Biol371 Lecture 6: Molecular Aspects of Microbial Growth PDF
Document Details
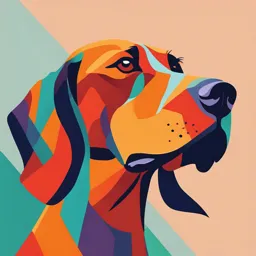
Uploaded by GoldenDeciduousForest
Michael Sgro
Tags
Summary
These lecture notes cover various aspects of microbial growth for Biol371. The material covers bacterial cell growth, the bacterial cell cycle, genome replication, and biofilm formation. The notes include visual aids and diagrams.
Full Transcript
Lecture 6 - Molecular aspects of microbial growth Presented by: Michael Sgro Materials covered: Chapter 8.2-8.5, 8.10-8.12 Bacterial cell growth Binary fission: a cell division following enlargement of a cell to twice its minimum size Generation time: time required for microbial cells to do...
Lecture 6 - Molecular aspects of microbial growth Presented by: Michael Sgro Materials covered: Chapter 8.2-8.5, 8.10-8.12 Bacterial cell growth Binary fission: a cell division following enlargement of a cell to twice its minimum size Generation time: time required for microbial cells to double in number During cell division, each daughter cell receives a chromosome and sufficient copies of all other cell constituents to exist as an independent cell Overview of the bacterial cell cycle Bacteria typically have a circular chromosome. The DnaA protein binds to the origin of replication (oriC) and initiates bidirectional DNA synthesis SeqA binds to hemimethylated DNA and blocks DnaA from binding to the oriC region, preventing additional rounds of replication Genomes segregate to poles of elongating cell Septum formation and cell division Genome replication in fast-growing cells E. coli has a generation time of 20 minutes but it takes 40 minutes to replicate its chromosome. How? Cells growing at generation times less than the replication time contain multiple replication forks (a) Generation time, 1 h ; replication time, 40 min our utes New rounds of DNA replication begin before the last round is complete (b) Generation time, 20 min ; replication time. 40 min utes utes Chromosome segregation Required so daughter cells gets a copy of genome and for septum formation Many bacteria use the Par (partitioning) system to distribute chromosomes and plasmids equally PopZ localizes to old pole of the cell and associates with ParB, which binds to the parS sequence near oriC The parS sequence on the daughter chromosome is bound by ParB and moved to the new pole by the activity of ParA Cell division and Fts proteins The divisome is a cell division apparatus made up of Fts proteins and other proteins essential for binary fission Involved in synthesis of new cytoplasmic membrane and cell wall material (septum) FtsZ forms a ring around the center of the cell ZipA anchors FtsZ to the cytoplasmic membrane FtsA recruits other Fts proteins FtsI helps synthesize new peptidoglycan Cell division and Min proteins DNA replication occurs before divisome formation because nucleoids block formation of the FtsZ ring (nucleoid occlusion) The Min proteins ensure the divisome forms at the center of the cell MinD binds MinC and together they prevent the FtsZ ring from forming. These oscillate between the poles MinE also oscillates, pushing MinCD aside toward the poles MinCD spend most of the time at the poles, allowing the FtsZ ring to form only in the center of the cell Determinants of cell morphology Like eukaryotes, bacteria contain a cytoskeleton to give cells their shape Shape-determining proteins known as SEDS (shape, elongation, division, sporulation) proteins MreB is the major shapedetermining factor in Bacteria MreB recruits other proteins involved in cell wall growth to form the elongasome in rodshaped bacteria Determinants of cell morphology Filaments of MreB move along the cell allowing growth at several points rather than a single plane (FtsZ ring) as in spherical bacteria MreB filaments in rod-shaped cell Crescentin forms filaments which give vibrioshaped cells their curve Crescentin localizes to the concave surface of the cell and is present in addition to MreB Crescentin in vibrio-shaped cells Diverse cell morphologies Diverse morphologies exist in bacteria, especially in the gram-negative Alphaproteobacteria Some synthesize peptidoglycan only at the poles, some grow by budding Morphology and phylogeny are typically unrelated Peptidoglycan biosynthesis Production of new cell wall is a major feature of cell division In cocci, cell walls only grow in opposite directions outward from the FtsZ ring Preexisting peptidoglycan needs to be severed to allow newly synthesized peptidoglycan to form All bacterial cells must synthesize new peptidoglycan and export it outside the cytoplasmic membrane Peptidoglycan biosynthesis Bactoprenol (orange section) is a highly hydrophobic molecule which carries peptidoglycan precursors through the cytoplasmic membrane Lipid II is the entire molecule including bactoprenol bound to the peptidoglycan precursor consisting of N-acetylglucosamine (G), N-acetylmuramic acid (M), and a pentapeptide Pentapeptide Peptidoglycan biosynthesis Removal of extra D-alanine provides energy for transpeptidation Biofilm formation Four stages of biofilm formation Random collision leads to initial attachment, facilitated by structures like flagella or by cell surface proteins Attachment causes expression of biofilm-specific genes encoding proteins for intercellular communication and extracellular polysaccharides Cyclic di-GMP is used as an intracellular signaling molecule. Accumulation triggers a transition from planktonic to biofilm growth Biofilm formation – cyclic di-GMP Two molecules of GTP are converted to c-di-GMP by proteins with GCDEF domains Phosphodiesterases degrade c-di-GMP Many effects including modifications to extracellular polysaccharides and proteins, reduced motility and virulence Biofilm formation – Pseudomonas aeruginosa Pseudomonas aeruginosa is an opportunistic pathogen that forms a tenacious biofilm containing polysaccharides that