BIOCHEM LEC PDF
Document Details
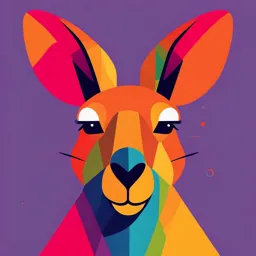
Uploaded by TollFreeScandium
Tags
Summary
This document appears to be lecture notes on biochemistry focusing on enzymes, their characteristics, and mechanisms of action. It discusses topics like enzyme features, classification, and mechanisms of catalysis.
Full Transcript
MODULE 5 - Enzymes: The Catalysts of Life The Features of an Enzyme and Enzyme-catalyzed Reaction Enzymes are globular proteins that serve as catalysts of biochemical reactions. The reactant or substrate binds to the hydrophobic cleft within the enzyme called the active site which serves as its ca...
MODULE 5 - Enzymes: The Catalysts of Life The Features of an Enzyme and Enzyme-catalyzed Reaction Enzymes are globular proteins that serve as catalysts of biochemical reactions. The reactant or substrate binds to the hydrophobic cleft within the enzyme called the active site which serves as its catalytic site. This process results to the formation of an enzyme-substrate complex (ES) that eventually dissociates to form the product and with subsequent recovery of the enzyme. Regardless of the mechanisms by which an enzyme facilitate the formation of the ES complex, the process results to a lower energy of activation (Ea), hence facilitating a faster reaction, while the enthalpy (ΔH) of the reaction remains constant as illustrated in figure below. Knowing how enzyme works will help you in understanding enzyme kinetics which is the study of the velocity of enzymatic reaction. There are two models by which we can represent the structural relationship between the enzyme and its substrate(s) namely the Lock and Key Model and Induced-fit Model. In the Lock and Key Model by Emil Fischer, the enzyme and its substrate(s) have complementary shape, which means that the substrate(s) fit through the enzyme active site like a key fits into a lock. Meanwhile, in the Induced-Fit Model by Daniel Koshland, the loose binding of the substrate(s) into the active site of the enzyme induces a conformational change in the enzyme's active site that results to a tighter fitting of the substrate(s). The two models are compared in the figure below. Classification of Enzymes Enzymes are classified according to the type of reaction they catalyze. The International Union of Biochemistry (IUB) proposed a systematic scheme of classifying and naming enzymes. The six general classes of enzymes are listed in Table 5.1 below: A numbering system is designed in naming an enzyme like 4.3.2.1. The first number stands for the major class of the enzyme which is lyase. The second number is the subclass which specifies the type of bond that was cleaved. The third number is the sub-subclass of the enzyme while the last number is the identification number. This numbering system, although systematic is cumbersome for general use hence the traditional method of naming enzyme still persists. Enzyme Prosthetic Groups Many enzymes require a nonprotein part in order to function properly called prosthetic groups. They are specifically called co-enzymes if they are derived from vitamins or as co-factors if they are derived from minerals or metals. A. Co-enzymes - vitamins that serve as co-enzymes are the vitamin B complex and vitamin C, which are all water soluble vitamins. They are not stored in our body and so they need to be taken daily in adequate amounts. The recommended dietary allowance (RDA) is the minimum amount of nutrients that should be taken daily from dietary sources or supplementation. Prolonged deficiency in these vitamins leads to certain disorders. The coenzyme form, reactions catalyzed, RDA, dietary sources and disorders associated with the deficiency of these vitamins are listed below. B. Cofactors - metals are electrophilic groups that aid in the functioning of enzymes. They are coordinated by certain amino acid residues in the enzyme. They induce by coordination a specific lock geometry for the attachment of substrates and maintenance of the native conformation of the enzyme. They can also activate certain bonds in the enzyme and/or substrate to facilitate catalysis. Some examples enzymes that requires metal cofactors are given below. Mechanisms of Enzyme Catalysis There are six mechanisms by which an enzyme facilitates catalysis namely: 1. Proximity and Orientation Effect The Collision Theory of Reaction states that every chemical reaction requires collision between the reactant particles and a successful reaction occurs when the colliding particles are in proper orientation and collides with sufficient energy. When the substrates bind at the enzyme's active site, it minimizes the energy of activation and bring the substrate particles at a proper orientation during their collision. This is known as proximity and orientation effect. The figure below shows the conditions for successful reaction as per the Collision Theory of Reaction. 2. Transition State Stabilization) It was classically theorized that conformational distortion or stain effect occurs as the enzyme somehow causes the substrate particles to become distorted toward the transition state. However, evidence show that it is unlikely and the enzyme might even be the one that is more strained than the substrate. The more acceptable concept is the Transition State Stabilization wherein the transition state makes better contacts with the active site of the enzyme than the substrate, hence, sufficient binding energy is not reached until the substrate enters the transition state. An example of transition state stabilization by the enzyme's active site amino acid residues is shown in the figure below. 