Biology Chapter 2024 PDF
Document Details
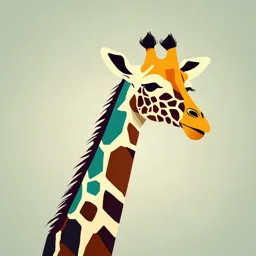
Uploaded by ManageableMeerkat
2024
Tags
Summary
This document is a chapter on introductory biology focusing on bacteria, viruses, and their characteristics, structures, and functions. It could be part of a larger textbook.
Full Transcript
Biology 2024 Thank you to our chapter sponsor: 1 Introduction to Bacteria Bacteria have been around for a very long time. In fact, they are the oldest known forms of life on Earth! The earliest fossils we know are of prokaryotes. That’s a group of organisms that includes bacteria. These fossils are...
Biology 2024 Thank you to our chapter sponsor: 1 Introduction to Bacteria Bacteria have been around for a very long time. In fact, they are the oldest known forms of life on Earth! The earliest fossils we know are of prokaryotes. That’s a group of organisms that includes bacteria. These fossils are about 3.5 billion years old! Since then, bacteria have evolved into a wide variety of types. They have also adapted to many different environments. They can live inside the human body, at the North Pole, and even at the bottom of the ocean! Did you know? There are more bacteria living in your mouth than there are people who have ever lived on Earth! Size of Bacteria Bacteria are single-celled organisms. This means that each bacterium is made up of only one cell. This is very different from humans. Our bodies are made up of trillions of cells. Bacteria are much smaller than human cells. Bacterial cells are between about 1 and 10 μm long. And most of them are only 1 to 2 μm in diameter. 1 μm, or micrometre, is 1 000 times smaller than a millimetre. That is much smaller than the human red blood cell, which is about 7 μm in diameter. Parts of a bacteria (©2023 Let's Talk Science). Even though they are small, bacterial cells have many different parts. 1. 2. 3. 4. 5. 6. Diagram showing the relative sizes of some very small things including bacteria, which are typically around 1 to 2 μm in diameter (Source: Michigan Nanotechnology Institute for Medicine and Biological Sciences). 2 7. Pili are hair-like structures that help bacteria attach to surfaces and to other bacteria. A plasmid is a small, circular, doublestranded molecule of DNA. Plasmids contain genes that help when the bacteria is in danger, such as when it is exposed to an antibiotic. Ribosomes are little round structures that produce proteins. They are found floating in the cytoplasm. The cytoplasm is a thick, water-based (aqueous) solution. Ribosomes and nuclear materials are found in the cytoplasm. Salts, nutrients, minerals and enzymes are also found in the cytoplasm. The cytoplasmic membrane is a thin layer of phospholipids and proteins. It controls the movement of nutrients in and out of the cell. The cell wall is a rigid layer that gives the bacteria its structure. It also protects the cytoplasmic membrane. The capsule is a third layer that helps keep the bacteria from drying out. It can also help protect the bacteria. It is only present in some types of bacteria. 8. 9. The nucleoid is made of DNA, RNA and proteins. The nucleoid controls the activity of the cell. The nucleoid is found within the cytoplasm. The flagellum is a structure that helps the bacteria move around and sense their environment. Classification of Bacteria There are millions of different types of bacteria in the world. That’s why it’s important to have a way to classify them. Scientists usually classify bacteria using two characteristics: The thickness of its cell wall Its shape Scientists use Gram staining to measure the thickness of cell walls. To do this, they stain bacteria with a dye called crystal violet. Thick cell walls keep the violet colour of the dye. Thin cell walls do not. Gram Positive bacteria have thick cell walls. They appear blue or purple when dyed. Gram Negative bacteria have thin cell walls. They appear pink or red when dyed. Bacteria Shapes Bacteria are classified into three major groups based on their shape. The first group is spherical (cocci), the second is rod-shaped (bacilli), the third is spiralshaped and others. Bacteria can be grouped according to their shape. Bacteria are typically either spherical, rod-shaped or have a spiral shape (© 2019 Let’s Talk Science based on an image by ttsz via iStockphoto). 3 Are Bacteria Bad? We often think of bacteria as being ‘bad’. That’s because some bacteria are pathogenic. This means they can make us sick. Lots of diseases and conditions are caused by bacteria. These include: tetanus typhoid fever tuberculosis strep throat anthrax food poisoning Introduction to Viruses Viruses have a big impact on humans. Coronavirus 2019 (COVID-19), the common cold, the flu, Severe Acute Respiratory Syndrome (SARS) and diseases like Acquired Immunodeficiency Syndrome (AIDS) are all caused by viral infections. Scientists have worked for hundreds of years to understand viruses and find better ways of fighting them. Virus Structure Electron microscope images of: Streptococcus pneumoniae which causes pneumonia (left), Salmonella typhimurium which causes food poisoning (centre) and Treponema pallidum, which causes syphilis (right). (Sources: CDC/Dr. Richard Facklam [Public domain] via Wikimedia Commons, Photo: Volker Brinkmann, Max Planck Institute for Infection Biology, Berlin, Germany [CC BY 2.5] via Wikimedia Commons, and CDC/Dr. David Cox [Public domain] via Wikimedia Commons). Viruses are very small. They are usually 17 to 400 nanometres in diameter. A human hair is much larger - about 80 000 nanometres wide. Even bacteria are about 100 times larger than viruses. Because viruses are so small, they have simple structures. They are much simpler than animal or bacterial cells. Viruses have three basic parts: Did you know? Researchers are starting to use bacteria to dye clothing. This could be a lot safer and more sustainable than traditional dyes. But only a small fraction of the bacteria in the world cause us harm. In fact, many bacteria are helpful! For example, probiotic bacteria in our digestive system helps protect us from other bacteria. Bacteria are all around you - even in your kitchen! Lots of food and beverages are made from bacteria. These include: vinegar kombucha yogurt chocolate kimchi cheese sourdough bread Bacteria are diverse and interesting living things. People may think of them as things that make us sick, but they do a lot of good for us as well! 4 Parts of a virus (©2023 Let's Talk Science). 