane membrane proteins on cytosolic side or 8. transmembrane membrane proteins on extra-cytosolic side Structural conformation of transmembrane proteins? Two ways for a protein to cross a biological membrane: 1. α helix – satisfies hydrogen bonding requirements of peptide backbone, a protein can have stable; one transmembrane α helical domain: Single-pass or multiple transmembrane α helical domains: Multiple-pass 2. β-barrel – hydrogen bonding requirements are satisfied … single β-strand/multi-stranded β-sheets are unstable multipass closed β-barrel like structures are stable Categories of peripheral proteins Peripheral proteins contain a wide range of proteins with varied functions; Cytoskeletal Proteins: help in maintaining cell shape and anchoring soluble proteins to membrane e.g. spectrin and actin in erythrocytes Enzymes: An important group of peripheral membrane proteins are water-soluble enzymes that associate with the polar head groups of membrane phospholipids Protein kinases, phosphatases etc. Lipases / phospholipases Palmitoyl protein thioesterases,and Cholinesterases One well-understood phospholipase C from erythrocytes - Hydrolyzes various bonds in the head groups of phospholipids. - Has an important role in degradation of damaged or aged cell membranes. GPI-Anchored Proteins :- While myristoylation, palmitoylation and prenylation all anchor proteins to the cytoplasmic side (inside) of membranes, some extracellular proteins are anchored to the outer surface of cells through the acyl chains of glycosylphosphatidylinositol (GPI) GPI-anchored proteins were discovered at about the same time (1976) by Ikazawa and by Low. GPI-anchored proteins are not trans-membrane but also cannot be removed by alkaline carbonate. Therefore, by definition, they are not peripheral proteins. Their major anchor seems to be through the acyl chains of PI that, without additional electrostatic or hydrophobic interactions, would be very weak. GPI anchored proteins only face outside and are normally clustered. The attached protein is indirectly linked from its C-terminal through a phosphoethanolamine, to a glycan (three mannose sugars), to a glucosamine and finally to the inositol sugar of PI GPI-anchored proteins are found in most eukaryotes, but not bacteria. Although GPI anchors are not considered to be a common motif of membrane protein structure, there are some 45 GPI-anchored proteins in man. In yeast, where all GPI proteins are known, only 15 of the 5,790 proteins (about 1 in 400 proteins) have GPI-anchors. GPI-anchored proteins have a wide variety of essential functions including enzymatic, antigenic, adhesion, membrane organization, and roles in several receptor-mediated signal transduction pathways. Among the better known GPI-anchored proteins are carbonic anhydrase,alkaline phosphatase,Thy- 1 (thymocyte antigen), and BP-3. The GPI-anchored protein can be released from the membrane upon hydrolysis by phospholipase C, and in fact this is how they were discovered. There are several, mostly rare, human genetic disorders that have been linked to GPI proteins. Perhaps the best known is Marfan syndrome Marfan syndrome (MFS) It is a genetic disorder that affects the connective tissue. Those with the condition tend to be tall and thin, with long arms, legs, fingers, and toes. They also typically have overly-flexible joints and scoliosis (sideways curvature of the spine). The most serious complications involve the heart and aorta. The lungs, eyes, bones, and the covering of the spinal cord are also commonly affected Lipid anchored proteins: Lipid-anchored proteins (also known as lipid-linked proteins) are proteins located on the surface of the cell membrane that are covalently attached to lipids embedded within the cell membrane. These proteins insert and assume a place in the bilayer structure of the membrane alongside the similar fatty acid tails. The lipid-anchored protein can be located on either side of the cell membrane. Thus, the lipid serves to anchor the protein to the cell membrane. They are a type of proteolipids (a protein covalently linked to lipid molecules). The lipid serves as a mediator of membrane associations or as a determinant for specific protein- protein interactions. Lipid groups can play an important role in increasing molecular hydrophobicity. This allows for the interaction of proteins with cellular membranes and protein domains. In a dynamic role, lipidation can sequester a protein away from its substrate to inactivate the protein and then activate it by Substrate presentation. Substrate