Biology Final Study Guide PDF
Document Details
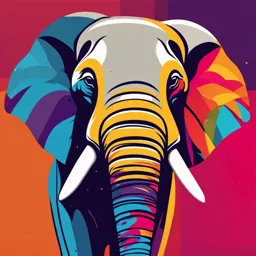
Uploaded by IrreproachableCognition3320
The University of Texas at Austin
Tags
Summary
This document provides a study guide for a biology final exam. It covers topics including ecological organization, biomes, and aquatic systems.
Full Transcript
Define and describe the levels of ecological organization, and summarize the primary areas of investigation within each level The most specific level of ecological organization is the individual level. The individual level studies the physiological, evolutionary, and behavioral ecology of an organis...
Define and describe the levels of ecological organization, and summarize the primary areas of investigation within each level The most specific level of ecological organization is the individual level. The individual level studies the physiological, evolutionary, and behavioral ecology of an organism's structure and the challenges posed by its specific environment. Next is population ecology that studies groups of the same species living together. It analyzes population size and the factors that may affect it. Community ecology studies populations of different species in an area, examining their interactions, community structure, and organization. After community ecology is ecosystem ecology, which is the community of organisms in a specific area PLUS the nonliving physical factors that the organisms may interact with. It focuses on energy flow and chemical cycling between the organisms and their environment. Landscape/Seascape ecology focuses on the factors controlling exchanges of energy across multiple ecosystems, asking questions like how do terrestrial animals affect aquatic systems? Finally, global ecology considers the biosphere, which is the sum of all the planet’s ecosystems and landscapes, examining the regional exchange of energy and materials. Identify terrestrial biomes and how their location and characteristics are influenced by climate and disturbance. The major terrestrial biomes are the tropical forest, savanna, desert, chaparral, temperate grassland, temperate broadleaf forest, northern continuous forest, tundra, high mountains, and polar ice. Biomes are determined by mean temperature and precipitation. Overall, biomes are major life zones that are characterized by vegetation type. However, both natural and human caused disturbances can alter the distribution of biomes. Disturbances such as storm or fire can change a community and remove organisms from it, altering resource availability. Humans have changed many of earth's biomes into urban and agricultural ones. Most terrestrial biomes are named for their major physical or climatic features, including their predominant vegetation. Grasslands are typically found in more moderate climates in the middle latitudes. Biomes also include the animals that are more likely to be adapted to the available vegetation. For example, large grazing mammals are more likely to be found in the grasslands than in the broadleaf forests. Differentiate photic zone and aphotic zone in aquatic systems Aquatic biomes are characterized by their physical and chemical environment. For example, marine environments are characterized by their high salt concentration, whereas freshwater environments typically have an extremely low salt concentration in the water. Aquatic biomes are divided into both vertical and horizontal biomes. Light is absorbed by water and photosynthetic organisms, so the intensity rapidly decreases with the depth of the water. In the upper photic zone, where light can sufficiently penetrate the water, there is enough light for photosynthesis, whereas in the aphotic zone, there is little light penetration. Together, these zones make up the pelagic zone. At the bottom of each zone is the benthic zone, consisting of all sediment and being occupied by benthos organisms. Lakes are divided horizontally into the littoral zones, where waters are shallow enough to support rooted plants, compared to the limnetic zone, where waters cannot support rooted plants Light Penetration: Yes - photic zone, No- aphotic zone Roots: Yes- Littoral zone, No- Limnetic Zone Open Water: Yes- Pelagic Zone, No- Benthic Zone Aquatic biomes have far less latitudinal variation. Evaluate how the distribution of species is limited by biotic and abiotic factors. Biotic factors include other species. The ability of a species to survive and reproduce is limited by these other species that may include predators or herbivores. The presence (or absence) of pollinators, food resources, parasites, pathogens, and competing organisms all act as biotic limitations on species distribution. Abiotic factors, such as temperature, water, oxygen, salinity, sunlight, or soil, also can potentially limit a species’ distribution. If the physical conditions do not allow a species to survive, then the species will NOT be found there. Population ecology studies how both