1. 2. 3. Nucleic acid is a set of genetic material. It is either Deoxyribonucleic acid (DNA) or Ribonucleic acid (RNA). It is packaged in a protein shell. A capsid is a protein coat that surrounds and protects the DNA or RNA. The capsid is made of proteins called capsomeres. The envelope is a covering for the capsid. It is made up of phospholipids and glycoproteins. Phospholipids form a fatty layer. Glycoproteins are a mix of proteins and carbohydrates.Not all viruses have envelopes. The ones that do not are called naked viruses. Even though they only have a few parts, viruses come in many different shapes. Pictures of viruses can look like creatures out of science fiction. Some have heads that are polyhedrons, or many-sided three-dimensional shapes. These are connected to little jointed shapes that look like legs. Other viruses look like round popcorn. Like bacteria, they can be classified based on their shape. Viruses are classified into four main categories: Helical Viruses These look like long rods. They can be rigid or flexible. The helical virus below is a tomato mosaic virus. It causes the leaves and fruit of tomato plants to appear blotchy. Polyhedral Viruses These have complicated structures. Their capsids can be attached to structures that look like legs. These are called tail fibres. The virus below is a bacteriophage. It infects bacteria. Bacteriophage (Let’s Talk Science using an image by VectorMine via iStockphoto). Scientists are not sure if viruses are alive or not. They do not share many of the characteristics of living things. They do not have cells. They have DNA or RNA, but they do not have a cell membrane or organelles. They cannot make their own energy. In other words, they don’t have a metabolism. A metabolism is all the chemical reactions an organism needs to live. They cannot reproduce on their own. They must live inside a host cell to make more viruses. Hosts can be bacterial, plant or animal cells. Polyhedral virus (Let’s Talk Science using an image by VectorMine via iStockphoto). Enveloped Viruses These are shaped like spheres and have a protein, fat or carbohydrate coat over their capsid. Coronaviruses are a type of enveloped virus. The COVID-19 pandemic is caused by a virus like this. So is influenza. Complex Viruses Are Viruses Living Things? Helical virus (Let’s Talk Science using an image by VectorMine via iStockphoto). These are many-sided viruses. Their capsids can have different numbers of sides. Most have 20 triangular sides and 12 vertices, or corners. The polyhedral virus below is the adenovirus. It causes respiratory illnesses. SARS and Middle East Respiratory Syndrome (MERS) are also caused by enveloped viruses. The image at the bottom of the previous column is an influenza virus. It causes respiratory illnesses like coughs and sore throats. They do not use energy on their own. Viruses only use the energy of their host cells. They are inactive before they come into contact with them. Some bacteria also work this way. They don’t really respond to their environments. Viruses interact with their host cells to infect them, but they don’t do too much more. How do Viruses Reproduce? Enveloped virus (Let’s Talk Science using an image by VectorMine via iStockphoto). Whether they are living or not, viruses can reproduce. This is what happens in your body when you have a viral infection. The virus uses your cells to replicate itself. This process is often called the virus life cycle. The steps in this cycle are illustrated on the next page. 5 Marine Microbiology: Meet the Microbes of the Sea! "Microbes" is another name for microorganisms. They are tiny living things. They’re too small for people to see with just their eyes. But they’re there! They live in every type of environment you can imagine. In rainforests, in the Arctic, in deserts and at the bottom of the ocean. The ocean is a marine environment. And a single drop of seawater can contain millions of marine microbes. These tiny living things help supply oxygen and food to other organisms that live in the ocean. They even help keep the ocean clean. What are the different types of marine microbes? There are many types of marine microbes. These include bacteria, which you can also find on land. Most bacteria are single-celled organisms. That means their bodies are made up of only one cell. In the ocean, bacteria support many chemical processes, including photosynthesis. Phytoplankton are another group of marine microbes. These microscopic creatures can create their own food. Most of the time, they do this through photosynthesis too. This means they convert sunlight and carbon dioxide from the ocean into oxygen and sugars. Have you heard of marine plants called algae? They are a common type of phytoplankton. Did you know? Only some of the oxygen you breathe comes from plants and trees on land. Every second breath you take comes from the billions of phytoplankton living in the ocean! Other types of microbes also live in the ocean. Fungi and viruses for example. There are also archaea and protists - single-celled organisms that look like bacteria. 