Master 1-Bioeco: Update on Microbial Physiology-Metabolism-Cellular Regulation PDF
Document Details
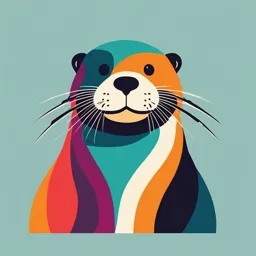
Uploaded by FlatteringSatire8869
Tags
Summary
This document provides an update on microbial physiology, metabolism, and cellular regulation. It covers topics like cell biology, energetics, and transport, and includes diagrams and figures to illustrate the concepts. The document is suitable for undergraduate-level biology courses.
Full Transcript
MASTER 1-BIOECO Update on Microbial physiology-Metabolism- cellular regulation Part 1: Cell biology Part 2: Physiology- metabolism Part 3: cellular Energetic & transport Part 4: cellular Regulation The cell: 6 functions are require...
MASTER 1-BIOECO Update on Microbial physiology-Metabolism- cellular regulation Part 1: Cell biology Part 2: Physiology- metabolism Part 3: cellular Energetic & transport Part 4: cellular Regulation The cell: 6 functions are required 2 Living systems kingdom Animaux Plantes Champignons Flagellés Ciliés Microsporidies Amibes Eucaryotes Diplomonadines Bactéries Archébatéries Mitochondries Gram positives Eubactéries Crénotes Protéobacteries Flavobacteries Cyanobactéries Euryotes Chloroplastes Bactéries vertes Aquificales non sulfureuses - établi par comparaison des séquences des rRNA Thermotogales - adapté de G.J. Olsen et C.R. Woese, « ribosomal RNA a key to phylogeny » FASEB Journal , 7: 113-123, 1993 The cell: general function Growth temperature pH of growth acidophile neutrophile alkalilophile Specific growth rate yeasts bacteria bacteria yeasts 1 3 7 9 11 pH Cellular aims Two aims: - to maintain in life - to reproduce Cellular composition of a common cell: 70-90 % of water 30-10 % dry mass of C = 50 % O = 20 % N = 14 % H=8% P=3% + salt (Mg, K, Na, Ca) < 3 % + oligo(trace)-elements (Fe, Ni, Co, Cu, Mg, Mn etc) + some additional needs vitamins growth factors pH: inside cells 6 -7.5/ outside; 2 -10 temperature: 4 to 80°c Basic requirement for a Culture medium Chemically defined or mineral medium i.e. knowledge of all components in g/l , use for quantitative physiology for prototrophic growth of microbes Water Glucose Ammonium sulfate K-Phosphate K2SO4 Few trace elements (can be obtained if use tap water) Adjust pH between 4 to 7 , according to the microbes (with K-phosphate) Complex medium Yeast extract/ peptone/sugar, use for basic microbiological test and optimal growth for strain that are auxotrophic for amino acids, bases , vitamines Metabolic process in a biological system Anabolism Catabolism Electron donors biomass i.e. glucose Electron acceptors i.e. oxygen, nitrate, sulfate ATP/ADP +Pi NAD(P)H /NAD(P)+ C Chemical formulae of a living system O - Yeast: C:H1.613:O0.557:N0.158 -> Mw= 26,01 g/mol N - E.coli: C:H1.77:O0.49:N0.24 -> Mw = 25,95 g/mol H sources Oxidixed donors Reduced acceptors Ex: glucose + O2 + NH3 + cell CxHyOzNw + H2O + CO2 the carbon mass and redox balance must be equilibrated Overview of the cellular metabolism 12 precusors metabolites are needed to construct the new cells Glycolysis: Glucose-6P glycosylation/ cell wall Fructose-6P glycosylation/ PEP amino acids (aromatic) Pyruvate amino acids (C3) Dihydroxyacetone –P lipids 3-Phosphoglycerate amino acids PPP Ribose –5-P Nucleic acid/histidine Erythrose –4-P amino acids (aromatic) TCA cycle: acetyl-CoA lipids/sterols a-cetoglutarate amino acids (C5) succinate heme oxaloacetate amino acids (C4) Cells express three forms of ‘energy’ chemical energy ATP ATP4- + H20 ADP3- + Pi- G0’= -32,2 kJ/mole Coupling reaction - as phosphoryl donor ATP + H2 0 ADP + Pi G’° = -32,2 KJ/mol Glucose + Pi glucose 6P + H20 G’° = + 12,2 KJ/mol Glucose + ATP glucose 6P + ADP G’° = - 20 kJ/mol http://equilibrator.weizmann.ac.il/ Redox energy NAD(P)+ NAD(P)H NAD+ + 2e + 2 H+ NADH + H+ E0’= 0.32 V