3. Electrostatic Catalysis The enzyme's active site is actually a hydrophobic pocket or cleft which means that it has much fewer water molecules as compared to the exterior of the enzyme. The exclusion of water results to a desolvation effect which lowers the local dielectric constant surrounding the substrate particles. This enhances the electrostatic interactions of the substrate particles and the enzyme's active site. This also prevents the undue reaction of water molecules to the substrate to form unwanted side products. The charged functional groups in the enzyme's active site also stabilizes the reaction intermediates. Both of this phenomena is included under electrostatic catalysis. This is illustrated for the enzyme superoxide dismutase in the figure below. 4. Acid-Base Catalysis The side chain functional group of several amino acids can donate or accept proton, hence, facilitating acid-base catalysis. The protonation and deprotonation cascade or charge transfer relay results to more reactive intermediates than the original substrates, hence, the reaction occurs at a faster rate. This is illustrated in the enzyme acetylcholinesterase where histidine residue serve as base catalyst for the reaction. 5. Covalent Catalysis Transient or intermediate covalent bond can form between the substrate and electrophilic or nucleophilic group amino acid residue in the enzyme's active site. Examples of which are acyl-serine, acyl-cysteine, phospho-amine. The prosthetic group or non-protein component of an enzyme such as B-vitamins and metals can also form this intermediate covalent bond with the substrate. An example of covalent catalysis is in chymotrypsin where serine residue forms a transient acyl-serine covalent bond with the protein substrate to facilitate the catalysis. 6. Metal Ion Catalysis Some enzymes contain metal ions as prosthetic group as as metalloenzymes where the metal ion is tightly bound (such as c, Fe3+, Cu2+, and Zn2+); metal-activated enzymes where the metal ion is loosely bound (such as Na+, K+, Mg2+, and Ca2+); or organometallic enzymes where the metal is bound in an organic prosthetic group (such as such as Fe2+in heme). The metal ion can perform any of the following functions: bind the substrate in a specific orientation or lock geometry; mediate electron transfer; electrostaically stabilize or shield negative charges; or stabilize the transition state. AN example of which is the role of zinc cation in alcohol dehydrogenase enzyme. Enzyme Regulation Enzymes are regulated in the cells by compartmentation, covalent modification, allosteric regulation, proteolytic activation and transcriptional control. 1. Compartmentation – enzymes are usually localized within a region or organelle in the cells. For example, the acid hydrolases that degrade worn out organelles and biomolecules, are confined within the lysosomes. If these enzymes are released to the cytoplasm, they will degrade other cell organelles. In addition, the acidic pH in the lysosome optimizes the activity of these enzymes. Meanwhile, the enzymes involved in the energy metabolism of the cell, is localized in the mitochondria where they are further distributed in the inter-membrane space, inner membrane, or the matrix. 2. Covalent Modification – the reversible attachment of a chemical group to specific amino acid residues in the enzyme may result to its activation or deactivation. The attachment of phosphate group (phosphorylation) is the most common way of regulating the activity of an enzyme. This is accomplished either by cyclic adenosine monophosphate (cAMP), cyclic guanosine monophosphate (cGMP), or kinase enzymes. Phosphate is attached on serine, threonine, or tyrosine residues of the enzyme. Phosphate adds a large negative charge to the enzyme that changes its conformation. This leads to an increase or a decrease in the activity of the enzyme. 3. Allosteric Regulation – this is the noncovalent binding of small molecules called effectors to sites in the enzyme other than the active site. The interaction induces conformational changes that activate or deactivate the enzyme. The enzymes that are acted upon by such effectors are known as allosteric enzymes. They are made up of several subunits that contain various regulatory sites. Feedback inhibition is the deactivation of an allosteric enzyme by one of its end products. This is the usual way of regulating a multi-step reaction pathway. 4. Proteolytic Modification – this is the removal of some amino acid residues from an inactive enzyme to convert it to its active form. Some enzymes and proteins are synthesized in inactive forms called zymogens. This is especially true for digestive enzymes. For example, both trypsin and chymotrypsin are synthesized as inactive trypsinogen and chymotrypsinogen respectively. Specific amino acid residues are removed, and the fragments are joined by disulfide bridges to make the enzymes functional. 