6 Did you know? Microscopic animals called rotifers live in brackish environments. That’s where freshwater and saltwater mix. Unlike other microbes, rotifers have more than one cell! On the next level of the food chain, bigger animals, like fish and whales, eat zooplankton. So without phytoplankton, there would be no life in the oceans! What do marine bacteria do? Microscopic rotifer (Bob Blaylock at English Wikipedia [CC BY-SA 3.0] via Wikimedia Commons). How do ocean food chains work? Cyanobacteria are a common type of marine bacteria. They are also a type of phytoplankton. That means they produce their own food through photosynthesis. Many cyanobacteria are also an important part of the nitrogen cycle. They convert nitrogen to a form that other marine organisms can use. Marine bacteria also help clean up ocean pollution. Some break down and consume dead marine animals. Others can even help break down oil after an oil spill! A food chain describes the relationship between organisms that eat other organisms. Plants are the base of land food chains. For example, cows eat grass. Then some people eat cows. Some marine bacteria are bioluminescent. And some of them live in symbiotic relationships with other marine organisms. Often, the relationship is mutualistic. That means both organisms benefit. Phytoplankton are the base of ocean food chains. They get their energy from the Sun. Then, on the next level of the food chain, zooplankton eat phytoplankton. Zooplankton include krill and the larvae of crabs and fish. Most of these animals are tiny, but some are larger, like jellyfish. They drift along with the ocean currents because they can’t swim very well and they can’t create their own food using photosynthesis. You may have seen pictures of scary looking fish called anglerfish that hunt their prey using lights. Those “lights” are actually bioluminescent bacteria! They help the fish catch food. In return, the fish give the bacteria a safe place to live and nutrients to survive. Artist’s illustration of an anglerfish (Source: spyder24 via iStockphoto). Comparison of terrestrial and marine food chains (Let’s Talk Science using images by lukaves via iStockphoto). Unfortunately, not all marine bacteria are helpful. Some of them can hurt other marine organisms or even cause disease in humans. For example, Vibrio cholerae are the bacteria responsible for the disease cholera. And as ocean temperatures rise, these types of harmful bacteria are spreading. 7 Colouring Clothing with Bacteria How do scientists study marine microbes? It’s not easy for scientists to study marine microbes. Only 1% of marine bacteria can be grown in a laboratory. So scientists have to study them in the ocean. The tiny size of bacteria makes this very hard. Do you love to wear clothing that is bright and colourful? Or are dark or muted colours more your style? People can make clothing in just about any colour they can imagine. Have you ever wondered where those colours come from? Scientists sometimes collect microbes using plankton nets. These cone-shaped nets have a very fine mesh. A container at one end of the net collects the samples. Colourful scarfs (Source: Alfred Derks via Pixabay). What gives clothing its colour? Most clothing today is coloured using synthetic dyes. A dye is something that colours materials like paper, plastic, leather and fabric. Dyes react chemically with the materials they are applied to. This keeps the colour from being washed away. US Environmental Protection Agency staff preparing a plankton net (Source: USEPA Environmental Protection Agency via Wikimedia Commons). Remote sensing is another way to study marine microbes. For example, scientists use satellites to study algal blooms from space. Scientists also now use DNA samples from ocean bacteria to study new types of marine microbes. In the past, people dyed yarn, fabric and clothing using what they had on hand. This included plants, animals and minerals. People used many parts of plants, including roots, berries, leaves, bark and wood to make dyes. Dyes made from natural materials are often called natural dyes. Going to the beach this summer? Remember that the water is full of creatures too tiny to see. Those critters help support all forms of life beneath the waves. And by producing the oxygen you breathe, they help you survive, too! 8 Examples of plants used to make dye (Source: mofles via Getty Images). Making natural dyes is very time consuming. Sometimes, it can also be expensive. It can also require a lot of water and land. Synthetic Dyes This damage can lead to problems with people’s immune systems. It can even lead to cancer. Some dyes also contain heavy metals. These can lead to learning disorders and kidney disease. In the mid-1800s, an 18-year old English chemist named William Henry Perkin was experimenting with coal tar in hopes of finding a cure for malaria. Coal tar is a thick, sticky liquid that is left over when coal is heated. He did not find a cure for malaria, but he did create an oily liquid that stained cloth purple. Scientists think this was the world’s first synthetic dye. A synthetic dye is a dye that is produced chemically. Did you know? The colour of Perkin’s dye was known as mauve in England, after the French name for the mallow flower. Chemists later called it mauveine. Mauveine (Hex #8D029B) Perkin’s dye proved to be very popular. Soon chemists began experimenting to find new colours. By the 1860s, synthetic dyes were big business and a whole rainbow of colours were available. Today, almost all dyes used to colour clothing are synthetic. Synthetic dyes are used because: They can produce bright colours They do not fade easily in light They are inexpensive to make They will not wash out They can colour synthetic fabrics like polyester and nylon, unlike natural dyes Did you know? It is estimated that up to 200 000 tons of synthetic dyes are produced for the textile industry each year. Problems with Synthetic Dyes As popular as they are, synthetic dyes pose some serious health risks for people and the environment. Runoff from textile dyeing factories can pollute water systems and harm ecosystems. Synthetic dyes can also impact human health. Dyes, such as azo dyes, are very toxic and can damage cells. Two women working in a pool of dye (Source: jun xu via Getty Images). Because of these concerns, natural dyes are making a comeback. But they haven’t just been using the old tried and true plants