presentation is a biological process that activates a protein. The protein is sequestered away from its substrate and then activated by release and exposure of the protein to its substrate. In the case of an interaction with an enzyme, the protein or organic substrate typically changes chemical form. Substrate presentation differs from allosteric regulation in that the enzyme need not change its conformation to begin catalysis. Overall, there are three main types of lipid-anchored proteins which include: a. prenylated proteins b. fatty acylated proteins and c. glycosylphosphatidylinositol-linked proteins (GPI). A protein can have multiple lipid groups covalently attached to it, but the site where the lipid binds to the protein depends both on the lipid group and protein. Prenylated proteins :- Prenylated proteins are proteins with covalently attached hydrophobic isoprene polymers (i.e. branched five-carbon hydrocarbon) at cysteine residues of the protein. More specifically, these isoprenoid groups, usually farnesyl (15-carbon) and geranylgeranyl (20- carbon) are attached to the protein via thioether linkages at cysteine residues near the C terminal of the protein. This prenylation of lipid chains to proteins facilitate their interaction with the cell membrane. The prenylation motif “CaaX box” is the most common prenylation site in proteins, that is, the site where farnesyl or geranylgeranyl covalently attach. In the CaaX box sequence, the C represents the cysteine that is prenylated, the aa represents any aliphatic amino acid and the X determines the type of prenylation that will occur. Roles and function :- Prenylated proteins are particularly important for eukaryotic cell growth, differentiation and morphology. Protein prenylation is a reversible post-translational modification to the cell membrane. It is important for their signalling functions and is often deregulated in disease processes such as cancer. More specifically, Ras is the protein that undergoes prenylation via farnesyltransferase and when it is switched on it can turn on genes involved in cell growth and differentiation. Thus overactiving Ras signalling can lead to cancer. An understanding of these prenylated proteins and their mechanisms have been important for the drug development efforts in combating cancer. Other prenylated proteins include members of the Rab and Rho families as well as lamins. Some important prenylation chains that are involved in the HMG-CoA reductase metabolic pathway. These isoprene polymers are involved in the condensations via enzymes such as prenyltransferase that eventually cyclizes to form cholesterol. Fatty acylated proteins :- Fatty acylated proteins are proteins that have been post translationally modified to include the covalent attachment of fatty acids at certain amino acid residues. The most common fatty acids that are covalently attached to the protein are the saturated myristic (14-carbon) acid and palmitic acid (16-carbon). Proteins can be modified to contain either one or both of these fatty acids. N-myristoylation (i.e. attachment of myristic acid) is generally an irreversible protein modification that typically occurs during protein synthesis in which the myrisitc acid is attached to the α-amino group of an N-terminal glycine residue through an amide linkage. This reaction is facilitated by N-myristoyltransferase. Proteins that have been myristoylated are involved in signal transduction cascade, protein-protein interactions and in mechanisms that regulate protein targeting and function. An example in which the myristoylation of a protein is important is in apoptosis, programmed cell death. After the protein BH3 interacting-domain death agonist (Bid) has been myristoylated, it targets the protein to move to the mitochondrial membrane to release cytochrome c, which then ultimately leads to cell death. Other proteins that are myristoylated and involved in the regulation of apoptosis are actin and gelsolin. S-palmitoylation is a reversible protein modification in which a palmitic acid is attached to a specific cysteine residue via thioester linkage. Palmitoylated proteins are mainly found on the cytoplasmic side of the plasma membrane where they play a role in transmembrane signaling. The palmitoyl group can be removed by palmitoyl thioesterases. It is believed that this reverse palmitoylation may regulate the interaction of the protein with the membrane and thus have a role in signaling processes. Furthermore, this allows for the regulation of protein subcellular localization, stability and