Biol 112 Notes - Google Docs PDF
Document Details
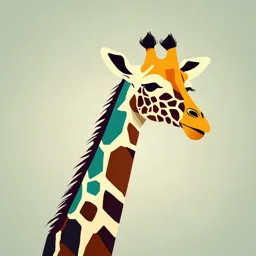
Uploaded by PanoramicGoshenite4959
Tags
Summary
These notes cover cell biology topics, including cell theory, prokaryotic cells, eukaryotic cells, organelles, DNA, RNA, proteins, and metabolism. They provide a basic understanding of the structure and function of various cell types. The document highlights the difference between prokaryotes and eukaryotes.
Full Transcript
WEEK 1 1.3 The Cell ll cells have a plasma membrane. Plasma membrane separates living cell material from A outside environment. Plasma membrane = cell membrane - Boundary between inside living and outside non-living does not mean cells are closed systems or indepen...
WEEK 1 1.3 The Cell ll cells have a plasma membrane. Plasma membrane separates living cell material from A outside environment. Plasma membrane = cell membrane - Boundary between inside living and outside non-living does not mean cells are closed systems or independent from environment. - Active and dynamic interplay between cells and their surroundings - done by plasma membrane - All cells require contributions from surroundings - simple ions and building blocks for macromolecules - Cells release waste - Plasma membrane controls movement of materials in and out of cell Internal membranes - Separate cell into compartments for different functions such as Nucleus Nucleus is where the cell stores its DNA - Nuclear membrane controls movement of materials in and out of nucleus Nucleus has its own space inside the cell called the cytoplasm. Not all cells have a nucleus PROKARYOTE - no nucleus - In our digestive system - Cause disease - salmonella, tuberculosis, etc - SMALL SIZE - REPRODUCE RAPIDLY - Obtain ENERGY / nutrients from DIVERSE SOURCES - Mostly SINGLE-CELLED ORGANISMS - some have SIMPLE multicellular forms EUKARYOTE - has nucleus - Humans, animals, plants, fungi - PROTISTS - single-celled microorganisms - Exist as single cellular organisms AND multicellular - Cells SPECIALIZE within multicellular organisms to perform different functions - Muscle cells contract, red blood cells carry oxygen to tissues, skin cells for extra barrier hree groups of cells: bacteria, archaea, and eukarya T Bacteria and archaea are Prokaryotes- mostly singlecelled and lack nucleus Eukarya are Eukaryotes- mostly multicellular andhave nucleus Many archaea can live in hostile conditions NA - Deoxyribonucleic acid D NUCLEIC ACIDS - Store and transmit information needed for growth, function, reproduction - DNA can be transmitted to other cells through cell division - All living organisms have DNA - Cells must be able to copy their DNA quickly - DNA is double helix. Each strand is made up by 4 types of molecules. - DNA is used as a template to make RNA. specialized molecular structures read the RNA to determine the building blocks needed to make a protein - PROTEINS are molecules that provide structure and do work in the cell - PROTEINS do cells internal architexture, shape, ability to move, chemical reactions, and more - Synthesis of RNA from a DNA is TRANSCRIPTION TRANSCRIPTION - Synthesis of RNA from a DNA template - Copying information from one form into another TRANSLATION - Synthesis of PROTEINS from a RNA template - Converts information (nucleic acids) to proteins Pathway from DNA to RNA (specifically mRNA) is the CENTRAL DOGMA of cell bio CENTRAL DOGMA - Pathway from DNA to RNA (specifically mRNA) - Basic flow of information in a cell and is a fundamental principle of bio GENE - The DNA sequence that corresponds to a functional product like a protein - Proteins are encoded by DNA, can define segments of DNA according to the proteins they encode. NA uses transcription to make RNA. RNA uses translation to make proteins. Proteins do D functions DNA REPLICATION - Allows genetic information to be passed from cell to cell - Each organisms DNA can be passed down generations because of double helix - During replication, each strand of the double helix is a template for a new strand - Replication is very precise/accurate - mistakes can be lethal - Errors in replication is a MUTATION MUTATIONS - Errors in DNA replication - Can end up in death for cell - Can lead to variations that make diversity and evolution Metabolism is the set of chemical reactions that sustain life Energy - Organisms acquire energy from the sun and chemical compounds METABOLISM - Chemical reaction, cells convert energy from one form to another - Build and break down molecules - Required to sustain life - ALL ORGANISMS use chemical reactions to break down molecules - In the process of chemical reactions, ATP (adenosine triphosphate) is released (energy) - ATP provides an accessible form of energy - ATP allows cells to carry out functions - growth, division, moving molecules in/out cell 3.1 Cell Theory Cell Theory 1. All organisms are made up of cells 2. The cell is the fundamental unit of life 3. Cells come from preexisting cells All organisms are made up of cells. - Some unicellular, some multicellular - Multicellular - cells carry out specialized functions The cell is the fundamental unit of life. - Simplest entity that we can define as living - Life: can reproduce, responds to environment, harness energy, evolve - Membranes/molecules are not defined as living - Cell is most basic form of life Cells come from preexisting cells. - Through cell division - Single parent cell produces daughter cells Structure and function of cells is closely related - Structure of RBC allows it to easily pass thru narrow blood vessels + high SA - Structure of neuron allows it to communicate with other cells Prokaryote - Bacteria, archaea - Lack a nucleus - enetic material is in one circular chromosome with many loops G - Genetic material is in theNUCLEOID - Have a cell wall surrounding cell membrane, helps cell keep its shape - Some bacteria have flagella, extend from their surface + allow to move - Prokaryotes aresmall(typically 1-2 um) diameter - High SA to volumeratio - helps absorb nutrients fromenvironment - Not many internal membranes - Translation occurs as soon as mRNA is transcribed from DNA template Eukaryote - Plants, animals, fungi, protists - Have a nucleus - Bigger than prokaryotes - Lots of internal membranes - Nucleus has majority of cells DNA in the form of many linear chromosomes in comparison to single circular chromosome of prokaryotes - Nucleus has more complex regulation of gene expression - Processes in eukaryotes of transcription and translation are separated in space/time - Transcription occurs in nucleus, translation in cytoplasm ORGANELLES - In EUKARYOTIC cells - Internal membranes in cell define compartmentsspecializedfor different functions - Like a factory with many departments - Organelles carry out different functions - important for life of cell - Nucleus is an organelle 24.1 Two Prokaryotic Domains ukaryotic cells (including cells that make up human body) have a membrane surrounded E nucleus & organelles that are in separate compartments for distinct cell function Prokaryotic - Simpler organization - No membrane surrounds cells DNA (like nucleus) - Cell compartments open - no wall Bacteria and archaea are small and not complex structure but can withstand environmental extremes Bacterial cell (prokaryotic) - Small but powerful - Diverse domain of prokaryotic microorganisms - No membrane surrounded nuclei, no energy-producing organelles, no sex - Diverse and extremely successful - NA is in a singular chromosome D - Many bacteria carry additional DNA in the form of PLASMIDS - No nuclear membrane separates DNA from surrounding cytoplasm, transcribed mRNA is immediately translated into proteins by ribosomes - Lack organellesfound in eukaryotic cells - Metabolism andother cell processes are carried outby proteins floating free in cytoplasmor embedded in cell membrane - Photosynthetic bacteria contain internal membranes - Photosynthetic bacteria have light triggered reactions PLASMID - Small circle of DNA that replicate independently of cells circular chromosome - Not essential for cells survival but may contain adaptive genes Photosynthetic bacteria & why are bacteria so small? - Very small (200nm to 2um) - SMALL BECAUSE DIFFUSION - Gain CO2 by diffusion from the environment into cell - Oxidizing bacteria take in small organic molecules & oxygen by diffusion - A small cell has more SA compared to volume - Interior parts of cell are closer to surrounding environment than a larger cell - Slowly diffusing molecules don’t have to travel far to interior - SA of spherical cell (area available for molecules inside cell)increases square of radius - Cells volume (amount of cytoplasm supported by diffusion) increasesas cube of radius - As cell size increases, becomes harder to supply the cell with materials for growth - Most bacteria are spheres, rods, spirals, or filaments - Some bacteria are multicellular but it is rare (form simple filaments or sheets of cells) 12.5 Genome Size and Packaging nowing genome sequences allows us to make comparisons between organisms. K Humans have about the same number of protein coding genes as many organisms with much smaller genomes - Despite having 100 million times as many cells as the worm, we have about the same number of protein coding genes Genes - The expression of protein coding genes can be regulated in many subtle