and animals. A new and unusual source of dyes is bacteria. Why Bacteria? Most living things have cells that can produce pigments. For example, humans produce melanin. This is what gives our skin, eyes and hair their colours. Pigments don’t just give us colour, they can also give us protection. The melanin in our skin and eyes helps protect us from damaging UV radiation. Did you know? Eumelanin is the pigment associated with darker colours, like brown and black, in our skin and hair. Pheomelanin is associated with other colours, like red, pink and yellow. Like people, bacteria produce pigments to help them survive. The pigments bacteria produce help them live in environments with extreme temperatures, pH and radiation levels. Pigments also help some bacteria undergo photosynthesis. Did you know? Cyanobacteria and purple and green sulphur bacteria are two types of bacteria that undergo photosynthesis. Their pigments absorb energy from sunlight, which they convert into a form of energy they can use. 9 Left to right: Cyanobacteria in water and on a shoreline (Source: Rosser1954 [CC BY-SA 4.0] via Wikimedia Commons); Purple sulphur bacteria (Source: Public domain image by kOchstudiO via Wikimedia Commons); Green sulphur bacteria in a hot spring (Source: IngerEriksen via Getty Images). Here are some examples of bacteria and the pigments they produce. The columns on the right describe their habitats and the roles the pigments play. Pigment Bacteria Habitat Role in bacteria Violacein (purple) Chromobacterium violaceum Freshwater and marine Defending against habitats other microorganisms Carotenoids (yellow and orange) Serratia marcescens Soil and water Surviving in areas with high levels of radiation Prodigiosin (red) Serratia marcescens Soil, human digestive system Defending against other bacteria Prodigiosin (red) Streptomyces coelicolor Soil Defending against other bacteria, helping cells use iron Pyocyanin (greenishblue) Pseudomonas aeruginosa Inside plants and animals Helping cells use iron, changing to reddishpink at lower pH levels Did you know? Scientists have even used some bacteria to break down synthetic dyes in the environment. How can bacteria be used to dye clothes? A number of pigments produced by bacteria can be used as dyes. When scientists first began using bacterial dyes, they grew bacteria right on the fabric. With this “live dyeing” method, each batch of fabric is unique. Their patterns reflect how the bacterial colonies grow. 10 Samples of fabric after bacterial dyeing (Source: Laura Luchtman & Ilfa Siebenhaar, Living Colour. Used with permission). The advantage of live dyeing is that the fabric does not need any harmful chemicals or pre-treating. It also uses very little water and energy. But so far, this method has only been used in laboratories. This is because some of the bacteria can be harmful to people. Live dyeing also needs to be done in a way that does not accidentally introduce other types of microorganisms onto the fabric. It would be challenging to use this method on a large scale. The Future of Bacterial Dyeing A number of companies are getting involved in genetically engineering bacteria to produce pigments. For example, Ginkgo Bioworks is engineering strains of bacteria to produce the pigment violacein. Colorifix is now growing and using bacteria on a larger scale. Here is how they do it. 1. Find a colour in nature that they want to create as a pigment. 2. Identify the DNA code producing that specific colour. 3. Engineer the microbes to produce the pigment they would in nature. 4. Ship about 5 ml of the bacteria to a dyehouse or mill. 5. Grow the bacteria at the dyehouse or mill using a fermentor. A fermentor is a big tank where the bacteria can grow and divide very quickly. The bacterial cells produce the pigment. It only takes 18 to 24 hours to make a large quantity of the pigment mixture. 6. Dye the fabric using the pigment mixture in a standard dyeing machine. DNA sequences for pigments found in nature are inserted into bacteria (Source: Colorifix Ltd. Used with permission). 11 What are the benefits of using bacteria? Unlike synthetic dyes, the pigments produced by bacteria are nontoxic and biodegradable. Because of the way they’re grown, pigments from bacteria attach to fabric without the use of harsh fixatives. Fixatives are chemicals that help dyes and pigments attach to fabric, so they don’t wash out. Unlike other natural pigments, bacteria are cheap to produce, grow quickly and do not require any land to produce. As an added bonus, many bacteria pigments can kill microorganisms and even help to treat cancer! All this plastic is a serious problem for marine life. Plastic waste is 80% of all marine debris. It gets eaten by animals like whales, fish, and seabirds. These animals then develop major health issues. Other kinds of plastic debris can entangle or strangle sea life. As the world becomes more concerned about sustainability, the future of dyes and pigments may come from the tiny but mighty bacteria. Plastic-Eating Bacteria: Nature's Recyclers The Plastic Problem Think about all the plastic you see everyday. You drink water out of plastic bottles and you eat food that's wrapped in plastic. The pen in your hand is probably plastic, and the device you’re reading this on is partly made of plastic. Where does all this plastic end up? We hope that it gets recycled into new plastic. But most of it does not get recycled. Instead, it ends up in landfills and in our waterways. Did you know? Only 9% of the plastic ever made has been recycled. The amount of plastic in the environment has become a big problem. And it gets worse every year. Over 12 million metric tonnes (Mt) of plastic enter the ocean every year. This is on top of the 362 million Mt that are already there. 4.1 million Mt of that comes from Canada. 