trafficking An example in which palmitoylation of a protein plays a role in cell signaling pathways is in the clustering of proteins in the synapse. Thus, palmitoylation can play a role in the regulation of neurotransmitter release. GPI proteins Glycosylphosphatidylinositols (GPI) proteins are attached to a GPI complex molecular group via an amide linkage to the protein's C terminal carboxyl group. This GPI complex consists of several main components that are all interconnected: a phosphoethanolamine, a linear tetrasaccharide (composed of three mannose and a glucosaminyl) and a phosphatidylinositol. The fatty acid chains of the phosphatidylinositol are inserted into the membrane and thus are what anchor the protein to the membrane. These proteins are only located on the exterior surface of the plasma membrane. Roles and function GPI proteins have a wide range of functions including acting as hydrolytic enzymes, adhesion molecule, receptors, protease inhibitor and complement regulatory proteins. GPI proteins play an important in embryogenesis, development, neurogenesis, the immune system and fertilization. More specifically, the GPI protein IZUMO1R/JUNO (named after the Roman goddess of fertility) on the egg plasma has an essential role in sperm-egg fusion. GPI modification allows for is in the association with membrane microdomains, in apical sorting in polarized cells. Peripheral Proteins – Spectrin :- Spectrin is a cytoskeletal protein that lines the intracellular side of the plasma membrane in eukaryotic cells. Spectrin forms pentagonal or hexagonal arrangements, forming a scaffold and playing an important role in maintenance of plasma membrane integrity and cytoskeletal structure. The hexagonal arrangements are formed by tetramers of spectrin subunits associating with short actin filaments at either end of the tetramer. These short actin filaments act as junctional complexes allowing the formation of the hexagonal mesh. Is named spectrin since it was first isolated as a major protein component of human red blood cells which had been treated with mild detergents; the detergents lysed the cells and the hemoglobin and other cytoplasmic components were washed out. In the light microscope the basic shape of the red blood cell could still be seen as the spectrin- containing submembranous cytoskeleton preserved the shape of the cell in outline. This became known as a red blood cell "ghost" (spectre), and so the major protein of the ghost was named spectrin. In certain types of brain injury such as diffuse axonal injury, spectrin is irreversibly cleaved by the proteolytic enzyme calpain, destroying the cytoskeleton. Spectrin cleavage causes the membrane to form blebs and ultimately to be degraded, usually leading to the death of the cell. Spectrin subunits may also be cleaved by caspase family enzymes, and calpain and caspase produce different spectrin breakdown products which can be detected by Western blotting with appropriate antibodies. Calpain cleavage may indicate activation of necrosis, while caspase cleavage may indicate apoptosis Spectrin on RBC Dimeric spectrin is formed by the lateral association of αI and βI monomers to form a dimer. Dimers then associate in a head-to-head formation to produce the tetramer. End-to-end association of these tetramers with short actin filaments produces the hexagonal complexes observed In humans, association with the intracellular face of the plasma membrane is by indirect interaction, through direct interactions with protein 4.1 and ankyrin, with the transmembrane ion transporter band 3, Protein 4.2 binds the spectrin tail region to the transmembrane protein glycophorin A. In animals, spectrin forms the meshwork that provides red blood cells their shape. The erythrocyte model demonstrates the importance of the spectrin cytoskeleton, in that mutations in spectrin commonly cause hereditary defects of the erythrocyte, including hereditary elliptocytosis and rarely hereditary spherocytosis. Integral Membrane Proteins- Glycophorin A on RBC :- A glycophorin is a sialoglycoprotein (combination of sialic acid and glycoprotein) of the membrane of a red blood cell. It is a membrane-spanning protein and carries sugar molecules. It is heavily glycosylated (60%). Glycophorins are rich in sialic acid, which gives the red blood cells a very hydrophilic-charged coat. This enables them to circulate without adhering to other cells or vessel walls. A particular mutation in Glycophorins is thought to produce a 40% reduction in risk of severe malaria. After separation of red cell