5. Transcriptional Control - this is the regulation of the genes that encode for a particular enzyme. Regulatory substances like hormones and growth factors, can directly or indirectly affect the expression of these genes. If the regulatory substance is polar, it binds to specific receptors in the cell membrane. This turns on a cascade pathway that activates a series of enzymes and proteins that ultimately affect gene expression. If the regulatory substance is non-polar, it crosses the cell-membrane and binds to specific cytoplasmic receptors. The complex formed passes through the nucleoplasm and binds directly to a specific segment in the DNA to affect gene expression of certain enzymes or proteins. MODULE 6 - Carbohydrates (The Sweet and Bitter Stuff) Monosaccharides Carbohydrates is a major class of biomolecules that is literally formed by hydration of carbon atoms, Cn(H2O)n. The building blocks of all carbohydrates are called monosaccharides or simple sugars. Monosaccharides are polyhydroxy aldoses or ketoses which can be represented by the planar Fischer projection structure as illustrated in Figure 1 below. Although monosaccharides are conveniently represented by Fischer projection structures, they exist biologically in cyclic forms. In the so-called Haworth structure, the intramolecular reaction between the carbonyl carbon and hydroxyl group of the reference carbon forms a cyclic hemiacetal or hemiketal. The resulting structure is either based on the five-membered or six-membered cyclic ether known as furan and pyran respectively. The transformation to this structure is quite tricky so it’s best to follow the steps listed in Sample Exercise 1 below. Disaccharides Disaccharides are carbohydrates with two sugar units that are linked by an O-glycosidic bond that is formed by condensation of two hydroxyl (-OH) groups into an ether bond. Disaccharides are classified into reducing and non-reducing type. Reducing disaccharide are those with free hydroxyl group (-OH) on the anomeric center while non-reducing disaccharides have no free hydroxyl group in their anomeric center. Figure 2 below illustrates the structure of lactose (a reducing disaccharide found in milk) sucrose (a non-reducing disaccharide in plants). Polysaccharides Polysaccharides are made up of numerous sugar units usually in the hundreds and even thousands. Starch and glycogen are both storage polysaccharides which serve as energy reserves of living organisms. Starch is the penultimate storage form of glucose in plants and is made up of two distinct macromolecules called α-amylose and amylopectin. A α-amylose macromolecule has linear chain of glucose subunits that are connected by α(1->4) O-glycosidic bond. Amylopectin has the same linear glucose chain base as in α-amylose but it has α(1->6) glucose branch for every 24-30 glucose residues. Glycogen is the storage form of glucose in animals and humans which has a similar structure to amylopectin except that the α(1->6) branching occurs more frequently at around 8-12 glucose residues. The figures belwo show the comparative structure of α-amylose and amylopectin in starch and glycogen. Reactions of Carbohydrates MODULE 7 - Lipids (The Good, Bad, and Ugly) Fatty Acids Lipids are water-insoluble biomolecules but are soluble in nonpolar solvents like chloroform, ether, and hexane. Unlike monosaccharides in carbohydrates, lipids have no single common building blocks hence they have more diverse in nature. There are eight major common groups of lipids namely fatty acids, acylglycerides, phospholipids, sterols, sphingolipids, isoprenoids, glycolipids, and polyketides. We will focus on the first four groups of the mentioned lipids in this module. Fatty acids are carboxylic acids (RCOOH) with long hydrocarbon chain. They can be classified into saturated fatty acids if there have pure single bonds on their hydrocarbon chain or unsaturated fatty acids if there is one or more C=C bond in their backbone. The Table below gives the most common saturated and unsaturated fatty acids and their notation. Interestingly, naturally-occurring fatty acids have even number of carbon atoms. The notation in the parenthesis gives the number of carbon atoms and C=C double bonds in the subscript and the position of such C=C double bond in the superscript. The melting point also increases with increasing molecular weight. For example, stearic acid has the notation C18:0 which means that it has 18 carbon atoms with no C=C double bond. Meanwhile, linoleic acid has the notation C18:2Δ9,12 which means that it also has 18 carbon atoms but with two C=C bonds located on carbons 9 and 12 respectively. Take note that for unsaturated fatty acids, the double bond is always cis- in configuration. In addition, the cis- double “bends” which renders a twisted instead of a linear shape for an unsaturated fatty acid. The figure illustrates the structure of stearic acid and linoleic acid. Since fatty acids are carboxylic acids, they possess a net negative charge from the dissociation of H+ of the carboxylic acid (-COOH to –COO-). In terms of melting point, saturated fatty acids would have higher melting point than equivalent unsaturated fatty acid. This is due to the greater number of intermolecular forces of attraction (particularly London dispersion forces) between the linear saturated fatty acid molecules. Consequently, the bent and more compact structure of unsaturated fatty acids leads to less of these interactions, hence, their lower melting point. For example, the melting point of stearic acid is around 690C while than of linoleic acid is at -50C. That is a stark difference even though they both have the same number of carbon atoms. Acylglycerides Acylglycerides (or simply glycerides) are esters of glycerol and