biotic and abiotic factors influence the density, distribution, and size of populations, or a group of single species living in the same area. The density of a population is the number of individuals per unit area. The most common pattern of dispersion is clumped, where individuals are located in patches. Define a population and identify three characteristics of populations: density, dispersion, and demographics A population is a group of individuals of a single species living in the same general area, meaning that they rely on the same resources, are influenced by the same resources, are influenced by similar environmental factors, and are likely to interact and breed with one another. Population boundaries can be either natural or arbitrarily defined. Density is the number of individuals per unit area or volume, while the dispersion is the pattern of spacing among individuals within the population boundaries. The most common dispersion pattern is clumped. Demography is the study of the biotic and abiotic factors that influence population density, including birth, death, and migration. Contrast the logistic and exponential models of population growth using the formulas and graphical representations. The exponential population growth equation represents the populations growth with excess and abundant resources. The equation is dN/dt =rN, where r is the intrinsic rate of increase and N is the number of individuals in the population. However, exponential growth cannot be sustained in any population and the more realistic population model limits growth by incorporating the carrying capacity (K), which is the maximum population size the environment can support. This formula is the logistic population growth population equation, where growth levels off as population size approaches the carrying capacity. dN/dt= rN[(K-N)/K]. The logistic model fits few real populations accurately, but is useful for estimating possible growth. In essence, the exponential model assumes unlimited resources, while the logistic model corrects for carrying capacity and limited resources. Describe the exponential model of population growth and its key assumptions. The exponential model of population growth assumes there are unlimited resources, so the population grows forever, never leveling off. It assumes there is no immigration or emigration, a constant r value, and that there is no variation within individuals. It assumes that all individuals will have the same number of offspring on average ®, regardless of population size. An r value >0 results in a increasing J-shaped curve. What is carrying capacity? Can carrying capacity ever be exceeded? Discuss the consequences of exceeding carrying capacity for populations and ecosystems. Carrying capacity is the maximum population size that a particular environment can sustain. It varies over space and time with the abundance of limiting resources. Energy, shelter, refuge from predators, nutrient availability, water, and nesting sites are all limiting factors. Crowding and resource limitation can have a profound effect on population growth rate. If populations exceed the carrying capacity, the environment may become unsuitable for the population. Resources will be depleted, and the population may die off. A population can only grow until it reaches the carrying capacity. Distinguish how density-dependent and density-independent factors may affect population growth. Give an example of each; and tell how density-dependent factors regulate population growth Density independent factors are any characteristic that is not affected by population density. This occurs with birth or death rates that do not chance with population density. This is things like droughts that kill dune fescue grasses by stressing the roots of the plants, no matter how many plants there are. Density dependent rates are defined by death rates that increase with population or birth rates that fall with rising density. Dune fescue reproduction rates decline as population density increases, due to scarcity of resources. Density dependent factors include disease, competition, and predation. Explain how limited resources and trade-offs may affect life histories. Compare this for r-selected and k-selected species K-selected species are typically larger, with longer lifespans. They produce few young at a time and exhibit logistic growth. R-selected species have short lifespans and are small, usually insects or small animals. They produce many offspring and exhibit exponential growth. A trade off is when an increase in one's life history trait (improving fitness) is coupled to a decrease in another's life history trait (reducing fitness). R selected species prioritize rapid reproduction and high population growth, minimizing parental care. This is allowing them to exploit favorable environments and quickly colonize areas. K selected species prioritize survival and competition for limited resources, leading to traits like