BIO515 Exam 2 Pt. 1 PDF
Document Details
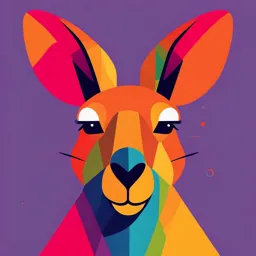
Uploaded by RetractableSitar
Tags
Summary
This document details the protein function, focusing on reversible ligand binding. It explains factors like specificity, cooperativity, and kinetics of ligand interaction. The document also introduces myoglobin, a protein with high affinity for oxygen, along with the concept of cooperativity in hemoglobin.
Full Transcript
BIO 515 EXAM 2 Protein function Reversible binding of ligands is essential. - Specificity of ligands and binding sites Ligand binding is often coupled to conformational changes, sometimes quite dramatic (induced fit) Multi-subunit proteins (w/ quaternary structure), conformational changes in one sub...
BIO 515 EXAM 2 Protein function Reversible binding of ligands is essential. - Specificity of ligands and binding sites Ligand binding is often coupled to conformational changes, sometimes quite dramatic (induced fit) Multi-subunit proteins (w/ quaternary structure), conformational changes in one subunit can affect the others (cooperativity) Interactions can be regulated. Illustrated by hemoglobin, antibodies, and muscle contraction A reversible transient process of chemical equilibrium: A+B à AB A molecule that binds to a protein is called a ligand (typically a small molecule) A region in the protein where the ligand binds is called the binding site. Ligand binds via same noncovalent forces that dictate protein structure. Why is this relevant? à allows the interactions to be transient, temporary, and reversible. Kinetics: - Association rate constant (ka) Dissociation rate constant (kd) At equilibrium: ka[P]*[L]=kd[PL] Equillium composition is characterized by the equilibrium constant Ka ["#] %& Ka=["][#] = %' Binding: - To figure out Ka we can often measure the fraction of occupied binding sites (q) In terms of the more commonly used equilibrium dissociation constant Solving for Kd, we get the definition of equilibrium dissociation constant Kd: - Kd=1/2 q “Kd is the concentration of ligand L upon half of the protein sites are occupied” Small Kd means HIGH affinity (takes less ligand to bind) Myoglobin - Structure: o o o o o a single polypeptide chain of 154 amino acids the chain consists of eight alpha helices (A through H) connected by bends. One heme group The heme group includes a porphyrin ring iron ion The heme bound FE cation can exist in the 2+ (reduced) or 3+ (oxidized) state. Specificity - Proteins typically have high specificity: only certain ligands bind High specificity can be explained by the complementary of the binding site and the ligand. Complementary in o Size o Shape o Charge o Or hydrophobic/hydrophilic character Specificity: Induced fit - - Conformational changes may occur upon ligand binding. This is called induced fit o Induced fit slows for tighter binding of ligands. o Induced fit allows for higher affinity for different ligands. Ligands and proteins can change their conformations. Globin’s are oxygen-binding proteins. - Protein side chains lack affinity for O2. Some transition metals (i.e. Fe+) bind to O2 well but would generate free radicals if free in solution. Organometallic compounds such as heme are more suitable, Fe2+ in free heme is oxidized to Fe3+. Evolutionary Solution: Capture the oxygen molecule with heme that is protein bound (evidenced in Myoglobin and hemoglobin) Porphyrin and Heme Heme group is a strong chromophore that absorbs both in ultraviolet and visible range – and changes color when bound to O2. This binding can be seeing through UV-Vis spectrophotometry. Flat ring organic molecules with high affinity for Fe, the Fe has 6 coordination bonds: - 4 with porphyrin ring - 2 are free (available) Myoglobin structure: - The His is called the “proximal” His, its close enough to the Fe that I coordinates with it directly. Binding to Oxygen Binding of Carbon Monoxide - CO has a similar size and shape to O2 it can fit in the same binding site Co binds free heme over 20,000 times better then O2 - But in protein pocket the heme has greatly decreased affinity for CO, but it still binds about 250 times better than oxygen. Protein Structure affects binding. 1. Complementary of the binding site and the ligand. O2 fits perfectly in the binding site whereas CO doesn’t “fit” very well. 2. Accessibility: The binding site is buried Depp inside the protein. The ligand can only reach it if protein “breathes” a little Co binds better to the free heme because of its shape, because its triple bonds form straight angles while O2 is on an angle. - However in the middle of protein CO only binds about 200x as well as O2 because of the specific shape of the binding site helps O2 bind but not CO. The distal His makes a H-bond with O2 but not with CO. Is myoglobin a good way to deliver oxygen to the tissues? - pO2 in lungs is about 13 kPa: it bind oxygen well pO2 in tissues is about 4 kPa Would lowering the affinity (P50) of myoglobin to oxygen help? - No Myoglobin cant release in tissues (high affinity curve) But just lowering affinity wont help bc now we don’t bind enough oxygen in the lungs What is the solution? - Hemoglobin! Hemoglobin - Hemoglobin binds oxygen cooperatively Tetramer of two subunits Each subunit is similar to myoglobin Cooperativity in Hb’s affinity for oxygen? - Requires quaternary structure with multiple subunits Requires a protein with multiple binding sites (like Hb) Subunits must be able to interact with each other. This is positive cooperativity first binding event increases affinity at remaining sites o Recognizable by sigmoidal binding curves Ligand Cooperativity Hill plot of cooperativity Quantitative description of cooperativity: - Cooperative proteins have multiple ligand-binding sites (n) So Ka becomes ["#(] o Ka=["][#]! [#! ] o And q becomes q =[#]! )*' o Taking the log of both sides gives the Hill Equation R and T states of hemoglobin - - - T=Tense State o More interactions, more stable o Lower affinity for O2 R=Relaxed state o