ways, causes different genes to be made in diff amounts at diff times in diff cells - Differential gene expression allows the same protein coding genes to be deployed in different combinations for avariety of cell types - Proteins can interact with each other so they can combine diff ways to perform functions - A single gene may yield multiple proteins - Differentexonsspliced together to make differentproteins - Posttransitional modification - Proteins undergo biochemical changes after theyve been translated Viruses, bacteria, and archaea have small compact genomes - Genomes are measured in numbers of base pairs - 1000b=1kb, 1,000,000b=1Mb, 1,000,000,000=1Gb - Most bacteria and archaea genomes have a defined function - 90% of their genomes have protein coding genes - Bigger genomes have more genes, can synthesize small molecules that other bacteria cannot /have trouble with - Archaea genomes range from 0.5 to 5.7Mb Among eukaryotes, no relationship exists between genome size and organism complexity - Just like # of genes doesnt correlate with organism complexity - Size of genome is unrelated to metabolic, developmental, and behavioral complexity of organism - Disconnect between genome size and organism complexity is C-VALUE PARADOX - C-value is the amount of DNA in a reproductive cell, paradox is contradiction - Difficulty of predicting one based on the other - Genomes of different species can contain vastly different amounts of repetitive DNA GENOME: entire sequence of DNA Why are some eukaryotic genomes so large? - POLYPLOIDY - having more than 2 sets of chromosomes in the genome - Prominent in plants - Humans have 23 chromosomes (46 in total) - Reason for large genomes is they contain large amounts of DNA that do not code for proteins, such asINTRONSand DNA sequences presentin many copies - Complete genome sequencing has allowed scientists to specify diff types of noncoding DNA more precisely - Only about 2.5% of human genome codes for proteins TRANSPOSABLE ELEMENTS: repeated sequences (transposons) - DNA sequences - can replicate and insert themselves into new positions in the genome - Potential to increase their copy number in the genome over time - Transposable elements considered selfish DNA because all they do is duplicate themselves and be a parasite - Make up about 45% of DNA in human genome DNA transposons vs retrotransposons - DNA transposons - Replicate and transpose by DNA replication & repair - About 3% of human DNA consists of DNA transposons - Retrotransosons - By means of an RNA intermediate - RNA is used as template to synthesize complimentary strands of DNA - Retro means backward - More than 40% of human genome consists of various types of retrotransposons Bacterial cells package their DNA as a nucleoid composed of many loops - Regardless of large or small genome, genomes are large relative to the size of the cell - ex. If circular genome of E.coli was fully extended, the length would be 200x the cells diameter - An enormous length of DNA must be packaged into a form that will fit into the cell while allowing DNA to replicate and function - Bacterial genomes are circular. DNA double helix is UNDERWOUND UNDERWINDING - “It makes fewer turns in going around the circle than would allow every base in one strand to pair with its partner base in the other strand” - Caused by an enzyme (topoisomerase 2) that breaks double helix, rotates the ends to unwind the helix, and unwinds it - Creates strain on DNA molecule, relieved by formation of SUPERCOILS - DNA molecule coils on itself - There is alsooverwinding SUPERCOILS - Relieves strain on DNA molecule after being unwound - DNA molecule coils on itself - Allows all base pairs to form, even thou molecule is underwound - Supercoils that result from underwinding are called negative supercoils - Supercoils that result from overwinding are called positive supercoils - In most organisms, DNA is negatively supercoiled - Supercoils of DNA form a structure with multiple loops called a NUCLEOID - Supercoil loops are bound together by proteins - Compresses the DNA molecule into a compact volume enome/Nucleoid of a bacteria/archaea is often called a chromosome. But a eukaryotic G chromosome has a very different structure EUKARYOTIC cells package their DNA as one molecule per chromosome - Have topoisomerase 2 enzymes, DNA is usually negatively supercoiled - DNA in chromosomes is packaged very differently than prokaryotes - Eukaryotic DNA is linear, each DNA molecule forms a single chromosome - In a chromosome, DNA is packaged with proteins to form a DNA protein complex called CHROMATIN CHROMATIN - In a chromosome, DNA is packaged with proteins to form a DNA protein complex called chromatin - Several levels of chromatin packaging - Eukaryotic DNA winds around HISTONE proteins HISTONEproteins - Eukaryotic DNA winds around - Found in all eukaryotes - Interact with any double stranded DNA, no matter what the sequence is - Very similar in different organisms - Proteins from one eukaryote can associate with DNA from another eukaryote - The histone proteins form the core of a NUCLEOSOME - Nucleosome wraps around histone proteins - Rich in positively charged amino acids lysine and arginine, which are attracted to negatively charged phosphates in DNA - If histones removed - DNA spreads out in loops around a supporting protein structure called the CHROMOSOME SCAFFOLD - Each loop of relaxed DNA is long and anchored to the scaffold at its base - Before removal of histones, loops are compact and supercoiled - Each human chromosome contains 2000-8000 loops depending on size NUCLEOSOME - Made up of 8 histone proteins, two of each histones H2A, H2B, H3, and H4 - Each nucleosome includes a stretch of approx 150 nucleotide pairs of DNA wrapped twice around the histone core - Like “beads on a strong” where the nucleosomes are beads and DNA is the string - Also called a10-nm fiber - These are areas of genome that can be activated for transcription 30-nm fiber - Chromatin is tightly coiled, forms a 30-nm fiber - Chromosomes in nucleus condense & prepare for cell division, each chromosome becomes shorter & thicker - 30-nm fiber coils on itself, forms 300-nm coil, then 700nm coil, then 1400nm coil - Progressive packaging makes chromosome condensation, an energy consuming process requiring many proteins ully condensed human chromosome is 5 times larger than the volume of a bacterial cell F The size of the eukaryotic chromosome is greater than the size of the bacterial nucleoid WEEK 2 4 types of macromolecules: lipids, carbohydrates, proteins,nucleic acids 2.2 Molecules and Chemical Bonds OLECULE: Groups of two or more atoms attached together that act as a single unit M CHEMICAL BONDS: When two atoms form a molecule, the bond attracting them & holding them together - Ability of atoms to form bonds with others - Many ways atoms can interact with one another Covalent bonds - Valence electrons - want full outer shell - Two adjacent atoms can share two pairs of electrons - double bond - Molecules most stable when valence shell is full POLAR covalent bond - Unequal sharing of electrons - In a molecule of water, electrons more likely located near oxygen atom - Unequal sharing of electron makes a difference in ability of atoms ability to attract electrons: ELECTRONEGATIVITY - Electronegativity increase L to R across periodic table - As the number of positively charged protons across a row increases, negatively charged electrons are held more tightly to the nucleus - When electrons are shared unequally between 2 atoms: polar covalent bond NONPOLAR covalent bond - Covalent bond between atoms that have the same (or almost same) electronegativity - Ex. H2 - Do not mix well with water Ionic bond - Between oppositely charged ions - Atom of very high and very low electronegativity - High electronegativity atom steals electron - Opposite charges - Nonmetal and metal Chemical reaction - Involves breaking and forming chemical bonds - Atoms keep identity but atoms they are bonded to change 2.5 Organic Molecules Proteins - provide structural support - Act as catalysts to facilitate chemical reactions Nucleic acids - Encode and transmit genetic info Carbohydrates - Provide energy source - Make up cell wall Lipids - ake up cell membranes M - Store energy - Act as signaling molecules - Hydrophobic POLYMERS - Complex molecules made up of repeated simpler units connected by covalent bonds - Proteins are polymers of amino acids - Nucleic acids are polymers of nucleotides - Macromolecules are building blocks of polymers - Rearranging the building blocks that make up macromolecules make diversity - Building blocks of polymers also called subunits or monomers Functional groups add chemical character to carbon chains - Polymers often based on a nonpolar core of carbon atoms - Attached to carbon atoms are FUNCTIONAL GROUPS - Groups of one or more atoms that have their own chemical properties regardless of what they’re attached to - Many functional groups are polar - Molecules containing these groups that would otherwise be nonpolar, become polar - Become soluble - disperse throughout cell - Reactive Proteins are made up of AMINO ACIDS - Proteins do cell work - Some proteins act as catalysts to speed up chemical reactions (ENZYMES) - Some proteins act as structure in cell - for shape and movement - Amino acid chain linked COVALENTly