fatty acids, hence they are considered as conjugated lipids. Unlike fatty acids which have a net negative charge, acylglycerides are neutral in nature. Monoacylglycerides, diacylglycerides, and triacylglycerides have one, two, and three fatty acids attached to –OH group of glycerol via ester bond as illustrated in the figure below. Diacylglycerides (DAG) act as “secondary messenger” molecules that transmit biochemical signals in the process called signal transduction. Meanwhile, triacylglycerides (TAG) serve as the storage form of fatty acids as oil or fat. Oil is the storage of fatty acids in plants and has an abundant amount of unsaturated fatty acids. Since unsaturated fatty acids have lower melting point than saturated ones, oil is liquid at room temperature. Meanwhile, fat is the storage form of fatty acids in animals and humans and is predominantly made up of saturated fatty acids. Since saturated fatty acids have higher melting point than unsaturated ones, fat is solid at room temperature. Phospholipids Phospholipids are phosphate esters of diacylglycerides. The phosphate group is polar and negatively charge in nature, which renders a polar characteristic to the otherwise non-polar hydrocarbon backbone. This is the reason why phospholipids are said to be amphipathic molecules – that is they contain both polar and non-polar components as illustrated below. The simplest phospholipids are called phosphatidic acids and they have the plain phosphate group at the head of the structure. More complex phospholipids are formed by conjugating ethanolamine, choline, and serine to the phosphate group as shown in the figure below. The mentioned small molecules forms phosphoester bond when their hydroxyl group (–OH) condenses with phosphate forming phosphatidylethanolamine (PE, also known as cephalin), phosphatidylcholine (PC, also known as lecithin), and phosphatidylserine (PS) This amphipathic nature of phospholipid makes it an important biomolecular component of the cell’s plasma membrane as phospholipid bilayer as shown in the Figure below. The polar “phosphate head” at the outer leaflets of the bilayer can interact with the polar aqueous environment of the cytoplasm and extracellular matrix. The nonpolar “fatty acyl tails” form the hydrophobic core of the bilayer which prevents the dissolution of the membrane by water, thus, delineating the cell from its environment. Sterols and Steroids Sterols are polycyclic conjugated lipids with perhydrocyclopentanophenantrene as base structure. There are three main kinds of sterols called phytosterol, ergosterol, and zoosterol which are found in plants, fungi, and animals respectively. Cholesterol is the most important zoosterol in animals and humans as illustrated below. It can be seen that cholesterol has four conjugated rings (rings A, B, C, and D) with 17 carbon atoms in the ring system. There are two methyl substituents that are attached to carbons 10 and 13 and a larger alkyl chain attached at C17, giving a total of 27 carbon atoms. Lastly, a hydroxyl group is attached at carbon 3 and there is a double bond between carbons 5 and 6. Steroid hormones are hormones that are derived from cholesterol which includes the adrenocorticoids (glucocortocoid and mineralocorticoid) and sex hormones (estrogens, progesterone, and testosterone). Adrenocorticoids are cholesterol-derived hormones that are synthesized in the cortex of the adrenal glands which are found atop each kidney. Glucocorticoids are adrenocorticoids that have important metabolic and immunological functions. Metabolically, they increase blood glucose level by stimulation of gluconeogenesis in the liver which is the synthesis of glucose molecules from non-carbohydrate substrates such as amino acids and glycerol. Immunologically, they stimulate anti-inflammatory response in the body by upregulating the synthesis of anti-inflammatory proteins while downregulating that of proinflammatory ones. Glucocorticoid drugs are used as powerful anti-inflammatory drugs for the treatment of allergies, asthma, autoimmune disorders, and cancer. Meanwhile, mineralocortocoid is an anti-diuretic hormone (ADH) which decreases urine volume and regulates water and electrolytes (minerals) balance in the body. In particular, it acts on the kidneys to stimulate the reabsorption of sodium ions and water in the collecting tubules while promoting the secretion of potassium ions. This process also increases blood pressure (hypertension). Estrogens are the female sex hormones that stimulate ovulation which is the maturation of the oocytes. It also stimulates endometrial growth, calcium retention in the bones, and the development of secondary sexual characteristics in females. These secondary sexual characteristics include the development of the breasts, widening of the hips, and growth of pubic hair. There are three primary major forms of estrogen namely estrone, estradiol, and estriol. Meanwhile, progesterone is the hormone that prepares the female’s body for pregnancy and its maintenance. Testosterone stimulates the development and maturation of the sperm cells in the process called spermatogenesis. It also stimulates the development of secondary sexual characteristics in males which include the growth of male bodily hair pattern (mustache, beard, pubic hair, and armpit hair); deepening of the voice and enlargement of voice box (Adam’s apple); and strengthening sexual drive or libido. Lipid Vitamins