12 Sea turtle with plastic grocery bag handle caught on its neck (Source: Jag_cz via Getty Images). Microplastics are another problem. Microplastics break off of larger plastic items and enter the food chain. There are many advantages to using plastic. The big disadvantage, though, is that most of it doesn’t biodegrade. An empty jug of laundry detergent that enters the ocean will still be in the ocean hundreds of years from now. Even the plastic engineered to be biodegradable takes a very long time to break down. Dealing with this needs some innovative thinking. What if something ate it? In March 2016, scientists in Japan made a startling discovery. They found that some of the bottles at a recycling plant were being broken down by bacteria. The newly discovered bacteria was named Ideonella sakaiensis. Did you know? This bacteria was named after the recycling plant in Sakai, Japan. Ideonella sakaiensis is a gram-negative, rod-shaped bacteria. Scientists believe that it has naturally evolved to use a certain type of plastic for food. For some time, scientists have thought about using microorganisms, like bacteria, to break down plastic. They have mostly tried using Escherichia coli (E. coli) bacteria. But E. coli doesn’t break down plastic very well. It prefers sugars over everything else. Ideonella sakaiensis, on the other hand, prefers a type of plastic called polyethylene terephthalate, or PET. PET is a type of plastic commonly used to make water bottles and food packages. One of the common roles of enzymes is in digestion. Digestive enzymes in bacteria work to break down larger molecules into smaller ones. These smaller molecules can then be absorbed by the bacteria. The bacteria takes what it needs and gets rid of (excretes) the rest. Ideonella sakaiensis produces an enzyme called hydrolyzing PET (or PETase). This breaks down the bonds in the polymer to form monomers. Then it absorbs the monomers to use for energy. This is similar to the way humans break down food. How to Eat a Plastic Bottle What do we mean when we say that bacteria can “break down” plastic? First, it’s important to know that PET plastic is made up of repeated units of C10H8O4. This type of molecule is called a monomer. Monomers can chemically react with other monomers to form long chains called polymers. Different types of plastic use different monomers to make polymers. Bacteria digesting a plastic water bottle ©2023 Let’s Talk Science). Hurry Up! Monomer of polyethylene terephthalate (PET) (Source: Schippmeister [CC BY-SA 4.0] via Wikimedia Commons). The bonds between the monomers are very strong. This makes plastic tough and durable. You can experience this yourself. Try to rip apart a plastic water bottle with your bare hands. It’s impossible! The strength of polymers is what allows plastics to remain in the environment for such a long time. Natural processes can normally only break plastic into smaller pieces. They cannot break polymer chains apart. Ideonella sakaiensis is different because it can break down the bonds between monomers. It does this using enzymes. Living creatures use enzymes for many different life processes. They speed up the chemical reactions that take place in cells. On the surface, using bacteria seems like a great way to get rid of all that plastic waste clogging up the environment. We could grow a bunch of Ideonella sakaiensis, add it to the plastic waste, and let the bacteria feast! Unfortunately, there are a few drawbacks to this idea. The first problem is that there is an awful lot of plastic waste. In the history of plastic, we have produced 8.3 billion Mt of the stuff. 79% of that has gone into landfills or into the environment. It will remain there until something finally comes along to break it down. It would be hard to make enough bacteria to eat the plastic we send into the environment every year, let alone the plastic that is already there. The second problem is that bacteria digest the plastic very slowly. 13 Other common items are made from a different type of plastic called polyethylene (PE). PE is the plastic that is used in shampoo and laundry detergent bottles. It is also used in things like plastic buckets and children’s toys. It is not biodegradable, and so far none of the bacteria can digest it. Large pile of plastic bottles (Source: STORYPLUS via Getty Images). So, scientists wondered if there was a way to speed up the process. In 2018, researchers at the University of Portsmouth figured out the 3D structure of PETase. This allowed them to engineer PETase to break down bottles in days, rather than months. This is still too slow to overcome all the waste we’ve created, but it’s a good start. In 2020, researchers at the same university combined PETase with another, similar enzyme. This produced a ‘super-enzyme’ that does the job six times faster. But there is still hope. In February 2023, scientists suggested the answer might be in waxworms. Waxworms are the larvae of wax moths. The scientists found that bacteria in the gut of the Lesser Waxworm (Achroia grisella) were able to digest low-density PE. This kind of plastic is used in things like shopping bags and bubble wrap. While there is still a lot of research to do, it is a promising start. Is it hot in here? A second problem is that the enzymes need to be in an environment where the temperature is above 30°C. These environments are only found in certain parts of the world. The rest of the world is too cold. This means that the cost of heating up the bacteria to get them to digest plastic, both in terms of money and environmental impact, is too expensive. Luckily, Swiss scientists found bacteria that can digest some types of plastic in colder temperatures. They saw that these bacteria made enzymes that broke down plastic at 15°C. They believe the enzymes could work in temperatures as low as 4°C. Everything Must Go! The downside of the cold-temperature bacteria is that they can only break down a few specific types of plastic. This is a problem with plastic-eating bacteria in general. For example, only bacteria that can make PETase can break down PET plastic. And the cold-temperature bacteria can only break down biodegradable plastics like polyester-polyurethane (PUR). 