Every amino acid contains: - A central carbon atom (the alpha carbon) - Covalently linked to 4 groups - An amino group (NH2) - A carboxyl group (COOH) - A hydrogen atom (H) - An R group (or side chain) which is different in different amino acids - Glycine has an H side chain, Alanine has a CH3 sidechain mino and carboxyl groups are ionized (charged) (at pH 7.4) A pH of cell is 7.4 Amino acids are linked in a chain to form a protein - C atom in carboxyl group of one amino acid is joined to N atom by a covalent link called a PEPTIDE BOND Dehydration reaction - Formation of a PEPTIDE BOND, loss of a water molecule - Occurs in the linking to form other polymers and complex carbs Proteins are composed of combinations of 20 different amino acids - Each can be classified according to chemical properties of its R group - Sequence of amino acid determines how it folds into its 3D structure - This determines the proteins function Nucleic acids encode genetic information in their nucleotide sequence - Nucleic acids are informational molecules - Nucleic acid = DNA or RNA - RNA is key in protein synthesis & regulation of gene expression - DNA and RNA are long strands of molecules of NUCLEOTIDES bonded covalently Nucleotide is composed of - A 5-carbon sugar (in DNA it is deoxyribose, in RNA it is ribose) - Ribose has a hydroxyl group (OH) on 2nd carbon, while deoxyribose has a hydrogen atom on 2nd carbon (hence the name) - A nitrogen-containing compound called a BASE - One or more PHOSPHATE GROUPS YRIMIDINE BASES: Cytosine, thymine, uracil. P PURINE BASES: guanine, adenine DNA has ATGC RNA has AUGC ach adjacent pair of nucleotides is connected by aPHOSPHODIESTER BOND - forms when a E phosphate group in one nucleotide is covalently joined to the sugar in another nucleotide - Formation of a phosphodiester bond involves formation & release of a water molecule (same as peptide bond) PURINE-PYRIMIDINE PAIRS that are COMPLIMENTARY Successive nucleotides - Along a DNA strand - Can occur in any order ARBOHYDRATES C Complex carbohydrates are made up of single sugars - Sugars from candy are quickly broken down to release energy - Carbohydrates are a major source of energy for metabolism SACCHARIDES Sugars are the simplest carbohydrates - Cyclic molecules (sometimes linear) containing 5-6 carbon atoms - C6 H1 2O6 is glucose, fructose, and galactose. Justarrangement is different MONOSACCHARIDES simple sugars (one) DISACCHARIDES two monosaccharides linked together by a covalent bond - C1 2H22O11 is sucrose that contains one molecule glucoseand fructose POLYSACCHARIDES many sugars - Simple sugars combine in many ways to form polymers - Provide long-term energy storage (starch, glycogen) - Or provide structural support (cellulose in plant cell walls) Complex carbohydrates - Long, branched chains of monosaccharides Monosaccharides - Unbranched carbon chains with either a: - HC=O (aldehyde) or C=O (ketone) - With an aldehyde group is aldoses, ketone group is ketosis - The other carbons each carry one –OH (hydroxyl) and one H atom - When the linear structure of a monosaccharide is written with aldehyde or ketone group at the top, carbons are numbered from top to bottom - Almost always in ring form rather than linear - Are the building blocks of complex carbohydrates - Monosaccharides are attached together by covalent bonds called GLYCOSIDIC BONDS - Involves the release of a water molecule - Formed between carbon 1 of one monosaccharide and a hydroxyl group carried by a carbon atom in a different monosaccharide molecule - Carbohydrate diversity stems in part from the monosaccharides - Some complex carbohydrates are composed of a single type of monosaccharide, while others are a mix of types Lipids - H ydrophobic molecules - Different from proteins, nucleic acids, carbohydrates because they are polymers made up of smaller repeating units with a defined structure - All hydrophobic - Share a property rather than a structure - Chemically diverse - Triacylglycerol- a lipid used for energy storage(vegetable oil, animal fat) - Fatty acid- long chain of carbon atoms attached toa carboxyl group (-COOH) - Glycerol- a 3 carbon molecule with OH groups attachedto each carbon - The carboxyl end of each fatty acid chain attaches to glycerol at one of the OH groups, releasing a molecule of water FATTY ACIDS - long chain of carbon atoms attached to a carboxyl group (-COOH) - The carboxyl end of each fatty acid chain attaches to glycerol at one of the OH groups, releasing a molecule of water - Differ in length of their hydrocarbon chain - # of carbons - Usually an even number of carbon atoms - synthesized by adding 2 carbon units - Sometimes have one or more C-C double bonds - Do not containSATURATED double bonds - No double bonds so the maximum amount of hydrogen atoms is attached to each carbon atom - each carbon atom is said to be “saturated” with H atoms - Fatty acids containing C-C double bonds are UNSATURATED - Chains of saturated fatty acids are straight, unsaturated have a kink at each double bond - Triacylglycerols can contain different types of fatty acids attached to the glycerol backbone - Hydrocarbon chains of fatty acids do not contain polar covalent bonds - Electrons are distributed evenly throughout molecule - Triacylglycerols are extremely hydrophobic - form oil droplets within cell - Efficient form of energy storage because a large # can be packed into small volume - Fatty acid molecules areunchargedbut constant motionof electrons leads to slight positive and negative charges - Attract or repel electrons in neighboring molecules - Set up areas of pos and neg charge in neighboring molecules - Temporarily polarized - weakly bind together due to opposite charges attracting - This is calledVAN DER WAALS FORCES - Weaker than hydrogen bonds - Helps to stabilize molecules - Melting pointsof fatty acids depend onlength andlevel of saturation - slength of hydrocarbon chain increases, # of van der waals forces also A increases, melting temperature increases due to more energy required to break the greater number of van der waals interactions - Kinks introduced by double bonds reduce tightness of molecules and decrease van der waalsinteractions , resulting in a lowermelting point - An unsaturated fatty acid has a lower melting point than a saturated fatty acid of the same length - STEROIDS are a second type of lipid - Ex. cholesterol - Has a core composed of carbon atoms bonded to form four fused rings - Hydrophobic - Cholesterol is in animal cell membrane - Precursor for synthesis of estrogen and testosterone - PHOSPHOLIPIDS are a third type of lipid - Cell membrane 3.2 Structure of Cell Membranes ll cells (prokaryotic and eukaryotic) are defined by membranes. A Membranes - Physically separate cells from environment - Define spaces where cells can carry out functions - Lipids are the main component of membranes - Properties allow to form a barrier in aqueous solutions - Proteins embedded in membrane to transport molecules - Carbs also in membranes Cell membranes composed oftwo layers of lipids - Phospholipids - Glycerol backbone attached to a phosphate group and 2 fatty acids - Phosphate is hydrophilic (polar, loves water) so it forms H bonds with water - 2 fatty acids are hydrophobic (nonpolar) do not form H bonds w water - Molecules with both hydrophilic and hydrophobic regions in a single molecule are AMPHIPATHIC - In an aqueous environment, amphipathic molecules will spontaneously arrange themselves into structures where the polar head groups on the outside interact with water, and the nonpolar tail groups come together on the inside, away from water - Shapes of phospholipids determined by bulkiness of head group relative to hydrophobic tails - MICELLES: lipids with bulky heads and a single hydrophobic fatty acid tail - wedge shaped and make a sphere - ipids with less bulky heads and two hydrophobic tails - rectangular and form a L BILAYER: structure formed of two layers of lipids, tails in between & isolated from aqueous environment - When phospholipids are added to a test tube of water at pH 7, they spontaneously form spherical bilayer structures called LIPOSOMES that surrounded a central space, resembling a cell - In liposomes, the bilayers form closed structures with an open inner space. Free edges would expose hydrophobic chains to aqueous environment - Membranes are self-healing, tears in membrane are rapidly sealed because of nonpolar molecules inside The first cell membranes may have formed spontaneously, capturing macromolecules. - Early cell membranes may have formed spontaneously, capturing macromolecules - Phospholipids naturally form liposomes when placed in water, requiring no enzyme action - Liposome formation depends on - High concentration of phospholipids - pH around 7 to maintain charged hydrophilic head groups - Liposomes can capture macromolecules as they form - In early life, liposomes can form, break, and re-form in environments like tidal flats - Liposomes can grow by incorporating more lipids and capturing nucleic acids and molecules - Early membranes may have been either leaky or impermeable, evolving over time to regulate molecular traffic - Over time, lipid synthesis became protein meditated within cells - Evidence suggests membranes originally formed through simple physical processes - Membrane evolution follows a “tinkering” process, evolving