longer lifespans, delayed reproduction, and higher parental investment. Describe and analyze the historical and recent growth of the human population The human population grew slowly until the 1700s, when the population finally reached one-billion. Since the 1800’s, the population has grown to eight billion. This is due to industrialization, energy, food resources, water, and medical care becoming more available and reliable. What are the main types of interspecific interactions. give an example of each: predation, parasitism, competition, mutualism, commensalism. Be able to describe how the presence of or increase in one of the interacting species can affect population change in another. Interspecific interactions are the relationships between individuals of two or more species in a community. Competition is (-/-) that occurs when individuals of different species compete for a resource that limits the survival and reproduction of both individuals. Example: weeds growing in a garden compete with garden plants for water and nutrients. Exploitation is the term for any sort of (+/-) interaction where one species benefits by feeding on the other. This can include predation, herbivory, and parasitism. Predation is a (+/-) interaction where one individual kills and eats the other. Herbivory is when (+/-) one organism eats parts of a plant or algae, harming it, but not killing it. Parasitism is a (+/-) interaction where one organism derives its nourishment from another organism, the host, that is harmed in the process. Parasites that live inside the host are considered endoparasites. Mutualism is a (+/+) interaction where both members benefit from the interaction. In mycorrhizae, for example, the plant often transfers carbohydrates to the fungus, while the fungus transfers limiting nutrients, such as phosphorus. Each partner benefits, but each partner also experiences a cost: It transfers materials that it could have used to support its own growth and metabolism. Commensalism is an interaction that benefits one species, but neither harms nor benefits the other species (+/0). Wildflowers that like low light conditions grow underneath trees, but the trees are completely unaffected by these flowers. Use example(s) to explain the principle of competitive exclusion. Competitive exclusion is the concept that when populations of two similar species compete for the same limited resources, one population will use the resources more efficiently and have a reproductive advantage that will eventually lead to the elimination of the other population. Even a slight reproductive advantage will eventually lead to the local elimination of the inferior competitor. Understand and apply the concepts of interspecific competition and ecological niches. In interspecies competition, two species who use the same limited resource will both have a negative effect on them. The species niche is its ecological role, defined as the set of conditions, resources, and interactions it needs or can use. The competitive exclusion principle says that two species cannot coexist if they occupy the same niche, i.e. if they are competing for identical resources. Two species who niches overlap may evolve by natural selection to have more distinct niches, resulting in resource partitioning. What is the difference between the fundamental niche and the realized niche? The fundamental niche is the entire set of environmental conditions they could possibly reproduce in, while the realized niche are the environmental conditions available to them and that are actually used after competition with other animals. How can a species’ ecological niche evolve as a result of natural selection? Two species whose niches overlap may evolve by natural selection to result in more distinct niches as a result of resource partitioning. This is so they do not violate the competitive exclusion principle. How is a food chain different from a food web? Apply the concept of trophic level in the food web. A food web is multiple interconnected food chains, accurately representing all species connections. What is the 10% rule in ecology? When energy is passed between trophic levels, only 10% of the energy will be passed on to the next level. When organisms are consumed, approximately 10% of the energy in the food is fixed into their flesh and is available for next trophic level (carnivores or omnivores). When a carnivore or an omnivore in turn consumes that animal, only about 10% of energy is fixed in its flesh for the higher level. Use examples to explain the differences among foundation species, keystone species and ecosystem engineers Keystone species do not form the ecosystem but help to keep it going. Foundation species modifies the environment and maintains biodiversity. A keystone species is a species that the ecosystem depends on, and so if the species was removed, the ecosystem would suffer and change drastically. Examples are starfish, wolves, and sea otters.. Keystone