DNA Replication Lectures 5-6 BIOL2010 2022-23 PDF
Document Details
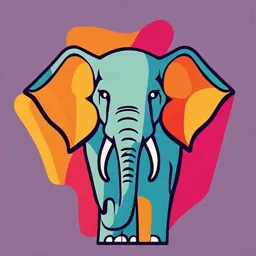
Uploaded by JoyousHawkSEye599
null
Dr M.L.Bellamy
Tags
Related
- Lecture 2.1 Molecular and Cellular Biology (DNA Replication) PDF
- Molecular Biology Instant Notes PDF
- Molecular Biology Lv2 DNA Replication Lecture 4 PDF
- Cell Cycle & DNA Replication Lecture 5 PDF
- Central Dogma of Molecular Biology Lecture Notes PDF
- Fundamentals in Biology 1: From Molecules to the Biochemistry of Cells PDF
Summary
These lecture notes summarize DNA replication, covering its composition, structure, synthesis, problems in E.coli, and specific details like the role of enzymes like polymerase, primase, and helicase. This document aims to help students understand the intricacies of DNA replication, which is a fundamental process in biology.
Full Transcript
BIOL2010 DNA replication Lectures 5-6 Dr M.L.Bellamy Overview 1) Composition/structure of DNA 2) DNA synthesis vs DNA replication 3) Problems and solutions in E.coli 4) The 3 steps in replication in E.coli 5) Differences in eukaryotes/viruses DNA replication...
BIOL2010 DNA replication Lectures 5-6 Dr M.L.Bellamy Overview 1) Composition/structure of DNA 2) DNA synthesis vs DNA replication 3) Problems and solutions in E.coli 4) The 3 steps in replication in E.coli 5) Differences in eukaryotes/viruses DNA replication Problems 4) Mutations/errors in synthesis → Incorrect nt added → RNA nt instead of DNA nt → Nicks in backbone (fragments) DNA replication Incorrect nt addition The incoming dNTP has to form the correct base pair to fit properly into the polymerase active site. → Shape discrimination → Binding affinity → Induced fit DNA replication Incorrect nt addition Shape discrimination Incorrect dNTPs are excluded by steric collisions, even if there are hydrogen bonds possible DNA replication Incorrect nt addition Binding affinity The polymerase checks the fit via the minor groove DNA replication Incorrect nt addition Induced-fit The incoming dNTP causes a conformational change OPEN CLOSED DNA replication Incorrect nt addition Pol III adds the wrong dNTP at a rate of 1 per 100,000 bp (error rate of 10-5) → Would be ~46 point mutations per E.coli chromosome replication → Error rate drops to 10-7 with proofreading activity of polymerases DNA replication Incorrect nt addition Proofreading Pol III has 3’-5’ exonuclease activity →Can remove the last nt added, if it was incorrect A mismatched nt at the 3’ end won’t be in the right place to add the next dNTP → Polymerase stalls The end of the strand becomes substrate for the exonuclease active site, which cleaves the terminal phosphodiester bond, releasing a dNMP DNA replication Incorrect nt addition Proofreading: 3’-5’ exonuclease The shortened strand will re-engage the polymerase active site → synthesis resumes DNA replication Incorrect nt addition DNA Polymerase I (Pol I) An enzyme with multiple activities: 5’-3’ polymerase (synthesis) 3’-5’ exonuclease (proofreading) 5’-3’ exonuclease (nick translation) Treatment with protease produces two fragments: Small N-terminal fragment (5’-3’ exonuclease) Large C-terminal fragment (Klenow fragment) containing polymerase and 3’-5’ exonuclease DNA replication Incorrect nt addition DNA Polymerase I (Pol I) 5’-3’ exonuclease (nick translation) →Can remove and replace nt ahead of it P OH DNA replication RNA in DNA rNTPs can’t be directly incorporated into growing DNA strands, because the extra OH in ribose causes a steric clash DNA replication Nicks Okazaki fragments revisited 5’ 3’ LEADING (1 primer) LAGGING 5’ 3’ Okazaki’s experiments showed the presence of fragments from the lagging strand, but didn’t show the presence of the continuous leading strand – why? → Leading strand also consists of fragments that need to be joined together (pseudo-Okazaki fragments) DNA replication Nicks Leading strand fragments The synthetic pathway for making dTTP, includes dUTP 1 2 3 4 5 6 3 rUM P rUDP d UDP d UTP d UM P d TM P d TDP d TTP 1:300 7 7 DNA Pol III will incorporate dUTP instead of dTTP 1 out every 300 times (once every 1200 nt synthesized) Since U shouldn’t be present in DNA it must be removed→nicks in chain→fragments of ~1200nt If enzyme 4 is mutated→More