from existing materials rather than being designed from scratch Cell membranes are dynamic - Lipids freely associate due to van der waals forces between fatty acid tails, allowing them to move rapidly within the membrane - A single phospholipid can travel across a bacterial cell in less than a second - Lipids can rotate around their vertical axis and bend, contributing to membrane fluidity - Membrane fluidity depends on lipid composition - Fatty acid tail length: longer tails reduce fluidity by increasing van der waals interactions - C-C double bonds: fewer double bonds reduce fluidity. Saturated fatty acids (no double bonds) pack tightly and limit mobility, while unsaturated fatty acids (with double bonds) create kinks, enhancing mobility - Cholesterol is a major component of animal cell membranes (approx 30% by mass) and its amphipathic nature allows it to insert into the bilayer - At high temperatures, cholesterol decreases membrane fluidity by stabilizing the structure - t low temperatures, cholesterol increases fluidity by preventing tight A phospholipid packing - Cholesterol helps maintain consistent membrane fluidity as temperature changes - Lipids such as sphingolipids can form defined patches called lipid rafts, where specific lipids, cholesterol, and proteins accumulate - Lipids rarely move between the two layers of the bilayer (lipid flip-flop) due to the difficulty of the hydrophobic head passing through the hydrophobic interior - The two layers of the membranes often differ in lipid composition Proteins associate with cell membranes - Cell membranes contain both proteins and lipids - Membrane proteins have different functions (transporters, moving ions, molecules across membrane, receptors, enzymes, anchors, etc) Integral membrane proteins - permanently associated with membranes, cannot be separated from membrane - Transmembrane proteins - Have 3 regions; two hydrophilic (in contact with outside environment) and hydrophobic inside Peripheral membrane proteins - temporarily associated with lipid bilayer thru weak noncovalent interactions - Interact with either the polar heads of lipids or with integral membrane proteins (hydrogen bonds - weak) Proteins often freely move in membrane WEEK 3 3.3 Membrane Transport Phospholipids with embedded proteins make up membrane surrounding all cells ell membrane responsible for maintaining homeostasis C Cell membrane is selectively permeable Hydrophobic interior of lipid bilayer prevents ions and charged polar molecules from moving across it Many macromolecules such as proteins and polysaccharides are too big to cross cell membrane - protein transporters facilitate transportation Passive transport across cell membrane involves diffusion - Concentration gradient - Areas of higher and lower concentrations - Passive transport occurs when molecules move across cell membrane by diffusion - Difference in concentration inside vs outside cell - Some molecules diffuse directly thru cell membrane (simple diffusion) (O and CO2) - any hydrophobic molecules are unable to move thru membrane via simple diffusion M due to the lipid bilayer being hydrophobic - Molecules move passively down a concentration gradient through protein transporters - FACILITATED DIFFUSION - Simple diffusion - molecules move directly through lipid bilayer - Facilitated diffusion - molecules move through protein channels - Some membrane channels are gated - open in response to a chemical or electrical signal - Carrier protein binds to and transports specific molecules Water movement across cell membranes - Via passive transport - Although the central part of the phospholipid bilayer is hydrophobic, water molecules are small enough to move passively via simple diffusion - Water moves through the cell membrane via channel proteins called AQUAPORINS - Allow water to move much more readily across membrane (facilitated diffusion) - This is main way water move thru membrane - Water moves from regions w higher water conc to regions of lower water conc - OSMOSIS - Moves from lower solute conc to higher solute conc - Osmosis continues until conc gradient no longer exists or until movement is opposed thru another force - Osmotic pressuredescribes tendency of solution todraw water in by osmosis Primary active transport uses ATP energy - Active transport - ‘uphill’ movement of substances against a conc gradient - Requires ATP - Cells move through transport proteins embedded in cell membrane (some act as pumps) - Sodium-potassium pump Sodium-potassium pump - primary active transport - Sodium kept at lower conc than external environment - Potass kept at higher conc than external environment - Have to be moved against a concentration gradient - Actively moves sodium out of the cell and potassium in the cell - Uses ATP to carry out Secondary active transport is driven by an electrochemical gradient - Small ions cant cross lipid bilayer - have transport proteins that build up the concentration of a small ion on one side of membrane - Conc gradient stores potential energy - Secondary active transport - Creates a difference in charge - protons positive so higher charge - Called an electrochemical gradient - If protons allowed to pass thru cell membranes by transporter protein, will move down electrochemical gradient toward region oflower protonconc - Movement ofprotons is always high to low - Movement of molecules is driven by protons and NOT ATP directly - Use of an electrochemical gradient as a temporary energy source happens ypertonic solution: solute conc higher than insidecell H Hypotonic solution: solute conc lower than insidethe cell Isotonic: at the same solute conc Many cells maintain size & composition using active transport - When RBC is in hypertonic solution, water leaves cell via osmosis, cell shrinks - When RBC is in hypotonic solution, water moves into cell by osmosis and cell bursts - When isotonic, normal ontractile vacuoles are organelles that take up excess water from inside cell then expel into C external environment Cell wall and cytoskeleton help maintain cell shape - Cell wall - Surrounds cell membrane - Made up of carbs and proteins - Depends on organism - lots of other stuff - Provides structural support & protection - Allows pressure to build up when water enters cell - Force exerted by water pressing against object is TURGOR PRESSURE - Turgor pressure builds as a result of water moving by osmosis into cells with a cell wall - Contain high conc of solutes - In plant cells, vacuole absorbs water and contributes to turgor pressure - Cytoskeleton - Protein filaments - Internal support WEEK 4 5.1 Molecular Structure of Proteins Amino acids differ in side chains - Carbon atom (alpha carbon) - HAS: - Amino group (NH2) - Carboxyl group (COOH) - Hydrogen atom (H) - An R group or side chain - pH from 7.35-7.45 - Amino group gains a proton (NH3+ ) - Carboxyl group loses a proton (COO-) - 4 covalent bonds from ‘a’ carbons are at equal angles - Tetrahedral Hydrophobic amino acids do not want to interact with water or form H bonds Hydrophobic amino acids have nonpolar R groups composed of hydrocarbon chains or uncharged carbon rings Water molecules in the cell form H bonds with each other instead of R groups Asymmetries in electron distribution create temporary charges in interacting molecules Hydrophilic water molecules interact with each other, hydrophobic molecules interact with each other, and this leads to the formation of oil droplets Most hydrophobic amino acids buried in the interior of folded proteins mino acids withpolarR groups have a permanent chargeseparation, where one end of R A group is slightly more negatively charged than the other - amphipathic - R groups are polar. At pH of cell - R groups of basic amino acids gain a proton & become + charged - Acidic amino acids lose a proton and become negatively charged - R groups are charged - usually located on outside surface of folded molecule - Can form ionic bonds with each other & other charged molecules Properties of amino acids important bc of their effect on protein structure - Glycine, proline, cysteine Glycine - R group is Hydrogen - exactly like hydrogen on other side of ‘a’ carbon - Not asymmetric - Nonpolar - Smaller to other amino acids - Freer rotation - Important for folding of proteins All other amino acids have 4 different groups attached to the ‘a’ carbon and are asymmetric Proline - R group is linked back to amino group - Creates a bend in polypeptide chain - Restricts rotation of CN bond - Constraints of protein folding - Opposite of glycine Cysteine - SH group - hen 2 cysteine side chains income into proximity, they can form a SS disulfide bond - W covalently joins side chains - Stronger than ionic interactions of other pairs of amino acids - Can form cross-bridges that can connect proteins Amino acids are connected by peptide bonds Peptide bond - Carboxyl group of one amino acid reacts with the amino acid of the next - A water molecule is released - Resulting molecule - R groups of each amino acid point in different directions On either side of peptide bond: - Carbonyl group C=O - Amide group N-H - Electrons of peptide bond are attracted to C=O more than N-H because of higher electronegativity - Peptide bond is shorter than single bond - doesn’t rotate like a single bond Polymers - Ends are chemically distinct Polymer of amino acids