species are often apex predators. A foundation species physically modifies the physical environment to produce and maintain habitats. Some examples are kelp and hardwood forests. Ecosystem engineers create new environments or modify new ones to suit their needs. An example is a beaver, who creates dams. Explain the basic difference between a bottom-up control versus a top-down control model in the way trophic levels affect one another in an ecosystem. Why isn’t one model the correct one for every ecosystem Bottom up means that organisms are controlled by what they eat, while top down means that organisms are controlled by what eats them. In the bottom up, the abundance of organisms at each trophic level is limited by nutrient supply or the availability of food at lower trophic levels. For example, the supply of nutrients controls plant numbers, which control herbivore numbers, which control predator numbers. In top down control, the abundance of organisms at each trophic level is controlled by the abundance of consumers at higher trophic levels. So, removing top predators (killer whales) increases the abundance of primary carnivores (sea otters), decreasing herbivores (urchins), which in turn increases the abundance of primary producers (kelp). Both of these occur in nature. What is a disturbance, and how does it fit into the nonequilibrium model? A disturbance is an event, such as a storm, fire, flood, drought, or human activity, that changes a community by removing organisms from it or altering resource availability. This emphasis on change in communities formed the nonequilibrium model, which describes most communities as constantly changing after disturbance. Even stable communities can rapidly change. Disturbances help maintain biodiversity by: Removing dominant species and allowing less competitive species to thrive, Creating habitats for pioneer species, Promoting genetic and species diversity through habitat heterogeneity Ecological succession is the change in species that occupy an area after a disturbance. What is the difference between primary succession and secondary succession? Describe an example of each type of succession. Give examples of pioneer species Primary succession occurs in an area that is virtually lifeless. There is no soil and the land is hard rock or lava formations. Soil must be formed before animals or plants can live there. Secondary succession occurs after a disturbance has removed most, but not all, of the organisms. The soil is left intact and plants and animals can move there more quickly. Pioneer species are the first species to return to barren land. In primary succession, these species are moss and lichens. In secondary succession, these species are typically grasses and dandelions. Summarize the overall processes of energy flow and chemical dynamics in ecosystems Chemical cycles include biogeochemical cycles like the nitrogen and carbon cycles. Producers take inert materials from the atmosphere and turn them into organic matter. Consumers obtain these nutrients by eating the producers. Decomposers break down consumers and return these nutrients to the system. Chemicals are recycled while energy leaves the system. Energy flow: Solar energy → Producers → Consumers → Decomposers → Heat (lost). Chemical dynamics: Nutrients cycle among the atmosphere, soil, water, and living organisms in a closed loop. Explain trophic levels in an ecosystem, emphasizing the roles of decomposers and detritivores. Trophic Levels Producers (Autotrophs): Form the base of the trophic pyramid. Use sunlight (photosynthesis) or chemical energy (chemosynthesis) to create organic matter. Examples: Plants, algae, and cyanobacteria. Primary Consumers (Herbivores): Feed directly on producers. Transfer energy from producers to higher trophic levels. Examples: Grasshoppers, deer, and zooplankton. Secondary Consumers (Carnivores/Omnivores): Feed on primary consumers. Examples: Frogs, small fish, and foxes. Tertiary Consumers (Top Predators): Feed on secondary consumers. Examples: Eagles, sharks, and lions. Decomposers and Detritivores: Occupy a crucial role across all levels by breaking down organic matter and recycling nutrients back into the ecosystem. Differentiate between gross primary production (GPP), net primary production (NPP), and net ecosystem production (NEP) GPP represents the total energy input into a system. It is all the energy captured by autotrophs through photosynthesis over a given time. NPP is the energy remaining after the autotrophs use part of the GPP for their own respiration, NPP= GPP -Rautotroph. The Net ecosystem production is the net energy after accounting for respiration for both autotrophs and heterotrophs. NEP = GPP - (Ra-Rh). Describe the effects of human activities on ecosystems, with a focus on eutrophication, "dead zones," and biological magnification Eutrophication occurs when excess nutrients enter water bodies as runoff from fertilizers, sewage, and