G°’ = -nF E°’ avec n= nombre de e et F = 96500 J xV-1x mole-1 Coupling reaction d’oxido-reduction Oxaloacetate + NADH +H+ Malate + NAD+ In vivo condition G°’ = -2 x F x [E°’ (oaa/malate) – E°’(NAD+/NADH)] Malate = 10-2M G°’ = -2 x F x (-0,17 + 0.32) = -28.9 kJ/mole NAD+ = 0.002M NADH= 0.0001M OAA= 0.0001M Note: G = G0’ + RT ln (products)/ [substrates] Catabolism versus anabolism glucose Transport Cell materials glucose 6P monomers polymers sugars-P carbohydrates Glycolysis a.a. proteins fatty acids lipids [4H] nucleotides DNA, RNA 2ATP Pyruvate oxidation 2 pyruvate [4H] CO2 2 acetyl-CoA [4H] [24H] Krebs cycle Respiratory chain ATP [4H] ATP CO2 [4H] CO2 O2 2 H2 O 1- Glycolysis: questions 1- What do we do with NADH? 2- What do we do with ATP 3- What are the end products of glycolysis? 4- How glycolysis is regulated? 5- What is controlling the glycolytic flux? 1- Glycolysis: questions 1- What do we do with NADH? Fermentation Respiration ½ 02 + NADH H20 + NAD+ 1- Glycolysis: questions 2- What do we do with ATP It is used for ‘anabolic reaction’ such as ATP + S P + ADP (AMP +Pi) Coupling catabolic activity (generate ATP) with anabolic activity for biomass production! can be storage of energy into a polymer like glycogen when growth is limited. can be lipids storage when excess of reducing power and energy under growth limitation. Glucose +ATP -> glc6p + ADP -> Gl1P + UTP (ATP) -> UDPGlucose +Ppi -> (glucose)n , liaison a1,4 et a1,6 glucosyl UDP +ATP -> UTP + ADP 1- Glycolysis: questions 4- How is glycolysis regulated? by effectors on key enzymes by the end product, ATP 5- What is controlling glycolysis? see chapter on Metabolic Control Analysis Note: Regulation # Control ! -> regulation is about ‘homeostasis’ -> control is about flux and production 2- Pentose phosphate pathway 2- Pentose-phosphate pathway 2- Pentose-phosphate pathway 1- What are the products of the PPP NADPH and Ribose-5P (D-erythrose 4-P) 2- How is PPP controlled? by ratio NADPH/NADP+ by ATPMg (inhibition of glucose -6-phosphate dehydrogenase)! 3- Krebs cycle or Tricarboxylic cycle (TCA) Attention: In E.coli, the first two reactions on the TCA generate NADPH instead of NADH And a transdehydrogenase allows conversion of NADPH into NADH. 3- TCA Cycle : amphibolic pathway Amino acids (C4) Fatty acids Heme synthesis Amino acids (C5) 3- TCA: regulation a- Amphibolic nature This needs anaplerotic reaction to refill the cycle replenishes the supply of intermediate metabolites ex: reaction catalysed by pyruvate carboxylase (biotine dependent) Pyruvate + HC03- +ATP oxaloacetate + ADP + Pi PEP + CO2 -> oxaloacetate + Pi (PEP carboxylase) b- controlled by ADP/ATP and NADH/NAD ratio The targets are Citrate synthase: inhibited by ATP and citrate isocitrate dehydrogenase: stimulated by ADP 4- Glyoxylate cycle: shunt the loss of Carbon in TCA takes place in plants, fungi etc. converts acetyl CoA into carbohydrates gl6P PEP gng CO2 pyruvate NADP+ NADPH The net reaction is 2 acetyl-CoA + NAD+ → succinate + 2 CoA + NADH + H+ 5- Fatty acids biosynthesis 5- Fatty acids biosynthesis Fatty Acid Synthesis Pathway FA Synthase Complex 6- Fatty acids degradation (mitochondrial vs peroxisomial) Note: in bacteria, only the Fatty- acid degradaytion-like “mito” occurs 7- Fatty acids degradation (peroxisomial) Peroxisome in liver cells Initiation of fatty acid oxidation PART 2: Energetic and transport I. Introduction system at equilibrium A B Keq = [B]/[A] (closed system) rate A B=B A Equilibrium versus steady state A B gi =[B]/[A] # Keq (open system) G6P pgi F6P F1,6P2 rate A B#B A ATP ADP rate versus flux A v1 B Flux = v2-v1 (for most of the reaction) v2 Coupling Common sense in biology as it serves to connect two systems/ two processes Cells express three forms of ‘energy’ chemical energy ATP ATP4- + H20 ADP3- + Pi- G0’= -32,2 