BIOL 1P91 Chapter 11 Student 2024 PDF
Document Details
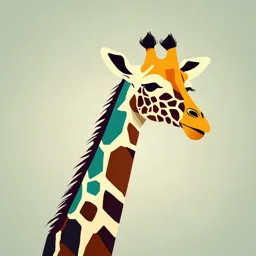
Uploaded by BetterThanExpectedChrysoprase3840
Brock University
2024
Tags
Related
- MgD S4 Lecture 1: DNA Structure and Chromosome Organization PDF
- DNA, Chromosome &DNA Replication Lecture Notes PDF
- BIOC 301 Biochemistry of Nucleic Acids Course Content Sep. 2024 PDF
- Nucleic Acids: DNA to DNA & DNA to RNA PDF
- DNA Structure and Types Lecture Notes PDF
- AQA A Level Biology Nucleic Acids: Structure & DNA Replication PDF
Summary
This document appears to be lecture notes for a biology course, focusing on nucleic acid structure, DNA replication, and chromosome structure. It discusses the chemical nature of genetic material, Griffith's experiment, and the molecular machinery involved in replication.
Full Transcript
Nucleic Acid Structure, DNA Replication, and Chromosome Structure Chapter 11 Chapter 11 Outline Biochemical Identification of the Genetic Material Nucleic Acid Structure Overview of DNA Replication Molecular Mechanism of DNA Replication Molecula...
Nucleic Acid Structure, DNA Replication, and Chromosome Structure Chapter 11 Chapter 11 Outline Biochemical Identification of the Genetic Material Nucleic Acid Structure Overview of DNA Replication Molecular Mechanism of DNA Replication Molecular Structure of Eukaryotic Chromosomes 2 Genetic Material Functions as a blueprint for the construction of living organisms Allows organisms to survive in their environments Must meet the following criteria: Information Replication Transmission Variation ClickBiochemical 11.1 to edit Master text Identification of the Genetic Material 3 Identification of the Genetic Material In late 1800s, scientists postulated that a chemical substance exists within cells that is responsible for the transmission of traits Researchers became convinced chromosomes were the hereditary material Observed to double & divide during cell division Found to contain DNA & protein In 1920s to 1940s, scientists expected that protein would be identified as the genetic material 4 Griffith’s Bacterial Transformation In late 1920s Frederick Griffith was studying two strains of a bacterium called Streptococcus pneumoniae Smooth (S): Secrete a polysaccharide capsule and look smooth when grown on a Petri plate Cause infections that are usually fatal in mice Pathogenic: Able to cause disease Capsule prevents mouse immune system from killing the bacteria Rough (R): Do not secrete capsules and look rough on a Petri plate Unable to cause infection in mice 5 Griffith’s Experiment 6 Transformation Transformed bacteria had acquired the information to make a capsule from the heat-killed cells, then proliferated & killed the mouse The information to make a capsule had been replicated and transmitted to daughter cells Consistent with the formation of a capsule being governed by genetic material Genetic material from dead bacteria had been transferred to rough bacteria & provided these with a new heritable trait (capsule) Griffith did not know the biochemical composition of the “transformation principle” 7 Feature Investigation Avery, MacLeod, and McCarty Followed-up on Griffith’s experiments in the 1940s to determine what substance is transferred from dead type S to live type R bacteria Purified DNA, RNA and protein from S type Only the purified DNA could convert R type to S type Treated DNA extract with DNase, RNase and protease Transformation still occurred following RNase and protease treatments DNase treatment prevented transformation Therefore DNA is the genetic material! 8 9 10 11 Transformation Transformation: A process in bacteria where DNA is taken up from the environment and incorporated into the cell Can be performed naturally by many species of bacteria (including Streptococcus pneumoniae) Can also be induced in the lab using specific conditions 12 Levels of DNA Structure Nucleotides form into a single strand of DNA Two strands form a double helix In living cells, DNA is associated with proteins to form chromatin that is packaged into chromosomes A genome is the complete complement of an organism’s genetic material ClickNucleic 11.2 to edit Master text Acid Structure Nucleotides of DNA Three components: Phosphate group Pentose sugar = Deoxyribose Nitrogenous base Purines Adenine (A) Guanine (G) Pyrimidines Cytosine (C) Thymine (T) Nucleotides of RNA Three components: Phosphate group Pentose sugar = Ribose Nitrogenous base Purines Adenine (A) Guanine (G) Pyrimidines Cytosine (C) Uracil (U) Conventional Numbering System In sugar