industrial waste. Excess nutrients stimulate the rapid growth of algae. When this algae dies, bacteria consume oxygen during decomposition, decreasing the oxygen levels in the water and causing hypoxic conditions. This causes fish deaths and reduces biodiversity. Dead zones are areas with low oxygen concentration, where marine life cannot survive. It is mainly caused by eutrophication. It causes the widespread death of aquatic organisms. This disrupts both marine food chains and fisheries, causing economic losses for industries reliant on thriving aquatic ecosystems. Biomagnification is the process by which certain toxic substances increase in concentration as they move up in trophic levels. Pollutants enter the ecosystem through water, soil, or air and are absorbed by primary producers. Consumers then eat these contaminated producers and accumulate high pollutant concentrations. Top predators then accumulate the highest levels of toxins, resulting in toxic effects such as reproductive failure, neurological damage, and death. This reduces biodiversity. They also pose risk to the humans who consume contaminated fish and other organisms. Example: The pesticide DDT causes eggshell thinning in birds, threatening their population. Mercury also accumulates in fish, specifically tuna, threatening humans who eat contaminated organisms. Eutrophication: Excess nutrients → algal blooms → oxygen depletion → biodiversity loss. Dead Zones: Hypoxic areas → widespread death of aquatic life → ecosystem and economic damage. Biological Magnification: Pollutant concentration increases up the food chain → harmful effects on top predators and humans. Efforts to reduce these impacts include sustainable farming practices, reducing industrial emissions, proper waste management, and conservation efforts to restore affected ecosystems. Understand trophic efficiency and the 10% rule in the energy transfer between trophic levels. Trophic efficiency is the percentage of energy that is transferred from one trophic level to the next in an ecosystem. This is very inefficient, where only about 10% of the energy is passed to the next level. Energy enters the ecosystem as sunlight, where producers (autotrophs) convert the solar energy into chemical energy via photosynthesis. Energy loss at each trophic level is due to metabolism, where energy is used during cellular processes and respiration, energy dissipated as heat, and the fact that not all biomass can be consumed or digested. This energy loss restricts the number of trophic levels in a food chain. High productivity ecosystems, like rainforests, can support more trophic levels. Compare and contrast energy pyramids and biomass pyramids in ecosystems Energy pyramids show the flow of energy through different trophic levels in an ecosystem, showing how energy decreases as it moves up the food chain. As energy moves up the trophic levels, 90% of energy is lost as heat, waste, and metabolic processes, passing on only 10%. The pyramid is always broad at the bottom with producers, and narrower at the top with consumers, showing the decreasing amount of available energy. It is typically measured in J or kcal. Biomass pyramids show the total biomass (the total mass of living organisms) at each trophic level in an ecosystem. Biomass is typically measured in grams per square meter. They show the total amount of living material in organisms at each trophic level. Like energy, biomass decreases at higher trophic levels, but not at the same dramatic level. The pyramid can occasionally be inverted. The amount of biomass at each trophic level is directly related to the amount of energy available at the level. Energy pyramids always decrease in size from bottom to top, reflecting the 90% energy loss at each trophic level. Biomass pyramids generally decrease from bottom to top but can sometimes show more biomass at higher trophic levels, especially in aquatic ecosystems where primary producers (e.g., phytoplankton) are small but have high turnover rates, and consumers (e.g., zooplankton) can accumulate more biomass. Explain the biogeochemical cycles, illustrate the water and carbon cycles, and provide a brief overview of the nitrogen cycle. The water cycle is the continuous movement of water within the Earth and atmosphere, involving processes like evaporation, condensation, precipitation, and infiltration. Evaporation is when water from the earth is converted to water vapor by the sun. Transpiration is when plants release water favor through small pores in their leaves. Condensation is when water vapor cools and condenses, forming clouds. Precipitation is water in the form of rain or snow falling back to earths surface. Infiltration is the same as percolation, when water moves through the soil and into the groundwater systems. Run off is water flowing over the ground back to bodies of water. 