kJ/mole Coupling reaction - as phosphoryl donor ATP + H2 0 ADP + Pi G’° = -32,2 KJ/mol Glucose + Pi glucose 6P + H20 G’° = + 12,2 KJ/mol Glucose + ATP glucose 6P + ADP G’° = - 20 kJ/mol http://equilibrator.weizmann.ac.il/ Redox energy NAD(P)+ NAD(P)H NAD+ + 2e + 2 H+ NADH + H+ E0’= 0.32 V G°’ = -nF E°’ avec n= nombre de e et F = 96500 J xV-1x mole-1 Coupling reaction d’oxido-reduction Oxaloacetate + NADH +H+ Malate + NAD+ G°’ = -2 x F x [E°’ (oaa/malate) – E°’(NAD+/NADH)] Malate = 10-2M G°’ = -2 x F x (-0,17 + 0.32) = -28.9 kJ/mole NAD = 0.002M NAD+= 0.0001M Note: G = G0’ + RT ln (products)/ [substrates] OAA= 0.0001M Chemiosmotic energy out in H+ Electrochemical potential of H+ µH+ = µ°+ RT ln[H+] + z FY µi -µo = RT.ln[H+]i/[H+]o + zF (Yi-Yo) Dividing by F and changing ln[H+] by -2,3 log[H+] (pH) µH+/F = P = -2,3 RT pHi-pH0 + Y gradient electrical Energetic conversion in the cell Two means to get ‘Cell Energy’ and to produce the cellular money’ 1- ATP synthesis at the Substrate Level Phosphorylation ex: PEP + ADP Pyruvate + ATP widely used during microbial fermentation low yield ex: yeast alcoholic fermentation: 2ATP/glucose homolactic fermentation 2ATP/glucose in out ATP 2- Chemiosmotic coupling mH+ P = Y – z pH ADP with z (charge) = -2,3RT/F = 0.059 volt H+ GATP = -m FP; ATPsynthase with m = number of H+ and F= 96500 Joule/volt x mole The respiratory chain The ‘classical’ respiratory chain Composed of 4 ‘redox/ pump’ complexes complexe I, II, III, IV needs to be embedded in a ‘non-permeable’ membrane to proton in between two compartments For ‘prokaryote’, it is localised at the cellular (plasma) membrane For ‘eukaryotes’, it is localised at the inner membrane of the mitochondria Coupling H+ transport and ATP synthesis a) Structure of the E.coli F0F1 ATPasynthase Coupling H+ transport and ATP synthesis b) Stoechiometry - NADH/ electron and protons- to ATP synthesis Equation: P = (Yi - Y0) - 2,3RT/F (pHi-pH0) E’° = 1,12V G°’ = -n FP = -218 KJ/mol, with n = 2 (electrons) G’° (ATP) = 32,2 KJ/mole How many ATP? (maximum = 3 ATP/ NADH: why?) Coupling H+ transport and ATP synthesis c) uncoupling Respiration and ATP synthesis Coupling H+ transport and ATP synthesis d) respiratory Control (RC) When an uncoupling is added is the o2 consumption taking place because the uncoupler causes the H+ to move out of the cell and thus accept the O2? RC = b/ a P/O = moles ADP /1/2 moles oxygen consumed Molecules perturbator It can alter the coupling - Weak acid such as acetate - Fatty acids Summary: Energetic conversion in the cell active transport synthesis mobility S NADH NADPH S active transport + + + + + + fermentation respiration O2, ATPsyn NO3-, P ase ATP SO4-- - - - - - - DCCD oligomycin Fermentation uncoupling hydrolases products C02 Type of transport a) passive/ facilitated transport b) Active/ carrier-mediated transport (secondary transport) c) endocytosis/exocytosis Energetic of transport Passive transport: Facilitated/active transport Fick Law « Michaelis-Menten Kinetic » Energetic of transport out In yo yi S H+ Transport S from out to in= change of electrochemical potential of Si and So with Z = charge of the solute S F = 96 500 Coulombs (electric charge associated to one mole of electron) µs = RTln[S0]/[Si] µH+ = RT Ln [H+]°/[H+]i + F [Y°-Yi] À l’equilibre: µs = µH+ RTln[S0]/[Si] = RT Ln [H+]°/[H+]i + F [Y°-Yi] Particularity of transporters From http://www.tcdb.org/tcdb/ 1. Channels/Pores 2. Electrochemical Potential-driven transporters 3. Primary Active Transporters 4. Group Translocators 5. Transport Electron Carriers 8. Accessory Factors Involved in Transport 9. Incompletely Characterized Transport Systems Particularity of transporters 6.1. General « structure » of a permease Particularity of transporters electrochemical driven proton pump (primary active transporter) P-ATPase Na+/K+-ATPase Ca++-ATPase G (ATP) ->P transport actif de molecule donc gradient Particularity of transporters Group translocators The phosphotransferase system (PTS) in bacteria Glucose + PEP glucose 6 –P + Pyruvate Multi drug resistance (MDR) (From Piddock, Nat Rev Microbiol, 2006) Part 3: Regulation General strategy of the metabolic control The cellular control that eventually adapts enzymes to the metabolic purposes takes place at two levels: (i) Modulation of the catalytic enzyme activity ie. change in substrate, effector, covalent modification. This is currently termed ‘metabolic regulation’ (i) Modulation of the amount of enzyme. This is currently termed ‘hierarchical control’ structure of metabolic pathways Linear pathway Ex: amino acid synthesis = accumulation of end product Cycling pathway Ex: Krebs cycle = amphilbolic nature , intermediates do not need to be abundant, just ‘catalytic’ Spiral pathway Ex: synthesis/ degradation fatty acids = like a linear but in a constrained manner avoid ‘dilution’ of intermediates in the cell medium lead to accumulation of end product or its degradation Control by modulation of the enzyme activity the committed step in a metabolic pathway Control by modulation of the enzyme activity a) By non-covalent, allosteric effectors Binding of allosteric effectors at allosteric sites affect catalytic efficiency of the enzyme Control by modulation of the enzyme activity a) By non-covalent, allosteric effectors Allosteric Activators – Decrease Km (increases the enzyme binding affinity) – Increases Vmax (increases the enzyme catalytic efficiency) Control by modulation of the enzyme activity By non-covalent, allosteric effectors Molecules that can act as allosteric effectors in the cell: End products of pathways – Feedback inhibition ! Ex: ATP inhibits PFK in glycolysis Substrates of pathways – Feed-forward activators ! Ex: Fru1,6-P2 activates Pyruvate kinase Indicators of Energy Status – ATP/ADP/AMP – NAD/NADH – Citrate & acetyl CoA Control by modulation of the enzyme activity b) By covalent modification (reversible mechanism) AX A Converter enzyme I Can affect Vm, Enz-R Enz-R-X Km, Ka, Converter enzyme II Ki X Exemple: -Phosphorylation/dephosphorylation -Ser/thr kinase; His-kinase, Tyr-kinase/Protein phosphatases Type1, 2a,2b, 2c - Methylation/demethylation -Che system in bacteria -Acetylation/deacetylation -Histone in eucaryotes - ADP ribosylation -EF-2 factor in response to botulism toxin - Uridylylation/deuridylylation -Glutamine synthase in E.coli. Control by modulation of the enzyme activity Ex 1: Protein phosphorylation/ dephosphorylation Serine/threonine protein kinases: phosphorylation of a serine/threonine residue Tyrosine protein kinases: phosphorylation of a tyrosine residue Histidine protein kinases: phosphorylation of a histidine residue (mainly present in bacteria) High number of ‘protein kinases’ in eukaryotic cells (> 100 in yeast ) Modulation of the amount of enzyme Specific proteolysis can activate certain enzymes and proteins (zymogens) – Digestive enzymes – Blood clotting proteins – Peptide hormones (insulin) Protein degradation is as essential to the cell as protein synthesis. For example, to supply amino acids for fresh protein synthesis to remove excess enzymes to remove transcription factors that are no longer needed. There are two major intracellular devices in which damaged or unneeded proteins are broken down: lysosomes and proteasomes Modulation of the amount of enzyme At the level of transcription in bacterial cells ‘operon’ repressorI promoter operator gene1 gene2 gene3 RNApol NO TRANSCRIPTION in yeast cells PolII ORF1 ‘regulon’ -750/-350 -750/-350 -200/-70 -150/-20 +1 TATAA Ti ATG Upstream Upstream Activating Repressing Transcriptional Sequence Sequence