increase pathogenicity and prevent antibiotic penetration Intercellular communication by quorum sensing is critical for biofilm development and maintenance Accumulation of acyl homoserine lactones (AHLs) signal population growth and high levels trigger c-di-GMP synthesis and activate other biofilm-specific genes DNA released by lysed cells can integrate into polysaccharides and promote biofilm formation A cell lysis protein causes some cells to burst in response to stress Biofilm formation – Vibrio cholerae In Vibrio cholerae, quorum sensing acts in the opposite way as it does in P. aeruginosa Biofilm formation is triggered by low cell densities and repressed by high densities Accumulation of signaling molecules represses biofilm-formation genes and activates flagellar genes Biofilms are more likely to occur in natural marine environment compared to intestinal cells where nutrients are more plentiful. Dispersal aids in transmission to new hosts Biofilm formation – Vibrio cholerae Small regulatory RNA reduces expression of repressor Polysaccharide-binding matrix proteins Antibiotic targets Antibiotics are antibacterial agents (generally) naturally produced by microbes Kill or inhibit bacterial growth Target essential molecular processes Antibiotic targets Many antibiotics target DNA replication, RNA synthesis, or translation Quinolones target DNA gyrase and topoisomerase IV, interfering with DNA unwinding and replication Rifampin and actinomycin prevent RNA synthesis by blocking RNA polymerase active site or RNA elongation Antibiotic targets Inhibition of protein synthesis Ribosomes in bacteria are 70S; eukrayotic are 80S Puromycin binds to A site in 70S ribosome, inducing chain termination Aminoglycoside antibiotics (e.g. streptomycin) target 16S rRNA and 30S ribosome, leading to error-filled proteins that inhibit growth Antibiotic targets Antibiotics targeting the cell membrane Daptomycin specifically binds to phosphatidylglycerol residues of the bacterial cytoplasmic membrane, leading to pore formation, depolarization and death Polymyxins are cyclic peptides whose long hydrophobic tails target the lipopolysaccharide layer, disrupting the membrane and causing leakage and death Antibiotic targets Antibiotics targeting the cell wall Β-lactams (penicillin, cephalosporin, derivatives) interfere with transpeptidation (formation of cross-links) between muramic acid residues Vancomycin binds to pentapeptide precursor and prevents interbridge formation Bacitracin binds to bactoprenol and prevents new peptidoglycan precursors from reaching site of synthesis Antibiotic resistance mechanisms Antibiotic resistance mechanisms are genetically encoded in four classes: 1. Modification of drug target 2. Enzymatic inactivation 3. Removal via efflux pumps 4. Metabolic bypasses Resistance can arise due to random chromosomal mutations E.g. Spontaneous mutants resistant to rifampin can be obtained by exposing a large population to the antibiotic Resistance can also exist on mobile genetic elements and be transferred by horizontal gene flow Many mobile resistance genes encode enzymes that inactivate antibiotic (e.g. Βlactamase cleaves a ring structure, or an acetylating enzyme adds acetyl groups to chloramphenicol) Antibiotic resistance mechanisms Penicillin is shown here as an example for different mechanisms of resistance Mechanisms of resistance shown in green PBP = penicillin-binding protein Antibiotic resistance – Efflux pumps Efflux pumps are ubiquitous and transport various molecules, including antibiotics, out of the cell Lowers intracellular concentration, allowing cell to survive at higher external concentrations Many can act on a broad range of compounds and transport multiple classes of antibiotics, contributing to multidrug resistance E.g. AcrAB-TolC in E. coli can pump out rifampin, chloramphenicol and fluoroquinolones Antibiotic resistance – Metabolic bypasses A metabolic bypass makes the antibiotic target no longer essential E.g. methicillin-resistant Staphylococcus aureus (MRSA) contains an alternative penicillinbinding protein that is not recognized by β-lactams MRSA synthesize this protein only in the presence of β-lactams due to a repressor and a β-lactam sensor Persistence and dormancy Persistence: When a population of antibiotic-sensitive bacteria produces rare cells that are transiently tolerant to multiple antibiotics Persisters are genetically identical but dormant (viable but do not grow) Dormancy prevents antibiotics from killing the cell When treatment is stopped, cells emerge from dormancy and grow Can cause recurring infections (e.g. cystic fibrosis from Pseudomonas aeruginosa) Toxin-Antitoxin (TA) modules Toxin-Antitoxin (TA) modules encode two components: a toxin which inhibits cell growth and an antitoxin that binds and counteracts the toxin Found in almost all bacteria Toxic activity thought to promote cellular adaptation by slowing cell growth to ensure survival during stress Toxin-Antitoxin (TA) modules The hipAB genes encode a TA module In E. coli HipA is a toxin that inhibits translation HipB is an antitoxin susceptible to Lon protease Normally they form a stable, non-toxic complex If Lon is activated by the signalling molecule PolyP, HipB is degraded and growth is arrested by HipA Stringent response HipA phosphorylates glutamyl-tRNA synthetase (GltX), preventing the tRNA synthetase from charging amino acids. This leads to ribosome stalling, inhibiting translation Uncharged tRNA entering the A site of the ribosome causes RelA to produce the alarmone (p)ppGpp, inducing the stringent response pathway Stringent response leads to cell dormancy Exiting dormancy Once antibiotic exposure ends, persisters exit the stringent response pathway and produce HipB (or other antitoxin) again, allowing cells to grow HipA-induced persistence occurs in cells randomly producing higher amounts of the PolyP signal molecule (phenotypic heterogeneity)