connected by peptide bonds is known as a polypeptide - Polypeptides consist of a few hundred amino acids - In a polypeptide at human pH, amino & carboxyl ends are in charged states of NH3+ and COO- are considered NH2 and COOH The sequence of amino acids dictates protein folding, which determines function - Primary structure- sequence of amino acids in a protein(sequence of amino acid determines how protein folds) - Secondary structures- interactions between stretchesof amino acids in a protein - Tertiary structure- longer-range interactions betweensecondary structures (support the 3D shape of polypeptide) - Quaternary structure- proteins are made up of severalindividual polypeptides that interact with each other unction of protein depends on its 3D shape F When fully folded, proteins contain pockets with + or - charged side chains (can ‘trap’ small molecules) equence of amino acids in a protein (primary structure) is represented by abbreviations S Amino acids in a protein are listed in order Left to Right - starting at amino acids and going to carboxyl end Secondary structures result from hydrogen bonding in the polypeptide backbone - H bonds form between carbonyl group in one peptide bond and the amide group in another - Allows polypeptide chain to fold - Polypeptide backbone is twisted in a coil with 3.6 amino acids per complete turn - Helix stabilized by H bonds between carbonyl (C=O) and amide group (N-H) four amino acids - R groups project outward from the ‘a’ helix - Chemical properties of the R groups largely determine where the ‘a’ helix is positioned in the folded protein ‘b’ sheet- polypeptide folds back & forth on itself - Form a pleated sheet stabilized by H bonds between carbonyl groups in one chain and amide groups in the other chain - Represented by dashed lines - Typically consist of 4-10 polypeptide chains (side by side) - Denoted by broad arrows - Direction of arrow is from amino end of polypeptide to carboxyl end - ‘b’ sheets can be formed by H bonding between polypeptide chains that are parallel - Antiparallel configuration is more stable because carbonyl and amide groups are more favorably aligned for H bonds Tertiary structures result from interactions between amino acid side chains - The tertiary structure of protein is 3D shape of single polypeptide chain - Made up of several secondary structure elements - Defined by interactions between amino acid R groups - Formation of secondary structures relies on interactions in the polypeptide backbone & independent of R groups - Determined by spatial distribution of hydrophilic/hydrophobic R groups along molecule - Determined by diff types of chemical interactions (ionic, H-bonds, van der waals) between R groups - Tertiary structures usually include loops or turns in the backbone so R groups can sit near each other 3D shape of proteins - Ball & stick model - Ribbon model - Space-filling model olypeptide chain is determined by the sequence of amino acids P Primary structure determines the secondary and tertiary structures Tertiary structure determines function because 3D shape of molecule Denaturation - process of molecules unfolding & losing structure - Can be denatured by chemical treatment or high temps - Disrupts H and ionic bonds holding structure together Polypeptide subunits can come together to form quaternary structures - Many proteins do not have quaternary structure, just tertiary Quaternary structure - Two or more polypeptide chains / subunits with a tertiary structure that form quaternary structure - Activity of complex can depend on quaternary structure formed by combination of various tertiary structures - Polypeptide subunits may be identical or different - Many proteins (ex. hemoglobin) composed of two ‘a’ subunits and two ‘b’ subunits - Subunits can influence each other in subtle ways & influence their function Chaperones help some proteins fold properly - Amino acid sequence (primary structure) of a protein determines how it forms its secondary, tertiary, quaternary structures - 75% of proteins, the folding process take place within milliseconds as the molecule is being synthesized he longer that polypeptides remain denatured, the longer hydrophobic groups are exposed to T other macromolecules in the crowded cytoplasm ydrophobic effect brings hydrophobic groups together - may prevent proper folding H Correctly folded proteins can unfold because of high temps Chaperones - Protect slow-folding or denatured proteins until they can get proper 3D structure - Bind with hydrophobic groups and nonpolar R groups to shield them from aggregation - repeated cycles of binding & release - Give polypeptide time to find its correct shape WEEK 5 4.1 Chemical Composition and Structure of DNA Deoxyribonucleic acid is a linear polymer of four subunits A DNA strand consists of subunits called nucleotides tructure relies on nucleotides S NUCLEOTIDES consist of: - 5 carbon sugar - Base - Phosphate groups (1 or more) 5 carbon sugar and phosphate groups form the backbone Each sugar linked to phosphate group of neighboring nucleotide 5’ carbon has negative charges on two of its oxygen atoms - Free hydroxyl groups attached to phosphorus atoms - Ionized by the loss of a proton and is negatively charged DNA is a mild acid - Loses protons in aqueous environment Each base attached to 1’ carbon of sugar and projects above the sugar ring BASES - A, G, T, C PURINES - A and G PYRIMIDINES - T and C NUCLEOSIDE - Combination of sugar and base A nucleoTide is a nucleoside with one or more phosphate groups ucleoside monophosphate, diphosphate, triphosphate is a nucleotide with 1, 2, or 3 phosphate N groups Nucleoside triphosphates are used to form DNA and RNA and carry ATP/GTP DNA is a linear polymer of nucleotides linked by phosphodiester bonds Phosphodiester bond - COPOC bond - Stable bond - Can withstand stresses such as heat & pH changes - Gives the DNA strand polarity Can say 5’-AGCT-3’ or 3’-TCGA-5’ Cellular DNA molecules are a double helix molecules of A = # molecules of T # # molecules of G = # molecules of C ugar phosphate backbones wind around the outside of molecule and bases point inward S 10 base pairs per complete turn - Diameter of molecule is 2nm Strands in double helix are antiparallel - 3’ and of one strand is opposite the 5’ end of the other Each base pair contains a purine and a pyrimidine (A with T and G with C) - Pairing two purines would cause a bulge, two pyrimidines would cause them to narrow Uracil (U) is used instead of T in RNA Why only A&T and G&C? - Hydrogen bonds - Relatively weak but when there is millions they are strong Base stacking - Interactions between bases - Nonpolar flat surfaces of bases group away from water molecules & stack tightly - Stability of DNA 4.2 DNA Structure and Function Double helix shows how chemical structure and biological function come together Number of possible base sequences of a DNA molecule is 133 nucleotides DNA molecules are copied in replication, which relies on base pairing Replication - Enables DNA to pass genetic info from cell to cell & parent to offspring - Enzyme: DNA POLYMERASE - When replication is complete, there are 2 molecules, each containing one parent strand and one daughter strand - Sequence of bases determines that of the other - Two parental and daughter strands are identical sequence - Errors are rare - Errors may be harmful to organism RNA is an intermediary between DNA and protein Enzymes convert energy into usable forms To specify amino acid sequence, uses RNA as intermediary molecule Transcription - copy from DNA to RNA in same nucleic acid language Translation - molecule of RNA is used as a code for the sequence of amino acids in protein - ‘Translation’ of languages from nucleotides that make up nucleic acids to amino acids that make up proteins Gene expression - Transcription and translation are two steps in process - When gene is expressed it is ‘turned on’ and when it is not expressed it is ‘turned off’ - Regulated, does not occur at all times in all cells - Only expressed at certain times and places - Specialized functions All cells in an individual contain the same DNA enetic info using DNA to RNA to protein applies to both prokaryotes and eukaryotes - G processes differ Prokaryotes - Transcription and translation occur in cytoplasm Eukaryotes - Transcription occurs in nucleus - Transcription occurs in cytoplasm - Allows for additional levels of gene regulation that are not possible in prokaryotic cells Only difference between DNA and RNA is that RNA has an extra OH group WEEK 6 4.3 Transcription Eukaryotes - DNA located in nucleus - Ribosomes located in cytoplasm - Therefore must have an intermediary molecule to transfer genetic information from DNA (nucleus) to ribosomes (cytoplasm) - Intermediary is RNA RNA is a polymer of nucleotides where the 5-carbon sugar is ribose RNA is a polymer of nucleotides linked by phosphodiester bonds (similar to DNA) - Has polarity determined by which end of the chain carries the 3’ OH group and which carries 5’ phosphate group DNA vs RNA - RNA - ugar is ribose S - Carries a OH group on 2’ carbon - Less stable - U replaces T - T has a methyl group on 5 carbon while U has a H - 5’ end is triphosphate - Shorter - Single stranded - DNA - ’ end is monophosphate 5 - Double stranded The earliest cells may have used RNA for both information storage and catalysis