ring, carbon atoms are numbered 1’ to 5’ 1’ carbon is to the right of the ring oxygen and attaches to the nucleotide Phosphate group is attached to 5’ carbon 3’ –OH group is important in linking nucleotides 16 A DNA Strand Nucleotides are linked by covalent phosphodiester bonds to form a polymer called a strand Strand has a specific directionality Sequence: 5’ – TACG – 3’ DNA Structure In 1953, James Watson and Francis Crick, with Maurice Wilkins, proposed the double helix structure of DNA Key contributions: Rosalind Franklin’s X-ray diffraction results Chargaff’s studies on base composition Linus Pauling’s method of working out molecular three-dimensional structure using ball and stick models 18 Rosalind Franklin’s X-Ray Diffraction Atoms in a substance will scatter X-rays diffracted by DNA onto photographic plate X-rays Pattern represents the arrangement of atoms A repeating structure will produce a diffraction pattern Wet DNA fibers related to the structural X-ray beam arrangement of atoms An X-shaped diffraction is characteristic of a helix DNA Base Composition Erwin Chargaff analyzed the base composition of DNA from many different species Results consistently showed that the amount of adenine is similar to the amount of thymine, and the amount of cytosine is similar to the amount of guanine Chargaff’s rule: Amount of adenine = Amount of thymine Amount of cytosine = Amount of guanine 20 Watson & Crick Pulled together existing knowledge from their colleagues’ experimental approaches Tried several models of DNA structure before finding a model consistent with all known data Published the structure of DNA in 1953 and awarded the Nobel Prize in 1962 21 Structure of the DNA Double Helix Two strands of deoxyribonucleotides form a double-stranded helical structure, with the sugar- phosphate backbone on the outside and nitrogenous bases on the inside The two strands are antiparallel = Opposite directionality Helical structure stabilized by hydrogen bonding between nitrogenous bases of two strands = Base pairing Two hydrogen bonds between A and T, three hydrogen bonds between G and C Base sequences of two DNA strands are complementary 5’-GCGGATTT-3’ 3’-CGCCTAAA-5’ 22 23 Three proposed mechanisms for DNA replication 11.3 Overview of DNA Replication Experiments of Meselson & Stahl In 1958, Matthew Meselson and Franklin Stahl devised experiment to differentiate among the three proposed mechanisms Experimental approach: Grew E. coli in media with 15N (heavy isotope), then transferred to media with only 14N (lighter, common form of nitrogen) Cells were allowed to divide and samples were collected after each generation DNA molecules were separated by density gradient centrifugation Original parental strands would contain 15 N while newly made strands would contain 14N 25 Two strands of a DNA molecule stay together when centrifuged Possible Bands: Band(s) observed Light density depends on mechanism of Intermediate density replication, and number of Heavy density generations in 14N media Results Consistent with a semiconservat ive mechanism 27 Semiconservative DNA Replication During replication, two parental strands separate and each serves as a template for synthesis of new strands New nucleotides are added according to complementary base-pairing rules (AT/GC rule) End result is two double helices with same base sequence as the original DNA molecule Each double helix has one parental strand and one newly made daughter strand 28 Bidirectional Replication DNA replication begins at an origin of replication Base-pairing is disrupted to allow the two strands to unwind Replication proceeds outward from the origin in both directions = bidirectional replication ClickMolecular 11.4 to edit Master text Mechanism of DNA Replication 29 Single Origin of Replication Bacteria 30 Multiple Origins of Replication Eukaryotes 31 Molecular Events of DNA Replication Origin of replication is bound by proteins that unwind the DNA to form a replication bubble Two DNA helicase proteins bind and begin moving in opposite directions to separate the two DNA strands Uses energy from ATP Creates a replication fork in each direction Unwinding of DNA generates tightened coils ahead of the replication fork Alleviated by an enzyme called DNA topoisomerase 32 Molecular Events of DNA Replication After the separation of parental DNA strands by helicase, single-strand binding proteins bind to the single strands and prevent them from re-forming a double helix Ensures that nitrogenous bases of the parental strands are kept exposed