BIOC 2300 Lecture 12 Fall2024 PDF
Document Details
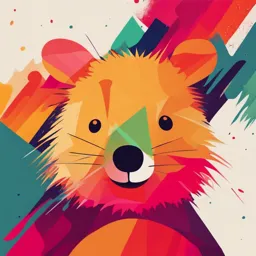
Uploaded by AwedVector
Dalhousie University
2024
Kathryn Vanya Ewart
Tags
Related
- BIOL340 Cell & Molecular Biology Lecture Notes PDF
- BCCB2004 Foundations of Cell Biology Lecture Notes PDF
- Membrane Biochemistry Lecture 10 - Transport Across Biological Membranes I
- Lecture Notes - The Cell PDF
- Transport Mechanism across Cell Membrane PDF
- Transport Across Biomembranes Lecture Notes PDF
Summary
These lecture notes cover membrane transport, including passive and active transport mechanisms, ligand-protein interactions, and quantitative treatments of protein binding. The document explores various aspects of cell biology and membrane processes. It details the fundamental forces behind intermolecular interactions and highlights the roles of proteins in facilitated transport.
Full Transcript
BIOC 2300 – Lecture 12 Membrane Transport Kathryn Vanya Ewart Room 9S, Tupper Medical Building [email protected] Content questions: [email protected] Session objectives Compare and contrast passive vs. active transport and the mechanisms of each. Many membrane...
BIOC 2300 – Lecture 12 Membrane Transport Kathryn Vanya Ewart Room 9S, Tupper Medical Building [email protected] Content questions: [email protected] Session objectives Compare and contrast passive vs. active transport and the mechanisms of each. Many membrane proteins bind to ligands. Review the fundamental forces that guide intermolecular interactions, the dissociation constant (Kd), and fractional saturation (Y). Explain the Hill coefficient, cooperative binding and relate the Kd to Gibb’s free energy. Explain how multipart ligands can have substantially greater affinity to their targets than their components. 2 Transport around the cell 3 Energetics of transport across a membrane Concentration gradient [X]A Lipid bilayer [X]B Passive transport occurs without energy input. Active transport requires energy input. 4 Membrane permeability Bilayers are not permeable to polar molecules/ions, which require protein-mediated (facilitated) transport. Figure 11-1 Molecular Biology of the Cell (© Garland Science 2008) 5 Which molecule could most easily move across a synthetic lipid bilayer? A. CO2 B. MgSO4 C. Water D. Glucose E. These can all traverse a lipid bilayer equally well 6 Which molecule could most easily move across a synthetic lipid bilayer? A. CO2 B. MgSO4 C. Water D. Glucose E. These can all traverse a lipid bilayer equally well CO2 is small and non-polar. It can therefore cross the lipid bilayer most efficiently. Water can cross to some extent, but less efficiently than CO 2, and so aquaporins (water channel proteins) contribute to water movement across membranes. Glucose is larger and polar and does not cross the membranes. It requires glucose transporter proteins. MgSO4 is ionic and would also not cross the lipid bilayer to any extent. 7 Methods of facilitated transport: Carrier molecules Hydrophobic exterior Valinomycin (an antibiotic) Hydrophilic interior Appling et al. (2018) Carrier molecules form a hydrophobic shield around polar molecules (often ions). 8 Methods of facilitated transport: Pores/channels Bacterial K+ channel Transport may be facilitated by pores/channels.... non-stoichiometric: fast (106/sec) No conformational change during transport always passive selective may be gated (e.g. regulated by protein binding to a by ligand or by voltage) OmpF pore 9 Methods of facilitated transport: Transporters Transport can also be facilitated by transporters: stoichiometric: slower (103/sec) Conformational changes passive or active (pumps) specific may be regulated Glucose transporter 10 Transport proteins have several modes of action e.g. Na-glucose e.g. glucose e.g. Na,K-ATPase transporters transporters Transporter proteins can move multiple ligands through conformational changes. 