membranes by SDS-polyacrylamide gel electrophoresis and staining with periodic acid-Schiff staining (PAS) (used to detect polysaccharides such as glycogen, and mucosubstances such as glycoproteins, glycolipids and mucins in tissues), four glycophorins have been identified. These have been named glycophorin A, B, C, and D in order of the quantity present in the membrane, glycophorin A being the most and glycophorin D the least common. A fifth (glycophorin E) has been identified within the human genome but cannot easily be detected on routine gel staining. In total, the glycophorins constitute ~2% of the total erythrocyte membrane protein mass. These proteins are also known under different nomenclatures but they are probably best known as the glycophorins. Glycophorin D is now known to be a variant of Glycophorin C. Role of GYP: Glycophorin A (MNS blood group), also known as GYPA, is a protein which in humans is encoded by the GYPA gene. GYPA has also recently been designated CD235a (cluster of differentiation 235a). Glycophorins A (GYPA; this protein) and B (GYPB) are major sialoglycoproteins of the human erythrocyte membrane which bear the antigenic determinants for the MN and Ss blood groups. Each glycoprotein crosses the membrane once and has an external N terminal domain (varying in length from 44 amino acids for GPB to 72 amino acids in length for GPA) as well as a C-terminal / cytosolic domain (GPB, 8 amino acids in length; GPA, 36 amino acids in length). There are about 40 known variants in the MNS blood group system. Most of the variants are the result of gene recombinations between GYPA and GYPB. The MNS blood group was the second set of antigens discovered. M and N were identified in 1927 by Landsteiner and Levine. S and s in were described later in 1947. Relevance for infection: The Wright b antigen (Wrb) is located on glycophorin A and acts as a receptor for the malaria parasite Plasmodium falciparum. Cells lacking glycophorins A are resistant to invasion by this parasite. The erythrocyte binding antigen 175 of P. falciparum recognises the terminal Gal-sequences of glycophorin A. Several viruses bind to glycophorin A including hepatitis A virus, bovine parvovirus, influenza A and B, Sendai virus, group C rotavirus, encephalomyocarditis virus and reoviruses Lipid-Anchored proteins – GPI While myristoylation, palmitoylation and prenylation all anchor proteins to the cytoplasmic side (inside) of membranes, some extracellular proteins are anchored to the outer surface of cells through the acyl chains of glycosylphosphatidylinositol (GPI) GPI-anchored proteins were discovered at about the same time (1976) by Ikazawa and by Low. GPI-anchored proteins are not trans-membrane but also cannot be removed by alkaline carbonate. Therefore, by definition, they are not peripheral proteins. Their major anchor seems to be through the acyl chains of PI that, without additional electrostatic or hydrophobic interactions, would be very weak. GPI anchored proteins only face outside and are normally clustered. The attached protein is indirectly linked from its C-terminal through a phosphoethanolamine, to a glycan (three mannose sugars), to a glucosamine and finally to the inositol sugar of PI GPI-anchored proteins are found in most eukaryotes, but not bacteria. Although GPI anchors are not considered to be a common motif of membrane protein structure, there are some 45 GPI-anchored proteins in man. In yeast, where all GPI proteins are known, only 15 of the 5,790 proteins (about 1 in 400 proteins) have GPI-anchors. GPI-anchored proteins have a wide variety of essential functions including enzymatic, antigenic, adhesion, membrane organization, and roles in several receptor-mediated signal transduction pathways. Among the better known GPI-anchored proteins are carbonic anhydrase,alkaline phosphatase,Thy- 1 (thymocyte antigen), and BP-3. The GPI-anchored protein can be released from the membrane upon hydrolysis by phospholipase C, and in fact this is how they were discovered. There are several, mostly rare, human genetic disorders that have been linked to GPI proteins. Perhaps the best known is Marfan syndrome Marfan syndrome (MFS) is a genetic disorder that affects the connective tissue. Those with the condition tend to be tall and thin, with long arms, legs, fingers, and toes. They also typically have overly-flexible joints and scoliosis (sideways curvature of the spine). The most serious complications involve the heart and aorta. The lungs, eyes, bones, and the covering of the spinal cord are also commonly affected.