14 Larvae of the Lesser Waxworm (Achroia grisella) (Source: Rasbak [CC BY-SA 3.0] via Wikimedia Commons). What Goes In Must Come Out The last thing to note about plastic-eating bacteria is that, much like when you eat food, they produce waste. This waste is in the form of the small monomers that the plastic was made from in the first place. It looks like goopy liquid. Unlike some other waste, this has some potential uses. It can be recycled into other plastic objects. For example, a recycling plant in France is using bacteria to break down PET plastic. And the waste is being used to make new PET objects that researchers say are good as regular PET plastic. The future of using bacteria to break down plastic looks bright, and maybe a little slimy. Discovering Drugs with the Help of Machine Learning Every cell in the human body contains proteins. These molecules are some of the building blocks of life. The human body is made up of billions of them and they are essential for helping us digest food, move and even think. Proteins help build and repair the body's tissues. They also keep the body working as it should. There are thousands of different proteins in the human body. Each one has one or more specific functions. ways to form proteins with a variety of shapes and sizes. The order of the amino acids determines the 3-dimensional structure of a protein. The structure determines the protein's function in the body. Determining the order of amino acids coded by human genes used to be a lot of work. But thanks Proteins as Drugs More and more proteins are now used in the development of drugs that treat illness and disease. Finding a new protein for a drug is often like finding a needle in a haystack. Researchers look at thousands of proteins found in nature until they find one that comes close to doing what they want it to do. Then comes the long and difficult process of getting the protein to do what they want it to in the body without any negative effects. All this is hard and takes lots of time and resources. More than half the time the process fails to deliver a protein that works. The Protein Folding Problem Proteins are made from long chains of molecular building blocks called amino acids. There are 20 different types of amino acids that make up most proteins. Amino acids can link up in many different 3D images of a variety of naturally occurring proteins both within and outside of a cell (Source: Screen grab from the Protein Data Bank https:// cdn.rcsb.org/pdb101/molecular-machinery/). to the Human Genome Project, researchers are now able to do it very quickly. What they cannot do quickly is figure out how the chains of amino acids fold up and carry out a protein's function. This is because there are so many ways a protein can fold. To predict the ways a protein could fold takes a huge amount of computing power. This is where Artificial Intelligence (AI) and Machine Learning (ML) can help. Steps in protein folding (Source: Adapted from an image by AMGEN. Used with permission). 15 Machine Learning and Protein Folding On July 22, 2021, DeepMind, a part of Google, published research on proteins and ML. ML was used to predict the structures of about 100 000 proteins. The researchers used a system called AlphaFold. AlphaFold uses protein data to learn how to predict protein structures. Even though the model's predictions aren't perfect, they are getting better every day. RoseTTAFold is a similar tool. It was developed by the Institute for Protein Design (IPD) at the University of Washington. It is important to note that it takes a very large amount of data about proteins to help design new protein drugs. This data is mostly collected from lab tests and clinical studies on patients. Small proteins binding with a large receptor protein in the membrane of a nerve cell (Source: JUAN GAERTNER/SCIENCE PHOTO LIBRARY via Getty Images). Did you know? The term generative biology comes from the models they use. We call these generative computer models. Proteins and Generative Biology Making Connections Finding and understanding natural proteins takes a very long time. But what if scientists could figure out a way to design protein drugs faster and with greater success? Or better still, what if they could skip the process of finding a protein in nature and just design one from scratch? This is where generative biology comes in. Predicting protein structure is not all that RoseTTAFold and AlphaFold can do. They can now also model how proteins connect (bind) to each other. Being able to see how proteins bind to each other is a key aspect of drug development. Generative biology is about using computers to learn from data to generate new data. For example, researchers can use data about proteins to train computer models. The more data that is put into these models, the better, faster and more successful these models will be. In the future, the computer models could learn how to make any protein people might want. ML could be used to create specific proteins that would bind to a specific target. This would be much faster than making them from scratch in the lab. The hope is that ML can help figure out what proteins are useful in fewer steps and with fewer surprises. By decreasing research time drug companies can get treatments to people even faster than before. Let’s Talk Science appreciates the contributions of Natasha Bond from Amgen in the development of this section of the handbook. Traditional protein drug discovery versus generative biology (Source: Adapted from an image by AMGEN. Used with permission). 16 Design your own bacteria! Consider using the Design & Build Process with this challenge. This activity will help build skills related to the Identify the Problem, Research, Generate Ideas, Plan, and Reflect & Share phases of this process. Materials: Paper and writing/drawing tools (e.g., pencils, coloured pencils, markers) or A device with a digital drawing program What to do! Imagine you are a research scientist working with bacteria. Your task is to design a new bacterium that can help humans in some way. 1. 