U & shorter fragments DNA replication Nicks Leading strand fragments Why is U a problem? U accidentally added opposite A → not a problem U formed by deamination of C → mutation G-C G-C G- C Correct G-U G-C A- U Incorrect Presence of U in DNA is therefore offensive as it suggests damage that will lead to a mutation DNA replication Nicks Leading strand fragments U in DNA U Base removed by Uracil-N-glycosylase (ung) Baseless nt recognised and phosphodiester backbone cleaved by AP(apyrimidinic) endonuclease (xthA) ung mutants Nicked DNA, with incorrect nt → No U removal → No fragments/nicks DNA replication Nicks Leading strand fragments Pol I binds to nick, then removes & replaces baseless nt P OH DNA replication Nicks Leading strand fragments Nick removal 5’ 3’ LEADING LAGGING 5’ 3’ DNA ligase seals DNA nicks LEADING LAGGING Overview 1) Composition/structure of DNA 2) DNA synthesis vs DNA replication 3) Problems and solutions in E.coli 4) The 3 steps in replication in E.coli 5) Differences in eukaryotes/viruses DNA replication Steps Initiation→Elongation→Termination 1. How does replication start? 2. How does replication progress? 3. How does replication stop? Initiation Origin of replication Circular chromosomes and plasmids have a single origin of replication (ori) Region of repetitive dsDNA that is rich in A-T Different in different organisms/plasmids e.g. oriC in E.coli chromosome 245bp long Initiation Origin of replication 1) Multiple DnaA products bind to 9bp repeats 2) Use ATP to separate(melt) the duplex at the 13bp repeats DnaA boxes Initiation Priming the forks 3) A DnaC protein binds to ssDNA and loads a DnaB helicase onto one strand, facing →3’. DnaC detaches and helicase moves to fork. 5’ 3’ 3’ 5’ Initiation Priming the forks 4) After ~65 nt have been unwound by the helicases, DnaG primase enzymes bind to them, forming a primosome. 5’ 3’ 3’ 5’ Initiation Priming the forks Primase An RNA polymerase (but not the one involved in transcription) It is self priming, adding RNA 5’→3’ on a 3’→5’ DNA strand (GTA site preferred) RNA primers are ~10 nt in length It has no editing functions (no proof reading) Activity is increased in the presence of helicase and itself (co-operativity) Initiation Primer synthesis 5) Primase synthesizes a ~10nt RNA primer and detaches from helicase. 3’ 5’ 5’ 3’ 3’ 5’ 5’ 3’ Initiation Preparing ssDNA 6) Single stranded binding protein (SSB) binds to exposed ssDNA preventing re-annealing. 3’ 5’ 5’ 3’ 3’ 5’ 5’ 3’ Initiation Preparing ssDNA Single stranded binding protein (SSB) Encoded by ssb gene Forms a tetramer Not sequence specific Leaves bases exposed when bound Binds co-operatively to ssDNA →Proteins at the ends are bound less well and are easier to displace by polymerases Initiation Preparing ssDNA 7) First primer and SSB trigger arrival of the Pol III holoenzyme at the 3’ end of the primer 3’ 5’ 5’ 3’ 3’ 5’ 5’ 3’ Initiation Replisome assembly Pol III holoenzyme 3x Pol III core (αεθ) 3x Tau protein(τ) Clamp Accessory loader proteins (δδ’χψ) Binds SSB Initiation Replisome assembly 8) The clamp loader loads a β clamp onto the DNA. Pol III core binds to the β clamp. 3’ 5’ 5’ 3’ 3’ 5’ 5’ 3’ Initiation Replisome assembly Clamp loader Binds β clamp proteins Transfers the β clamp onto DNA at primer 3’ end ATP hydrolysis detaches loader Initiation Replisome assembly β clamp Encoded by dnaN gene Forms a ring dimer Not sequence specific Binds Pol III core and imparts processivity → Goes from 10s of bp to >50,000 Initiation Replisome assembly 9) Pol III travels to replication fork, synthesizing the leading strand as it goes and displacing SSB As it catches the helicase, a replisome forms. 3’ 5’ 5’ 3’ 3’ 5’ 5’ 3’ Initiation Priming the forks Replisome Pol III holoenzyme Primosome Occupies ~50nm area around the replication fork 5 DNA replication Steps Initiation→Elongation→Termination 1. How does replication start? 2. How does replication progress? 3. How does replication stop? Elongation Replication forks Lagging strand synthesis 1) As helicase unwinds the duplex, primase re-binds and synthesizes a new primer. 5’ 3’ 3’ Elongation Replication forks Lagging strand synthesis 2) A β clamp is added to the primer by the clamp loader. 