RNA/DNA can store information in nucleotides Some RNA can act as enzymes to facilitate chemical reactions RNA is involved in replication, transcription, translation RNA played a key role in origin of life In transcription, DNA is used as a template to make complimentary RNA TRANSCRIPTION - DNA unwinds, one string is used as a template for synthesis of RNA transcript (complimentary in sequence except U instead of T) - Transcript produced by polymerization of ribonucleoside triphosphates - Enzyme that carries out is RNA POLYMERASE - Adds nucleotides to 3’ end of growing transcript - Only template strand of DNA is transcribed - Nontemplate strand is not transcribed TRANSCRIPTION PROCESS 1. INITIATION - RNA polymerase and other proteins are attracted to DNA - DNA separated - Transcription begins 2. ELONGATION - Successive nucleotides added to 3’ end of growing RNA transcript - RNA polymerase proceeds along template strand 3. TERMINATION - RNA polymerase encounters sequence in strand that causes transcription to stop - Causes RNA transcript to be released ll nucleic acids are synthesized by addition of nucleotides to 3’ end A Grow in 5’ to 3’ direction DNA template and RNA transcription are ANTIPARALLEL RNA is synthesized 5’ to 3’ direction, DNA is read opposite (3’ to 5’) Transcription starts at a promoter and ends at a terminator PROMOTERS - A few hundred base pairs where RNA polymerase and other proteins bind to DNA - Refers to a region in double sided DNA but transcription is initiated on only one strand TERMINATORS - Transcription stops at the terminator - Transcript is released hen the promoters are in opposite orientation, transcription occurs in opposite directions (can W only proceed 3’ end of transcript) In bacteria, promoter recognition is mediated by a protein called sigma factor - Associates with RNA polymerase and facilitates binding to specific promoters - Once transcription starts, sigma factor dissociates, RNA polymerase continues transcription alone Promoter recognition in eukaryotes - Requires the combined action of at least 6 proteins - General transcription factors - assemble promoter of a gene - Assembly of general transcription factors is necessary but not sufficient - Needs transcriptional activator protein - Each bind to specific DNA sequence (ENHANCER DNA sequences) - TRANSCRIPTIONAL ACTIVATORproteins help control whenand where transcription occurs - Able to bind with enhancer DNA sequences and proteins to begin transcription Presence of transcriptional activator proteins that bind with enhancers controlling expression of gene is required for transcription of all eukaryotic genes to begin Mediator proteins - Once transcription begins, can attract mediator complex of protein, which recruits RNA polymerase complex to the promoter Enhancers can be located anywhere - even far from the gene - Recruitment of mediator complex and RNA polymerase may cause DNA to loop around ells have several types of RNA polymerase enzymes, but in all prokaryotes/eukaryotes they C only use one RNA polymerase adds successive nucleotides to the 3’ end of the transcript nce transcriptional initiation starts, successive ribonucleotides are added to grow transcript - O elongation ranscription takes place in a bubble-type place where two strands of DNA are separated and T growing end of RNA transcript is paired with template strand Bacteria - length of transcription bubble is approx 14 base pairs, length of region of paired RNA-DNA is approx 8 base pairs The RNA polymerase complex is a molecular machine that opens, transcribes, and closes DNA 4.4 RNA Processing Primary transcript - RNA transcript that comes off template DNA strand - Contains complement of every base that was transcribed from DNA template - Includes info needed to direct ribosome to produce protein corresponding to gene mRNA (Messenger RNA) - the RNA molecule that combines with the ribosome to direct protein synthesis (carries message from DNA to RNA) Primary transcripts in prokaryotes are translated immediately Prokaryotes- Transcription and mRNA - Relation between primary transcript and mRNAismRNA - Even as 3’ is being synthesized, ribosomes bind with special sequences near 5’ end and begin protein synthesis - Have no nucleus so transcription/translation are not spatially separated - Processes are coupled - Connected in space and time - Often contain genetic info for synthesis of two or more different proteins - POLYCISTRONIC mRNA Primary transcripts in eukaryotes undergo several types of chemical modification Eukaryotes - Envelopes surrounding nucleus is a barrier between transcription and translation - Transcription in nucleus - Translation in cytoplasm - Allows for complex chemical modification of primary transcript - RNA processing - Converts primary transcript into finished mRNA - Can be translated by ribosome RNA Processing - 3 types of chemical modifications - 5’ end of primary transcript is modified by addition of special nucleotide - Unusual linkage - 5’ cap - Consists of modified nucleotide called 7-methylguanosine - An enzyme attaches modified nucleotide to 5’ end of primary transcript backward - 5’ cap is essential for translation in eukaryotes because ribosome recognizes mRNA by its 5’ cap - otherwise the ribosome would not attach to mRNA and translation wouldn’t occur - Polyadenylation - Addition of a string (approx 250 ribonucleotides) to 3’ end - Forms poly(A)tail - Export of mRNA into cytoplasm - Both 5’ cap and poly(A)tail stabilize RNA transcript - Removal of noncoding introns - Joining exons and removing introns is called RNA SPLICING - Catalyzed by a complex of RNA and protein (SPLICEOSOME) Not every stretch of RNA transcript is translated into protein ranscripts in eukaryotes often contain regions of protein coding sequence that are expressed T (EXONS) or interspersed (INTRONS) Introns - Important for gene expression - 90% of human genes contain at least one intron (most 6-9, largest is 147) Multiple introns - Allows for process calledalternative splicing - Primary transcripts from the same gene can be spliced in different ways to yield different mRNAs and different protein products - More than 80% human genes are alternatively spliced ome noncoding RNA transcripts are processed differently from protein coding transcripts and S have functions of their own ot all primary transcripts are processed into mRNA N Some RNA transcripts have functions on their own rRNA (ribosomal RNA) - Eukaryotic cells, genes and transcripts for rRNA are concentrated in nucleolus - Nucleolus is a non-membrane-bound structure inside the nucleus tRNA (transfer RNA) - Carry individual amino acids for use in translation snRNA (small nuclear RNA) - Essential part of spliceosome required for RNA processing mall regulatory RNA molecules that can inhibit translation or cause destruction of RNA S transcription is miRNA (micro RNA) and siRNA (small interfering RNA) 5.2 Protein Synthesis tructure of protein determines function S Diversity of tertiary and quaternary structures explains wide range of functions Primary structure governs structure Translation uses many molecules found in all cells ell uses ribosomes (made up of RNA and protein), bind with mRNA and are the site of C translation Prokaryotes: occurs as soon as mRNA comes off DNA template Eukaryotes: transcription and translation physically separated (nucleus and cytoplasm) ibosome contains small subunit and large subunit (includes 1-3 types of ribosomal RNA and R 20-50 types of ribosomal protein) Eukaryotic ribosomes are larger than prokaryotic ribosomes Aminoacyl (A) site, peptidyl (P) site, exit (E) site When mRNA is on ribosome and there are nonoverlapping groups of three nucleotides very 3 adjacent nucleotides is a CODON E Each codon in mRNA codes for a single amino acid in polypeptide chain hree bases in the ANTICODON make up the ANTICODON LOOP T Anticodon is the base pair with corresponding codons very tRNA has nucleotide sequence CCA at 3’ end E The 3’ hydroxyl of the A site is the attachment site for the anticodon corresponding to the amino acid nzymes calledaminoacyl tRNA synthetases connectspecific amino acids to specific tRNA E molecules Most organisms have one aminoacyl tRNA synthetase for each amino acid nzyme binds to multiple sites on any tRNA that has an anticodon corresponding to the amino E acid - Formation of covalent bond between amino acid and tRNA tRNA without amino acid is uncharged tRNA with amino acid is charged - Very accurate and specific, do not attach to the wrong amino acid Codon - anticodon interactions The genetic code shows the correspondence between codons and amino acids odon AUG specifies to Methionine (Met) by base pairing with the anticodon of a charged tRNA C (tRNAMet) Most codons specify an amino acid according to a genetic code Three stop codons: UAA, UAG, UGA - Where translation terminates and protein is released from ribosome More codons than amino acids, many amino acids are specified by more than one codon Initiation codon is AUG olypeptide is synthesized from amino end to carboxyl end P Met forms the amino end of any polypeptide being synthesized - Met is often cleaved off by an enzyme after synthesis is complete AUG codon is not translated Initial Met creates amino acid of polypeptide chain Downstream codons are read one by one, nonoverlapping Ribosome binds to a tRNA