until DNA polymerase use them as templates for synthesis of complementary strands 33 DNA Synthesis The enzyme DNA polymerase synthesizes DNA using another strand of DNA as a template Slides along the DNA and covalently links new nucleotides to the free 3’-OH of the last nucleotide Catalysis requires correct hydrogen bonding between incoming dNTPs and template strand Incorporation of a dNTP breaks a high energy bond between two phosphates, which is highly exergonic 34 35 Primers DNA polymerase can only add a new nucleotide to the 3’-OH of an existing nucleotide, which has two consequences for replication: Cannot begin DNA synthesis on a bare template strand DNA synthesis always proceeds in a 5’ to 3’ direction An enzyme called DNA primase initiates DNA synthesis by making a short segment of RNA 10 – 12 nt long = RNA primer Must be removed and replaced with DNA later 36 Leading and Lagging Strands A replication fork moves in a single direction with DNA synthesis occurring for two newly created strands The two template strands have opposite directions New daughter strand must be synthesized antiparallel to its template DNA polymerase can work in the 5’ to 3’ direction only Causes the two daughter strands to be replicated differently Leading strand Lagging strand 37 Leading Strand Synthesis A single RNA primer is made at the origin The primer is extended by DNA polymerase Attaches nucleotides in a 5’ to 3’ direction as it moves forward Synthesis of DNA is continuous from the primer, and makes one long molecule Synthesis occurs in the same direction as fork movement 38 Lagging Strand Synthesis Continuous replication is not possible Direction of synthesis is opposite to fork movement Requires multiple RNA primers Each primer is made near the replication fork rather than at the origin Short segments of DNA are synthesized = Okazaki fragments Fragments are eventually connected to each other to form a continuous lagging strand 39 40 Bidirectional replication from an origin 41 Primer Removal and Replacement A special DNA polymerase removes RNA primers & replaces with DNA e.g. DNA polymerase I in E. coli RNA primer I Binds to 5’ end of RNA primer DNA bases Removes RNA nucleotides in the 5’ to 3’ direction = Exonuclease activity Fills in vacant region with DNA using 3’ end of adjacent DNA 42 DNA Ligase Used after removal of RNA primers and replacement with DNA Connects the newly synthesized DNA to the DNA originally next to the primer by catalyzing the missing phosphodiester bond between bases Used to seal DNA fragments of lagging strand, and on the leading strand after the primer at the origin is removed and replaced Also used to seal together adjacent DNA strands stemming from multiple origins of replication 43 Proteins involved in the synthesis of the leading and lagging strands in E. coli 44 Proteins involved in the synthesis of the leading and lagging strands in E. coli 45 DNA Replication is Very Accurate DNA Polymerase has high fidelity because: Correct hydrogen bonding is more stable than mismatches The active site of DNA polymerase is unlikely to form bonds if pairs mismatched DNA polymerase has proofreading activity Detects when a mispaired base has been incorporated Removes mismatch with 3’ to 5’ exonuclease function Cells also have other repair enzymes that are dedicated to detecting and fixing DNA abnormalities Prevents DNA mutation 46 Your DNA Repair Enzymes are Busy 47 48 Telomeres Regions at the end of linear eukaryotic chromosomes Consist of a short DNA sequence (~6 nt) that is repeated several hundred to a few thousand times in a row Special proteins bind to this sequence Identify telomeres & protect chromosome ends 49 End Replication Problem DNA polymerase can only synthesize in a 5’ to 3’ direction & requires a primer Cannot copy the very end of the lagging strand Even if RNA primer was placed at the very last base, the primer would have to be removed Produces a single-stranded 3’ overhang Causes linear chromosomes to become progressively shorter with every round of DNA replication 50 Telomerase Telomerase is an enzyme that attaches many new copies of the DNA repeat sequence to the ends of chromosomes to prevent chromosome shortening Composed of protein & RNA Only expressed in some cell types! Telomerase binds and synthesizes new telomeric DNA, then moves down & repeats this process Occurs many times to lengthen the 3’ end Longer 3’ overhang provides an upstream site for a new RNA primer to be made & DNA copied by DNA polymerase 51 DNA replication by telomerase 52 53 54