5’ 3’ 3’ Elongation Replication forks Lagging strand synthesis 3) A Pol III core binds once enough ssDNA has emerged for the β clamp to reach it. 5’ 3’ 3’ Elongation Replication forks Lagging strand synthesis 4) Primer 1 bound, with correct polarity. First Okazaki fragment starts. 3’ 1 5’ 3’ Elongation Replication forks Lagging strand synthesis 5) DNA is pulled by helicase and Pol III. The lagging strand loops out, picking up SSB. 3’ 5’ 1 5’ O NE” 3’ RO MB “T Elongation Replication forks Lagging strand synthesis 6) Okazaki fragment lengthens. Lagging strand loop gets longer. 5’ 5’ 3’ 1 O NE” 3’ RO MB “T Elongation Replication forks Lagging strand synthesis 6) Okazaki fragment lengthens. Lagging strand loop gets longer. 5’ 5’ O NE” 3’ RO MB “T 3’ 1 Elongation Replication forks Lagging strand synthesis 7) First Okazaki fragment finished. Primase re-binds helicase, then adds a second primer. 5’ 5’ 2 3’ 3’ Elongation Replication forks Lagging strand synthesis 8) Pol III core and β clamp detach from DNA, releasing completed fragment. 5’ Primer 2 gets a β clamp. 3’ 2 3’ Looping process repeats for primer 2 Elongation Fragment removal Lagging strand editing Pol I binds the end of the first Okazaki fragment and replaces the RNA with DNA. DNA ligase seals the nick. 5’ 3’ 2 3’ Process repeats for each fragment DNA replication Steps Initiation→Elongation→Termination 1. How does replication start? 2. How does replication progress? 3. How does replication stop? Termination DNA forks Ter and Tus To prevent the forks overshooting there are 23 bp sequences called Ter, which bind the Tus protein. Tus can be displaced by the fork only in the correct direction, otherwise the helicase stalls Termination DNA forks Topoisomerase IV As the forks get within 200bp of each other, there is no longer room for DNA gyrase to bind → +ve supercoiling, which is relieved by Topoisomerase IV decatenating the molecules Initiation Origin of replication Why does initiation only occur once per cell cycle? OriC contains GATC sequences, which are substrates for dam (DNA adenosine methylase). dam methylates N6 of adenine. After replication, only one strand will be methylated CH3 H CH3 CH3 N 5’-GATC N 5’-GATC 3’-CTAG N 3’-CTAG 5’-GATC N CH3 3’-CTAG N6-methyladenine CH3 Initiation Origin of replication Why does initiation only occur once per cell cycle? Hemimethylated GATC sequence binds SeqA protein → Prevents DnaA binding to OriC The GATC sites are methylated by dam very slowly (~13 mins), so newly synthesized dsDNA remains hemimethylated and new initiation is prevented → Binds DNA to the membrane May assist with the separation and movement of each chromosome into its new cell Initiation Origin of replication Why does initiation only occur once per cell cycle? In dam - strains: 1. Hemimethylated plasmids are not replicated 2. Methylated undergo one round of replication 3. Unmethylated are replicated normally Replication of E.coli chromosome takes ~40 minutes, but they can reproduce every ~20 minutes by starting new replication before the last has finished. Overview 1) Composition/structure of DNA 2) DNA synthesis vs DNA replication 3) Problems and solutions in E.coli 4) The 3 steps in replication in E.coli 5) Differences in eukaryotes/viruses DNA replication Eukaryotes Overall similarity to prokaryotes: Use helicases to unwind the duplex and create replication forks Use SSBs to hold the ssDNA apart Use RNA primers for the polymerase/clamp Use various DNA polymerases for synthesis of the new DNA on leading and lagging strands DNA replication Eukaryotes Broad differences to prokaryotes: 1. Occurs in the nucleus, not the cytoplasm 2. More genetic material to replicate (can be four orders of magnitude greater) 3. More than one chromosome (46 in humans) 4. Linear chromosomes (except mitochondrial) 5. Additional packaging (e.g. nucleosomes) DNA replication Eukaryotes More specific differences: 1. Multiple origins per chromosome (10s-1000s) 2. DNA polymerases are much slower: 50 nt/second versus >1000 nt/second 8 hours versus 40 minute replication 3. Polymerases synthesizing the leading and lagging strands aren’t physically linked together 4. No DNA polymerases with 5’-3’ exonuclease 5. Okazaki fragments are much shorter: ~165 nt versus ~2000 nt