2. 3. 4. 5. Identify the Problem - Think about what you want your bacteria to do. How will it help people? Research - You might want to review the sections in the handbook about bacteria, including Introduction to Bacteria, Colouring Clothing with Bacteria, and Plastic-Eating Bacteria: Nature's Recyclers to get an idea of some different aspects and functions of bacteria. Generate Ideas - What kind of parts might it need? What might it need for protection? What might it need to survive in its environment? Plan – Which ideas are your best? How will you show people what your bacteria might look like? Reflect & Share - Share your design with others. What’s happening? Bacteria are all around. They can be found almost everywhere! Bacteria are very good at adapting to new environments. That’s partly because they can reproduce so quickly. For example, E. coli can double its population every 20 minutes! Each time an organism reproduces, there is a chance its DNA will change. These changes are called mutations. Sometimes mutations help bacteria to survive. If one bacterium has a helpful mutation, other bacteria may develop it too. In one day, you could have a whole population that can now survive in a new environment. Compare that to humans. It would take many children and many years to spread a mutation in a human population. This is why bacteria have so many different functions and abilities. This is also why we need to keep making new antibiotics. Bacteria can quickly evolve to become resistant to them! This is where the term drug-resistant bacteria comes from. People can help by only taking antibiotics when they really need them. Why does it matter? Some bacteria can harm us, and some bacteria can help us. We use bacteria to make different foods, dye clothing, and break down plastics. And there are so many other things bacteria can do! Have you ever seen the terms probiotics or prebiotics on food packages? Those are foods that help maintain the “good” bacteria in your body to help keep you healthy! There are many “good” bacteria in our bodies that help protect us against “bad” ones. Investigate further! Create a 3D model of your bacterium. Do bacteria like yours exist in real life? Find out! How else do people use bacteria? 17 Ayo Bolaji Computational Biologist Public Health Agency of Canada I was born/grew up in: Saki, Oyo State Nigeria. I lived there until I was 7 years old, then moved to Ibadan, Nigeria. I left when I was 14 for London Ontario, Canada. I now live in: Winnipeg, Manitoba My B.Sc. in Biology was earned at Queen's University in Kingston, Ontario, and my PhD in Cell and Molecular Biology was earned at the University of Sherbrooke, Quebec, Canada. Both degrees provided me with a solid foundation in genetics, cells, and host-pathogen relations. During my postdoctoral fellowship at the University of Manitoba from 2019 to 2021, I became interested in computational biology. I was interested in finding a biological alternative to using fertilizers to safeguard Canadian crops such as canola, soybeans, and maize. I developed the Nanopore sequencing platform as well as data analysis procedures for finding and analyzing the genes of interest in bacteria. What I do at work I work at the Public Health Agency of Canada's National Microbiology Laboratory. I am a Genomics Liaison Officer between the Federal and Manitoba governments. I assist in the monitoring of microorganisms, bacteria and viruses that may have an effect on our health. I also engage in investigations of these organisms. In my day-today work, I use computational biology tools. This includes software, algorithms, and math. These tools help us understand the genomics of pathogens of public health concern. I also make certain that the information gained is communicated to doctors and epidemiologists. I make sure the information is in a form that is easily understood by non-researchers. This is important because the information I provide is used to make public health decisions and policies. My career path I had always known I wanted to be a researcher. But I wasn't sure what kind. During the COVID-19 epidemic, I worked as a computational biologist at the National Microbiology Lab. This allowed me to understand how my skills could be used to help make better public health decisions. Being the only 18 minority in the room was always difficult for me in school. But now, my views and opinions are valued. My research findings have aided in the development of health policies that have been carried out on a national and provincial scale. Today, I consider it a privilege. I am motivated by I like being able to provide light on the nature of a disease and to understand what makes it more contagious than expected. When I figure out complex parts of an organism's genome, this information can be used to drive treatment for patients. It might also help responses to outbreaks. It might even be used to modify public health policies. How I affect peoples' Lives I am also an Adjunct Professor at Providence University College. Here I teach a second-year Genetics course. Because it is a small university, I love the small class size. It is always rewarding for me to watch students grasp complex concepts like gene editing and gene therapy. It’s great to see them plan for a future career in genetics/genomics. References Introduction to Bacteria Arizona State University. (2014, July 3). Microbes: The good, the bad, the ugly. Bailey, R. (2019, August 20). Bacteria shapes. ThoughtCo. Bruckner, M. Z. (n.d.). Gram staining. Carlton College. Davidson, M. W. (2015, November 13). Bacteria cell structure. Florida State University. Humm Kombucha. (2017). The difference between good bacteria and bad bacteria. Microbiology Online. (n.d.). Bacteria. Microbiology Society. Microscope Master. (n.d.). Unicellular organisms. Introduction to Viruses Freudenrich, C., & Kiger P. J. (2020). How viruses work. HowStuffWorks. Howell, A. (2020) Are viruses alive? | Ask A Biologist. Arizona State University. Khan Academy. Are viruses dead or alive? Morgridge Institute for Research. (2020). Virus structure. Open Text BC. (n.d.). The viral life cycle. Wagner, R. & Krug, R. (n.d.). Virus. Encyclopaedia Britannica. Marine Microbiology: Meet the Microbes of the Sea! Australian Institute of Marine Science. (n.d.). Marine microbes. Becker, J. (n.d.). A drop in the ocean is teeming with life. MIT WHOI. Abrahart, E.N. & Stothers, J.B. (n.d.). Dye. Britannica. com. Azman A-S, Mawang C-I, Abubakar S. Bacterial Pigments: The Bioactivities and as an Alternative for Therapeutic Applications. Natural Product Communications. 