with an anticodon that can base pair with codon Amino acid on tRNA is attached to growing chain to become new carboxyl end of polypeptide chain Translation consists of initiation, elongation, and termination initiation - AUG is recognized, Met is established as first amino acid Elongation - successive amino acids added one by one to chain Termination - addition of amino acids stops, completed polypeptide chain is released from ribosome One group of initiation factors binds to the 5’ cap and is added to mRNA during processing - ometimes recruit small subunit of ribosome, other times bring up a transfer RNA S charged with Met hen AUG codon is read, a large ribosomal subunit joins the complex and initiation factors are W released tRNAMet bound at P site of ribosome Next tRNA in line binds to A site Once tRNA is in place, reaction happens and the amino acids are connected A RNA in large subunit is catalyst The new polypeptide becomes attached to tRNA in A site Uncharged tRNAmet shifts to E site, released fromribosome, shifts to P site This empties A site, available for next tRNA in line Process requires energy - proteins called elongation factors - Bound to GTP molecules and break high energy bonds to provide energy ermination occurs because stop codons do not have corresponding tRNA molecules T When ribosome encounters a stop codon, protein release factor binds to its A site Release factor causes bond to break, creating carboxyl terminus of polypeptide, then is released Small and large ribosomal subunits disassociate from mRNA and from each other Elongation and termination is similar between prokaryotes and eukaryotes Initiation in eukaryotes - Initiation complex forms at 5’ cap Initiation in prokaryotes - mRNA molecules have no 5’ cap - Formed at one or more internal sequences present in mRNA (shine-dalgarno sequence) - Ability to initiate translation internally - allows to code for more than one protein - Each shine-dalgarno sequence can be an initiation sequence Operon - Type of gene translation Transfer RNAs may originally have served in RNA synthesis 12.2 Recombinant DNA and DNA Editing ecombinant DNA is the basis of genetically modified organisms R DNA editing can be used to alter gene sequences - Can make use only of existing DNA sequences - Many techniques to alter nucleotide of almost any gene CRISPR - Used to alter the nucleotide sequence of almost any gene in any cell - Introduce at different points three types of molecules into a cell - A guide RNA that is complimentary to target DNA - A gene for a protein that cleaves DNA when associated with guide RNA - A piece of DNA that acts as a template - Target DNA identified by guide RNA, cleaved by protein, and replaced with sequence 17.0 Genetic and Epigenetic Regulation raits inherited such as height, weight, diabetes, high blood pressure are influenced by multiple T genes that interact with one another, as well as lifestyle factors Hundreds of genes contribute to adult height ertain genes only expressed in response to signals C Gene regulation encompasses the ways the cell controls gene expression ene regulation can occur in almost any step from DNA to mRNA to protein or after the protein G is made Each event in the expression of a gene is a potential control point 17.1 Transcriptional Regulation in Prokaryotes(bacteria) In prokaryotes, transcription and translation are not physically separated Transcriptional regulation: whether or not transcription occurs - can be positive or negative Positive regulation - Regulatory molecule (usually protein) must bind to the DNA near the gene for transcriptionto occur Negative regulation - Regulatory molecule (usually protein) must bind to the DNA near the gene for transcriptionto be prevented Most promoters contain one or more short sequences that help recruit proteins to do this - TATA box (25-35 base pairs upstream) located here Positive regulation - Regulatory molecule (usually protein) must bind to the DNA near the gene for transcription to occur - Includes DNA, RNA pol, and transcriptional activator protein (activator) - DNA has two binding sites - One for activator, one for RNA pol - When activator is present and can interact with DNA binding site, RNA pol is recruited to promoter and transcription occurs - Ability of repressor to bind DNA is determined by allosteric interaction with a small molecule Negative regulation - Activator not presentor is not able to bind withDNA, RNA pol is not recruited to promoter and transcription does not occur Repressoris present - turns off transcription - Binding site of repressor can be upstream, downstream, or overlapping with promoter LLOSTERIC EFFECT: Sometimes activator combines with a small molecule that alters its A shape and binding ability - Sometimes activator cannot bind with DNA, but combining with the small molecule changes its shape and allows it to bind, resulting in transcription - Typically only encodes proteins that are needed only when small molecule is present in cell - A small molecule that interacts with the repressor and prevents it from binding DNA and blocking transcription is called aninducer Inducerinteracts with repressor and prevents it frombinding DNA and blocking transcription mall molecule often changes the conformation of the repressor so it can bind to the repressor S binding site and prevent transcription - Genes like this are often needed to synthesize the small molecule - In e. Coli, protein for synthesis are negatively regulated by the same gene. When that gene is present in sufficient amounts, it binds with a regulatory protein to form functional repressor, and transcription of the genes doesn’t occur - When the level of tryptophan drops too low, transcription is initiated Lactose - Lactose consists of one molecule of both glucose and galactose - An enzyme cleaves lactose, releasing glucose and galactose - Both molecules can then be broken down and used as a source of carbon and energy - Enzyme only present in presence of lactose or molecules that are chemically similar - Lactose leads to an expression of the gene for the enzyme Lactose - structural genes - LacZ - Is the gene for enzyme beta-galactosidase - Cleaves lactose molecule into glucose and galactose - Nonmutant form is LacZ+ - LacY - Is the gene for protein lactose permease - Transports lactose from external into the cell - Nonmutant form is LacY+ - LacA - Is the gene for enzyme transacetylase - Not required for breakdown of lactose Bacteria containing LacZ– mutants or LacY–mutantscannot use lactose as energy LacZ+ and LacY+ are essential for using lactose andcell growth Without LacY, lactose cannot enter the cell Without LacZ, it can’t be broken down LacZ is the protein product, LacZ+ is the ability to make the protein LacI - repressor protein - Regulates lacZ and lacY LacP - promoter protein - Located between lacI and lacZ - Recruit RNA polymerase to initiate transcription LacO - operator protein - Binding site for repressor - CRP also does this acZ and lacY are transcribed together L Typically a group of related genes are next to one another on DNA and share a promoter, then are transcribed together into a single molecule of mRNA (polycistronic mRNA) PERON- region of DNA consisting of promoter, operator,and coding sequence for O polycistronic mRNA - Found in bacteria and archaea In a polycistronic mRNA, each coding sequence is preceded by a ribosome binding site so translation can be initiated at each coding sequence The lactose operon isnegatively regulatedby a proteinencoded by the lacI gene - The genes oflactose operon are always expressed unlessthe operon is turned offby a repressor or regulatory molecule - LacI gene (encodes repressor protein) is ALWAYS expressed at a low level - The repressor protein binds with the operator lacO, the RNA pol is not recruited, and transcription does not occur - When lactose is present, repressor protein cannot bind to operator, RNA pol is recruited, transcription occurs - Lactose acts as an inducer of the lactose operon(prevents binding of repressor protein) - Said inducer is not always lactose itself, but an isomer too (allolactose) - Lactose in cell is always accompanies by allolactose - Binding of inducer to repressor results in allosteric change in repressor structure - Inhibits protein ability to bind to operator - Absence of repressor from operator allows RNA pol to produce polycistronic mRNA Constitutive mutant- gene that produces product constantly,without regulation actose operon is positively regulated by CRP-cAMP L Ability of repressor to bind with either operator (in absence of lactose) or inducer (in presence of lactose) CRP-cAMP is a protein that activates gene expression when binding DNA 11 DNA Replication and Cell Division ells come from preexisting cells C Multicellular organisms begin life as a single cell - then divide 11.2 DNA Replication efore division, DNA must be replicated so each daughter cell receives genetic info from parent B cell DNA replicates semiconservatively NA double helix unwinds and separate into single strands at a site calledreplication fork D Each individual parental strand is a template strand for the synthesis of a daughter strand - Semiconservative replication Then each new DNA molecule consists of one strand that was originally part of the parental molecule and one newly synthesized strand Conservative replication - original DNA molecule intact, daughter DNA is completely new DNA replication involves many enzymes NA replication begins atreplication fork(whereparental strands separate) D EnzymeDNA helicaseseparates the strands of the parentaldouble helix - Breaks down H bonds holding base pairs together Single stranded binding proteinbinds to single strandedregions - Prevents single stranded regions from coming back together Enzymetopoisomeraseworks upstream from replicationfork - Relieves stress from unwinding - ind or unwind DNA to help relieve stress that occurs during replication and W transcription - Type 1 topoisomerases cut one strand of DNA - Type 2 topoisomerases cut both strands of DNA - Type 2topoisomerase works upstream from replicationfork and relieves stress on double helix by unwinding cut strands in the opposite direction from how they are unwound at replication fork EnzymeRNA polymerasedoes two things: - There are many RNA pol enzymes, same basic function - Attach nucleotide to another nucleotide - Each new DNA strand begins with a primer (short stretch of RNA) for DNA synthesis. Made by RNA primase - EnzymeRNA primase makes short piece of RNA complementaryto DNA parental strand (RNA primer) - Can onlyadd nucleotides to 3’ end of another nucleotide - At 5’ and is phosphate group, at 3’ end is hydroxyl group - DNA synthesis occurs when the 3’ hydroxyl group attacks the 5’ phosphate group of incoming nucleotide - DNA synthesis occurs only in 5’ to 3’ direction - As incoming nucleotide triphosphate is added to growing DNA strand, one of nucleotides phosphate bonds is broken, providing energy for reaction - Outermost two phosphates (pyrophosphates) are released in the process to provide energy for the reaction In replicating DNA, one daughter strand is synthesized continuously and the other in a series of short pieces Since DNA is antiparallel and can only be elongated only at 3’ end, daughter strands are synthesized differently eplication fork moves along, creates region of single stranded DNA R RNA primer is laid down. RNA pol takes over. Daughter strand of replicating DNA molecule has 3’ end pointed toward replication fork so as parental strand unwinds, nucleotides can be added onto 3’ end.Daughter strand synthesized as one longcontinuous polymer. This is the leading strand ther daughter strand synthesized differently O 5’ end is near replication fork, but strand cannot grow that direction Replication fork moves left to create single stranded region of parental DNA Synthesis of daughter strand is initiated on this single stranded region with its 5’ near the replication fork. Elongation of new strand occurs at 3’ end. Daughter strand growsaway from replication fork instead of toward it As parental double helix unwinds, new RNA primer is laid down, which is extended by DNA pol until it reaches the piece in front of it This daughter strand is synthesized in short discontinuous pieces. Called the lagging strand ecause RNA pol complex extends an RNA primer, all new DNA strands have a short stretch of B RNA at 5’ end. For lagging strand, there are many primers, one for each of the discontinuous fragments of new DNA When growing fragment comes into contact with primer of fragment synthesized earlier, a different DNA pol complex takes over, removes RNA primer, extends growing fragment with DNA nucleotides to fill space left Replacement is completed, adjacent fragments are joined (or ligated) byDNA ligase DNA pol complexes for each strand stay in contact with each other - Synthesis of leading and lagging strands is coordinated to occur at the same time and rate - Lagging strands polymerase releases and retrieves lagging strand for synthesis of each RNA primer - Positioning of polymerases is so the leading strand and lagging strand pass through in the same direction, so the lagging strand has to be looped around. - Ensures no strand outpaces the other DNA polymerase is self correcting because of its proofreading function - Separate from elongation (synthesis) When each new nucleotide comes in line for prep for attachment to growing DNA strand, nucleotide is temporarily held in place by H bonds that form between base in new nucleotide and base of template strand ery rarely an incorrect nucleotide is attached. DNA pol can correct this because it detects V mispairing Mispairing activates a DNA cleavage function of DNA polthat removes incorrect nucleotide and puts in correct one This highly reduces errors 11.3 Replication of Chromosomes asic steps of semiconservative DNA replication are universal but replication of chromosome B gives challenges Replication of RNA in chromosomes starts at many places simultaneously NA synthesis is initiated at theorigin of replication D The opening of the double helix at each origin of replication site forms areplication bubble Replication bubble has a fork on either side, each with a leading and lagging strand with topoisomerase 2, helicase, and single stranded binding protein DNA synthesis begins at replication fork. Forks move in opposite directions, replication bubble increases in size. When two replication bubbles meet, they fuse to form one large replication bubble hen two replication bubbles fuse and the leading strand from one meets the lagging strand of W the other,the ends of the strands meet and are joinedby DNA ligase or circular DNA F Some DNA molecules are small circles, not long linear molecules. They only have one origin of replication. Replication takes place at both replication forks. Replication forks proceed in opposite directions around circle and fuse on opposite side 6.0 intro Need to harness energy from environment 6.1 An overview of metabolism Organisms can be classified according to their energy and carbon sources rganisms that capture energy from sunlight are PHOTOTROPHS (such as plants) O Use sunlight to convert CO2 and water into sugar and oxygen - sugars (glucose) can then be used to make ATP Sunlight provides energy to make glucose, then use that to make ATP HEMOTROPHS - organisms that get energy directly from chemical compounds (animals) C Ingest other organisms, obtain glucose that break down into oxygen CO2 and water. Energy of chemical bonds of organic molecules converted to energy carried in bonds ATP UTOTROPHS - self feeders (plants) A Plants are autotrophs and phototrophs so they’re PHOTOAUTOTROPHS ETEROTROPHS - organisms eat other organisms H CHEMOHETEROTROPHS HOTOHETEROTROPHS - gain energy from sunlight but get carbon from ingesting organic P molecules HEMOAUTOTROPHS - take energy from inorganic molecules but build own organic C molecules - often found in extreme environments Metabolism - breaking down glucose (or other) and making useable energy atabolism - chemical reactions that break down molecules into smaller units and produce ATP C Anabolism - chemical reactions that build molecules from smaller units and require energy input (usually ATP) Catabolism - break down nabolism - build up A Cycle - macromolecules -> subunits -> macromolecules Ie. protein -> amino acids 6.2 Kinetic and Potential Energy Chemical energy is potential energy - Energy stored in bonds between atoms in molecule ost stable = lower potential energy M Strong bonds do not need a lot of energy to exist (low potential energy) TP is readily accessible form of energy A Energy stored in bonds connecting phosphate groups 6.3 Laws of Thermodynamics nergy is conserved E Energy transformations always result in an increase in disorder in the universe - ‘Disorder’ being entropy ntropy E Entropy increases, number of positions and motions available to molecule Gas - given more space, molecules less constrained, move more freely, more entropy GAS HAS MOST ENTROPY, solid has least entropy Chemical reactions - entropy occurs in release of thermal energy (heat) Catabolic reactions result in increase in entropy - Single molecule broken down into smaller ones, more freedom to move around Anabolic reactions result in decrease in entropy - Synthesise larger molecules (proteins, nucleic acids) 6.4 Chemical Reactions ells break down glucose, produce CO2 C CO2 converted into carbonic acid (animals) In aqueous environment (blood), carbonic acid exists as HCO3- and protons H+ Readily reversible - carbonic acid -> CO2 and water and vise versa t equilibrium, rate of forward reaction = rate of reverse reaction, [conc] of reactants/products A don’t change Increase [react] or decreasing [prod], favours forward reaction aws of thermodynamics determine whether a reaction requires or releases energy available to L do work mount of energy available to do work is Gibbs free energy A Compare free energy of reactants and products to determine whether the reaction releases Gibbs free energy (delta G) If products have more free energy than reactants, delta G is positive, energy is required to drive reaction forward If products have less free energy than reactants, delta G is negative, energy is released and available to do work XERGONIC- negative delta G (release energy) (spontaneous) E ENDERGONIC- positive delta G (require energy) otal energy = energy available to do work + energy not available to do work (because of T increase in entropy) otal energy (enthalpy) (H) = energy available for work (Gibbs)(G) + energy lost to entropy T (temp*entropy)(TS) G = H-TS The hydrolysis of ATP is an exergonic reaction (exothermic) DP is