1. Evaporation 2. Transpiration 3. Condensation 4. Precipitation 5. Infiltration or runoff The carbon cycle is the movement of carbon between the atmosphere, organisms, and the earth's surface. Carbon is a key element in all living organisms and is found in the atmosphere as carbon dioxide. Plants use sunlight to convert atmospheric carbon into organic carbon molecules like glucose. Herbivores and carnivores transfer carbon through the food system, and respiration and decomposition release CO2 back into the atmosphere. Fossil fuel formation stores carbon underground and combustion releases it back to the atmosphere. The nitrogen cycle begins with nitrogen fixation, where atmospheric nitrogen is converted into ammonium or nitrates by nitrogen fixing bacteria in symbiotic relationships with plants. Nitrification is when ammonium is converted into nitrites and then nitrates by nitrifying bacteria. Assimilations is plants absorbing the organic nitrogen. Denitrification is the conversion of nitrates into nitrogen gas, releasing nitrogen back into the atmosphere. 1. Nitrogen fixation 2. Nitrification 3. Assimilation 4. Ammonification 5. Denitrification Water Cycle Water (H₂O) Evaporation, transpiration, condensation, precipitation, infiltration, runoff; Essential for maintaining water availability for ecosystems. Carbon Cycle Carbon (C) Photosynthesis, respiration, decomposition, fossilization, combustion; Regulates climate, supports life through energy storage and release. Nitrogen Cycle Nitrogen (N)Nitrogen fixation, nitrification, assimilation, ammonification, denitrification; Provides essential nutrients for plant growth, supports biodiversity. Define conservation biology and identify its major goals. Conservation biology is the scientific discipline that focuses on the study and preservation of biodiversity, with an emphasis on understanding and mitigating the impacts of human activities on ecosystems and species. Its main goals are preserving biodiversity, restoring degraded ecosystems, and sustaining ecosystem services (air and water purification, soil fertility, pollination, etc), mitigating human impact, managing species and habitats, promoting sustainability, conserving genetic diversity, and educating and engaging the public. In summary, conservation biology seeks to understand the factors leading to biodiversity loss and to apply this knowledge to prevent further damage, restore ecosystems, and promote sustainable coexistence between humans and nature. Explain the concept of biodiversity and distinguish among its three levels Biodiversity is the variety and variability of life on earth, encompassing the diversity of species, ecosystems, and genetic differences within and between organisms. It is a key component of healthy ecosystems and provides numerous benefits to humans, such as food and medicine. The levels of biodiversity are genetic diversity, species diversity, and ecosystem diversity. Genetic diversity is the variation in genetic makeup within and between populations. Examples are different alleles in a gene in a population, providing adaptability, resilience, and evolutionary potential. The second level, species diversity, is the variety and abundance of species in a given area. Examples are different species of plants and animals in a forest, enhancing the ecosystem functions, stability, and human well-being. The ecosystem diversity is the variety of ecosystems both regionally and globally. It includes forests, wetlands, deserts, and coral reefs. This supports a wide range of species, ecosystem services, and resilience. Describe the concept of Minimum Viable Population (MVP) and its importance in conservation The minimum viable population is the smallest population size at which a species is able to maintain its genetic diversity and long term survival, without the risk of extinction due to inbreeding, genetic drift, or environmental changes. It represents the threshold below which a population is expected to face challenges. MVP ensures that a population retains enough genetic variation for evolutionary adaptation. It also ensures demographic stability, accounting for the need for a stable birth-to-death ratio that ensures the population can grow or maintain its size over time. It provides environmental variability, accounting for changes in factors like food availability and climate conditions. It prevents the allele effect, where individual reproductive rates decrease as population size shrinks. In summary, the Minimum Viable Population is a fundamental concept in conservation biology, helping to guide efforts aimed at maintaining healthy, sustainable populations of endangered species. By identifying and maintaining populations above this critical threshold, conservationists can reduce the risk of extinction and preserve biodiversity for future generations. Explain the extinction vortex and its role in species decline The extinction vortex is a concept used to describe the downward spiral that occurs when a species’ population size becomes so small that it becomes increasingly vulnerable to factors. As a species’ population shrinks, the factors contributing to its decline become more pronounced, causing the species to face greater challenges to survival. 