11 Uniport of glucose Glucose Transporters Often referred to as GLUT transporters. In one conformation, open to the extracellular side and pick up glucose. Then, change of conformation opens s 12 Effect of Na+/K+-ATPase on cellular ion concentrations Ion (mM) Outside Inside Na+ 150 5 - 15 K+ 4 140 Ca++ 1-2 10-4 Mg++ 1-2 0.5 The Na+ and K+ gradients are maintained by the Na +/K+-ATPase. This protein is is an ATP-dependent ion pump, which uses one-third of our total energy at rest. 13 Session objectives Compare and contrast passive vs. active transport and the mechanisms of each. Many membrane proteins bind to ligands. Review the fundamental forces that guide intermolecular interactions, the dissociation constant (Kd), and fractional saturation (Y). Explain the Hill coefficient, cooperative binding and relate the Kd to Gibb’s free energy. Explain how multipart ligands can have substantially greater affinity to their targets than their components. 14 Most transport across membranes is regulated Libretexts Biology: 3.23: Diffusion, Active Transport and Membrane Channels by J.W. Kimball (https://bio.libretexts.org/Bookshelves/Introductory_and_General_Biology/Biology_(Kimball)/03% 3A_The_Cellular_Basis_of_Life/3.23%3A_Diffusion_Active_Transport_and_Membrane_Channels) Ion channels, for example, can be gated (regulated) by voltage or small organic molecules that are not the ion that is transported. In response to ligand binding, the channel opens and allows the ions to cross into (or out of) a cell. 15 The nicotinic acetylcholine receptor: a ligand-gated channel Acetylcholine Cell membrane Receptor protein (a channel protein) Receptor protein in closed conformation (a channel protein) Libretexts Medicine: 3.5: Neurotransmitters- Acetylcholine in open conformation (https://med.libretexts.org/Sandboxes/admin/Introduction _to_Neuroscience_(Hedges)/03%3A_Neuronal_Communica tion/3.05%3A_Neurotransmitters-_Acetylcholine) Nicotinic acetylcholine receptors are ion channels that are regulated by the binding of the ligand acetylcholine. When acetylcholine is bound by the receptor, the receptor changes from a closed conformation to an open one. In the open form, ions can pass through the channel. This is one example of the regulation of molecule passage across a cell membrane. 16 Non-covalent forces drive intermolecular interactions! Heme binding to O2 Antibody binding epitope Transcription factor binding to DNA 17 Quantitative treatment of 1:1 binding For a typical intermolecular interaction between 1 protein and 1 ligand: Kon P + L ⇄ PL P = Protein (typically a protein at lower Koff concentration) At equilibrium… L = Ligand (small molecule ligand or another protein) P L K off = [PL]K on Koff = off rate, Kon = on rate Kd is the inverse of the Keq: Kd = 1/Keq Rearranges to: P [L] Koff A small Kd means the complex = = 𝐾𝑑 PL Kon is high affinity! Can determine by measuring rates or [L] concentrations! Fraction bound = Y = K +[L] d The dissociation constant (Kd) is a measurement of the affinity of two molecules for each other. 18 Classic binding curve [𝐿] Y= 𝐾𝑑+[𝐿] When 50% of P is bound: 𝑃 = 𝑃𝐿 Y 𝑃 [𝐿] 𝑃𝐿 [𝐿] 𝐾𝑑 = = = [𝐿] 𝑃𝐿 𝑃𝐿 𝐿 = 𝐾𝑑 [L] van Holde (Fig 14.6) The Kd is equal to the concentration of L at which 50% of P is bound. 19 Session objectives Compare and contrast passive vs. active transport and the mechanisms of each. Many membrane proteins bind to ligands. Review the fundamental forces that guide intermolecular interactions, the dissociation constant (Kd), and fractional saturation (Y). Explain the Hill coefficient, cooperative binding and relate the Kd to Gibb’s free energy. Explain how multipart ligands can have substantially greater affinity to their targets than their components. 20 Quantitative treatment of protein binding to two or more ligands Some proteins have multiple binding sites for the same ligand. Many of these are multi-subunit proteins (with quaternary structure) have multiple sites for a single ligand. So, they can bind two (or sometimes more) of a ligand at once. In these cases, the binding can become more interesting because there are three possibilities: Binding at each site is independent (no effect of any site on the others) Binding at each site favours binding at other sites. Binding at each site diminishes binding at other sites. 21 The Hill equation models cooperative binding Positive cooperativity, n>1 No cooperativity, n=1 Negative cooperativity, n