initiation Modulation of the amount of enzyme Induction activation (derepression) Modulation of the amount of enzyme Repression Intrinsic control of metabolic pathways General rule: In a given, linear metabolic sequence, the enzyme that catalyses the first committed step is usually retro-inhibited by the end product of this pathway. This is often seen for amino acids biosynthesis pathways, as well as in purines and pyrimidines pathways Intrinsic control of metabolic pathways Integrated mechanism of control Signal transduction in an eukaryotic cell Stimulus (Ligands) Sensor/receptor GDP GTP (signal/snd messenger) Kinase cascade Transducer(s) Targets/cellular responses Metabolic/genetic responses Integrated mechanism of control 3 – The Intracellular Messengers: Cyclic AMP (cAMP) Diacylglycerol (DAG) & Inositol Triphosphate (IP3) Cyclic GMP (cGMP) Fructose 2, 6- bisphosphate i) To be formed and active in a cell at very low concentration (10-6 to 10-7 M) ii) Be synthetized from ubiquitous molecules such ATP for cAMP and Fru6P + ATP for F2,6P2 but their synthesis/ degradation systems must be highly controlled iii) To be not an intermediary of any metabolic pathway IV.6. Integrated mechanism of control Signal transduction dependent on the cAMP also called: ‘Protein Kinase A signalling pathway’ (typical case of a GPCR) Integrated mechanism of control Ex 1: Control of Glycolysis/gluconeogenesis in liver by the PKA signalling pathway Integrated mechanism of control Ex 2: Control of Glycogen degradation in mammals by the PKA pathway Integrated mechanism of control Ex: Insulin receptor in liver cells Integrated mechanism of control Ex 3: insuline-Tyrosine Kinase signalling pathway regulating glycogen deposition Integrated mechanism of control Conclusions: Many signalling pathways in eucaryotic cells examples shown here are main signalling cascades in yeast Integrated mechanism of control Global control in a bacterial cell Stimulus/ligands T, pH, C, N-sources etc. Two-components system Sensor/receptor ADP ATP His-OP (signal) N- sensor -C ADP Transducer(s) ATP Regulator -C N- Regulator Asp-OP Cellular responses Targets Integrated mechanism of control two components system in bacteria Integrated mechanism of control Ex: Chemosensory transduction in Bacteria Metabolic and hierarchical control Glucoseout ATP glucosein (glycolysis) = rate of ethanol.hr-1 g-1 cells glucose-6-P What limits this rate? ATP fructose-6-P PFK PFK = no rate limiting in glycolytic flux, Why? fructose-1,6-P But ‘ if ATP utilisation rate is accelerated’ glycolysis 2 NADH This would increase the flux, Why? 2 ATP 2 ATP 2 NAD+ 2Ethanol Metabolic and hierarchical control Flux Jp (min-1.g-1 cells) es ea eb ec ed e S a b c d e p p In a metabolic pathway, one has to use commonly the term ‘rate limiting step’ But! The rate of P or flux of P is not necessarily dependent on this rate limiting step at this reaction step, This leads to the notion of ‘METABOLIC CONTROL’ definition: ‘An enzyme may control the flux of a pathway, which means that any little change in its concentration [E] (activity, amount) would lead to a change in the flux [F] of the same extent as the change in the concentration of [E] Metabolic and hierarchical control Metabolic Control Analysis (MCA) Concept: The claim that’ a metabolic pathway ‘is rate limited by a single enzyme is not exact In a given metabolic pathway, each enzyme participates to a certain degree to the flux within the pathway. This notion has been quantitatively expressed as ‘control coefficient’ for any enzyme of the pathway. 