1. Population decline- the initial trigger is a drastic reduction in population size 2. Inbreeding depression- individuals in a shrinking population are more likely to mate with relatives, leading to inbreeding. This reduces genetic diversity and increases the frequency of harmful genetic traits. Inbreeding results in reduced fertility, lower survival rates, and weaker offspring. 3. Genetic Drift- in small populations, random changes in gene frequency can have significant effects, reducing the ability of the population to adapt to environmental changes. 4. Demographic Stochasticity- random fluctuations in birth and death rates lead to periods of low reproduction or high mortality 5. Loss of environmental resilience- small populations cannot adapt to changes in the environment, such as shifts in climate, the introductions in new diseases, or changes in food availability. 6. Allele Effect- In some species, individual fitness decreases as the population size shrinks 7. Critical threshold- the factors causing the extinction vortex may become irreversible and the species may face a high risk of extinction, even with conservation efforts. Role in Species Decline: the self perpetuating decline, increased vulnerability, difficulty in recovery A well-known example of the extinction vortex is the cheetah (Acinonyx jubatus), which has experienced significant population declines. The cheetah's small population size has resulted in low genetic diversity, leading to inbreeding and genetic issues, such as low sperm quality and weak immune responses. These factors, combined with habitat loss and human-wildlife conflict, create a feedback loop that hinders the cheetah's recovery Identify and provide examples of human activities that threaten biodiversity, such as habitat fragmentation, and explain how these contribute to biodiversity loss. Habitat destruction refers to the direct alteration or elimination of natural habitats due to human development and land use changes. Examples include deforestation and wetland draining. These destroy the home environments for species, forcing them to either migrate to new areas or face extinction if no suitable habitats remain. It affects food chains and ecosystem services, like pollination and water filtration. Habitat fragmentation occurs when large continuous habitats are broken into smaller, isolated patches due to human activities, making it difficult for species to survive or reproduce. Roads and highways often divide habitats, disrupting breeding grounds and migration routes. Agricultural expansion is when large tracts of land are converted into small, isolated patches of forest, reducing connectivity between ecosystems. Fragmentation decreases genetic diversity by isolating populations, increasing the risk of inbreeding. Other examples include pollution, overexploitation, climate change, and invasive species, Use an example to illustrate the concept of biological magnification One well-known example of biological magnification is the case of DDT (dichlorodiphenyltrichloroethane), a pesticide widely used in the mid-20th century. Although DDT is effective at controlling pests, it is also a persistent chemical that does not break down easily in the environment. Over time, it accumulated in ecosystems and caused significant harm to wildlife, especially birds of prey, such as bald eagles. Describe the advantages and disadvantages of movement corridors and biodiversity hotspots in conservation Movement corridors are areas of habitat that connect isolated patches of natural habitat, allowing wildlife to move between them. These corridors help maintain gene flow, reducing the risks of inbreeding and providing access to food. They increase genetic diversity, enhance species survival, provide resilience to climate change, and promote ecosystem connectivity. However, they are costly and facilitate the spread of invasive species. They increase the likelihood of conflicts between wildlife and humans, such as vehicle collisions, poaching, and crop damage. Both movement corridors and biodiversity hotspots play crucial roles in conservation, but they each come with trade-offs. Movement corridors are valuable for ensuring species mobility and genetic diversity, but they require careful planning and management to avoid conflicts with human land use and invasive species. Biodiversity hotspots are key to conserving high levels of species diversity, but they may risk neglecting other important ecosystems and create challenges related to human pressure and inequitable conservation. Effective conservation strategies often incorporate both concepts, using movement corridors to maintain connectivity between habitats within hotspots, while also expanding efforts to protect other important ecosystems beyond traditional hotspots.