2018;13(12). http://doi:10.1177/1934578X1801301240 Bernard, J.P. (2018, July 16). A brief history of synthetic dyes. First Source Worldwide, LLC. Celedón, R. S., & Díaz, L. B. (2021). Natural Pigments of Bacterial Origin and Their Possible Biomedical Applications. Microorganisms, 9(4), 739. https://doi.org/10.3390/microorganisms9040739 Chimileski, S. (2017, Nov. 4). Bacterial Dyes in Fashion. American Society for Microbiology. Cratsenburg, E. (n.d.). Natural Colors with Octarine Bio. Ginkgo Bioworks. Lellis, B., Fávaro-Polonio, C. Z., Pamphile, J. A., & Polonio, J. C. (2019). Effects of textile dyes on health and the environment and bioremediation potential of living organisms. Biotechnology Research and Innovation, 3(2), 275–290. https://doi.org/10.1016/j. biori.2019.09.001 Narsing Rao, M. P., Xiao, M., & Li, W.-J. (2017). Fungal and bacterial pigments: Secondary metabolites with wide applications. Frontiers in Microbiology, 8. https://doi.org/10.3389/fmicb.2017.01113 Photosynthesis Education. (n.d.). Photosynthesis in Bacteria. Textile Engineering. (2023, May 7). Synthetic Dyes: Properties, Types, Classification and Application. Weber, H. (2022, May 27). Steal this hot new summer look (it’s bacteria). TechCrunch.com Plastic-Eating Bacteria: Nature's Recyclers Lin, L. Y., & Meighen, E. A. (2009, January 25). Bacterial bioluminescence. Photobiology.info. Ali, S.S., T. Elsamahy, D. Zhu and J. Sun (2023). Biodegradability of polyethylene by efficient bacteria from the guts of plastic-eating waxworms and investigation of its degradation mechanism. Journal of Hazardous Materials 443(B): https://doi.org/10.1016/j. jhazmat.2022.130287 Lindsey, R., & Scott, M. (2010, July 13). What are phytoplankton? NASA. Carpenter, S. (Mar 10, 2021). The Race to Develop Plastic-Eating Bacteria. Forbes. Microbial Life Educational Resources. (n.d.). Microbial life in marine environments. Carleton College. Carrington, D. (Sept 28, 2020). New super-enzyme eats plastic bottles six times faster. The Guardian. Roach, J. (2004, June 7). Source of half Earth's oxygen gets little credit. National Geographic. Castro, J. (April 26, 2014). How Do Enzymes Work? Live Science. Colouring Clothing with Bacteria Cohen, L. (May 11, 2023). Plastic-eating microbes from one of the coldest regions on Earth could be the key to the planet's waste problem. CBS News. Center for microbial oceanography. (n.d.). Marine microbes. Hall, D. (2019) Marine Microbes. Smithsonian Ocean. Aalto University. (2022, Sept. 30). Lab-grown pigments and food by-products: The future of natural textile dyes. Physics.org. Daniell, K. (Apr 16, 2018). Engineering a plastic-eating enzyme. EurekaAlert! 19 Dutfield, S. (Mar 23, 2022). Plastic-eating bacteria: Genetic engineering and environmental impact. Live Science. European Climate, Infrastructure and Environment Executive Agency (August 3, 2023). New LIFE for waste plastic. Geyer, R., J. R. Jambeck and K. L. Law (Jul 19 2017). Production, use, and fate of all plastics ever made. Science Advances 3(7): https://doi.org/10.1126/ sciadv.1700782 Horton, H. (May 10, 2023). Microbes discovered that can digest plastics at low temperatures. The Guardian. Kaur, K., S. Sharma, N. Shree and R. Mehrotra (2023). Recent Advancements and Mechanism of Plastics Biodegradation Promoted by Bacteria: A Key for Sustainable Remediation for Plastic Wastes. Biosciences Biotechnology Research Asia 20(1): http:// dx.doi.org/10.13005/bbra/3063 Morris, A. (Feb 6, 2023) How waste-eating bacteria digest complex carbons. Northwestern Now. Oceana (n.d.). Stop Plastic Pollution: Let’s Work Towards Zero Plastic Waste. Ruthi, J., M. Cerri, I. Brunner, B. Stierli, M. Sander and B. Frey (2023). Discovery of plastic-degrading microbial strains isolated from the alpine and Arctic terrestrial plastisphere. Frontiers in Microbiology 14. https://doi. org/10.3389/fmicb.2023.1178474 Tournier, V., C.M. Topham, A. Gilles, B. David, C. Folgoas, E. Moya-Leclair, E. Kamionka, M.-L. Desrousseaux, H. Texier, S. Gavalda, M. Cot, E. Guemard, M. Dalibey, J. Nomme, G. Cioci, S. Barbe, M. Chateau, I. Andre, S. Duquesne, and A. Marty (2020). An engineered PET depolymerase to break down and recycle plastic bottles. Nature 580: 216-219. Wilkes, R.A., J. Waldbauer, A. Caroll, M. NietoDominguez, D. J. Parker, L. Zhang, A.M. Guss and L. Aristilde (2023). Complex regulation in a Comamonas platform for diverse aromatic carbon metabolism. nature chemical biology 19: 651-662. World Economic Forum (January 2016). The New Plastics Economy: Rethinking the future of plastics. Yoshida, S., K. et al. (Mar 11, 2016). A bacterium that degrades and assimilates poly(ethylene terephthalate). Science 351(6278): 1196-1199. https://doi.org/10.1126/ science.aad6359. 20 Discovering Drugs with the Help of Machine Learning Amgen. (2022, July 6). Generative Biology: Designing Biologic Medicines with Greater Speed and Success. Dill, Ken A et al. The protein folding problem. Annual review of biophysics vol. 37 (2008): 289-316. DOI: 10.1146/annurev.biophys.37.092707.153558 Callaway, E. (2020, November 30). “it will change everything”: Deepmind’s ai makes gigantic leap in solving protein structures. Nature News. https://www. nature.com/articles/d41586-020-03348-4 Beam, A. & Gibson, M. (2019, Nov. 11). The Coming Age of Generative Biology. Flagship Pioneering.