1- Control coefficient: E y X0 S1 ….S S6 …. P Jp Slope = C Expressing as a fractional changes of E and J dJ/J Jp = dJ/dE. E/J (lnJ) C E = d lnJ/dlnE dE/E (lnE) IV.7. Metabolic and hierarchical control Metabolic Control Analysis (MCA) 4- Measuring Control coefficient by direct method Several methods can be used to determine control coefficients by perturbation of the rate of reaction: Alteration of enzyme concentration by genetic means. Titration with inhibitors. Titration with purified enzyme. The most important point to have in mind is that each perturbation should affect one step only. If one wants a complete picture of the control of the variable in question, then the same procedure will have to be repeated for each step of the system. However easy this might appear, there are several problems associated with this approach Metabolic and hierarchical control Hierarchical and Metabolic Control Analysis genee mRNAe Hierarchical control Protein [P] Metabolic control S A B C D P E F G JP = f([S],[A], [B],…[Xi]) flux Hierarchical and Metabolic Control Analysis Mathematical formulation of the hierarchical and metabolic control For any enzyme v = f (e). g (S, P, M) ln v = lnVmax + ln g(S,P, M) activity [enzyme] [S, effectors] e en Steady state 1: J1 S1 A1 ….F1 H1 …. P1 e en Steady state 1: J2 S2 A2 ….F2 H2 …. P2 Since J ~ lnv , and dividing by J in both sides ln Vmax + ln g (S,P,M) 1= J J rh rm (From Ter Kuile & Westerhoff, Febs Lett. 500, 169, 2001) Metabolic and hierarchical control genee rtranscript mRNAe rtransla rh Protein [P] rpostransla rm S A B C D P E F G JP = f([S],[A], [B],…[Pi]) flux Metabolic and hierarchical control Hierarchical rh: E y Metabolic rm: X0 S1 ….S S6 …. P Jy rh dlnEi/dlnJ rm = 1- rh rh = 0; rm =1 metabolic lnE rh=1; rm=0 transcriptional rh=0.5; rm=0.5 shared rh=-1; rm=2 compensatory lnJ (Ter Kuile & Westerhoff, Febs Lett. 500, 169, 2001) A case study: flux control in the Chitin metabolic pathway in S. cerevisiae 80 60 % of the cell wall Glucose (Glucan) 40 Mannose (Mannoprotein) Glucosamine (Chitin) 20 0 wt mnn9 kre6 knr4 fks1 Chitin metabolic pathway in S. cerevisiae Glucose Glucose-6-P 2-10% Carbohydrate Reserve (glycogen / trehalose) Gfa1p Glycolysis 80% Fructose-6-P + Glutamine Glucosamine-6-P + Glutamate Acetyl-CoA CoASH Gna1p N-acetyl-glucosamine-6-P Agm1p N-acetyl-glucosamine-1-P Chs4,5,6,7 UTP PPi Uap1 Chs1,2,3 UDP-N-acetylglucosamine (Chitin) + UDP n OH OH OH O O O HO O O OH HN-COCH 3 HN-COCH 3 HN-COCH 3 cell wall n (chitin) n-1 Biosynthesis of chitin in yeast Flux of chitin production (µg/g dry mass x hr) = content of chitin (µg/drymass) x growth rate (hr-1) 2.0 ln rate of chitin production (µg/mgdw/hr) 1.5 1.0 0.5 Slope 0.90 C Jchitin Gfa1 0 0 0.5 1 1.5 2 2.5 3 3.5 4 ln Gfa1p (nmol/min/mg protein) Correlation: Transcript of GFA1 versus activity of Gfa1p 4 of Gfa1p activity Relative increase gas1 3 mnn9 2 kre6 knr4 1 wt 0 0 1 2 3 4 Relative increase of GFA1 mRNA Biosynthesis pathway of chitine F6P Gfa1p Gfa1p Gna1p Agm1p Uap1p Chsp Chitin + Gln Correlation : Transcript levels and chitin deposition GFA1 CHS3 CHS1 4 Relative increase of GFA1mRNA 3 GFA1 2 CHS3 1 0 0 5 10 15 20 25 CHS1 µg chitin / mg dry mass () wt () kre6 () knr4 () mnn9 () gas1 Biosynthesis pathway of chitine F6P GFA1 GFA1 GNA1 AGM1 UAP1 CHSs CHS1,3 Chitin + Glu Biosynthesis of chitin in yeast Flux is controlled mainly at the transcription level 4.0 (nmol/min/mg protein) 3.0 ln Gfa1p 2.0 1.0 0 0.5 1 1.5 2 ln flux chitin (µg/mg dry mass.hr) r h = 0.88; r m = 0.12 Metabolic and hierarchical control In summary REGULATION is about “HOMEOSTASIS” CONTROL is about “PRODUCTIVITY” Ex: In yeast, Glycolysis is regulated mainly at the PFK reaction step but…. the flux of glycolysis is controlled by the glucose uptake / or ATP utilisation or.. else ELASTICITY is about degree of enzymatic change to change of its effector Ex: an enzyme with an elasticity of 10 , this means that 10% change due to the effector if this enzyme has 100 % of control in the pathway, then 10 % of change would result in 10 % change in flux