Fewer Interactions, more flexible o Higher affinity for O2 O2 binding triggers a TàR conformational change. Conformational change from the T state to the R sate involves breaking ion pairs between the α1-B2 interface. The T to R transition triggered by Oxygen binding. Binding of O2 causes ab pairs to slide past each other and rotate, so C-terminal His on b1 loses salt bridge to Lys on a2 and turns toward center, narrowing the pocket. Conformation change is triggered by oxygen binding. - Max Perutz determined the structures if Hb in presence and absence of Oxygen and saw a significant conformational change. He hypothesized that it was caused by a shift in the irons position in the Heme upon oxygen binding which causes the His to shift, which cases the helix the His is on to move, which ultimately causes some salt-bridges between subunits to break, and the structures begin to relax. The next O2 binding event does the same, causing even more Hb relaxation. - O2 binding in the binding pocket of one subunit causes localized movement of the proximal His, which causes a cascade of movement throughout the subunit leading to major global rearrangement of the Hb that facilitates the next O2 binding to the next subunit. pH effect on oxygen binding to hemoglobin - - Actively metabolizing tissues generate H+, lowering the pH of the blood near the tissues relative to the lungs Hb affinity for oxygen depends on the pH o H+ binds to Hb and stabilizes the T state o Protonates His146 which then forms a salt bridge with Asp94 o Leads to the release of oxygen (in the tissues) The pH difference between the lungs and the metabolic tissues increases efficiency of the O2 transport Bohr effect: Caused by a drop in pH which reduces Hb affinity for oxygen. Hemoglobin and CO2 export - Co2 is produced by metabolism in tissues and must be exported 15-20% of carbon dioxide is exported in the form of a carbamae on the amino terminal residues of each of the polypeptide subunits Consequence- stabailization of T-state. Why? - The formation of a carbamate yields a proton (H+) which can further contribute to the Bohr Effect. The carbamate forms additional salt bridges stabilizing the T state The rest of the carbon dioxide is exported as dissolved bicarbonate - Formed by carbonic anhydrase and is also producing a proton 2,3-Bisphoshoglycerate regulates oxygen binding (BPG) - Negative hetrophic regulator of Hb function Present at mM concentrations in erythrocytes o Produced from and intermediate in glycolysis Small negatively charged molecule binds to the positively changed central cavity of Hb Stabilizes the T state Sickle cell anemia is due to a mutation in hemoglobin - Glu6àVal in the B chain of Hb The mutated valine side chain makes the Hb “sticky” and binds another Hb and so on, forming fibers. This sickles the red blood cells. This is the first example of a molecular disease. Antibodies are immunoglobulins - Made up of twoi pairs of peptide chains: one pair of identical latheg (heavy) chains and one pair of identical small (light) chains Antigens bind via induced fit - Antigen binding causes significant structural changes to the antibody Covid-19 spike Protein antigen - - Moderna and Pfizer vaccines code for the Covid-19 spike protein allowing our cells to make the spike protein antigen and allowing our immune systems to make antibodies against the spike protein before we get infected. ELISE: detect and quantitate a ligand of interest in a sample Motor proteins can shift conformations to move - Motor proteins require energy to function-usually ATP - ATP binding and hydrolysis causes conformational change that elicit work Myosin thick filaments slides alone actin thin filaments - Muscle contractions are driven by differential binding of myosin heads to acin filaments depending on whether bound to: ATP, ADP+Pi, ADP only Bindi g, ATP hydrolysis and realeses of ADP and Pi cause four different conformations and movement t (along actin filament) Common to motor proteins- multiple conformations that are significant to cause movement of protein What do proteins do? Signal transduction: detect, transform, and transmit information - Growth factor is a small protein released by cells to trigger other cells to divide Binding of growth factor causes receptor to change conformation and dimerize Signaling: Intracellular part now binds other proteins to trigger cell division Cancer is often caused by mutations that make growth factor receptor signaling too active There are thousands of cell surface receptors – each for a different signal Enzymes: Thermodynamics - Favorable reaction: Exergonic (-DG) Unfavorable: Endergonic (+DG) DG=DH-TDS Why enzymes? - Life is metastable and it is controlled kinetically by enzymes. Most biological reactions are slow. o Enzymes are catalysts that sped up just the right reactions at the right time. Biological reactions - - ATP hydrolysis is highly exergonic (-30.5 kj/mol) o Is ATP unstable? o Yes and no it is metastable it is thermodynamically unstable (highly negative DG) o But kinetically stable (large activation energy DG) Enzymes that hydrolyze ATP speed up this reaction when required and where required. What are enzymes? - Enzymes are biological catalysts. o increase reaction rates without being used up - Most enzymes are globular proteins o However, some RNA (ribozymes and ribosomal RNA) also catalyze reactions Properties of enzymes: 1. They speed up rxns by lowering the activation energy required for the rxns to proceed. 2. They bind their substrate with specificity. 3. They are NOT changed or used upon by the reactions they promote. a. Thus one enzyme can convert MANY substrate molecules to products Important enzyme terms: Enzymes bind specific substrates, catalyze a reaction, release products. Enzyme catalyst: speeds up reaction without itself being destroyed thus one enzyme can convert many substrates molecules to product Substrate: bind specifically in enzyme active site, and is converted to a product Co factors: inorganic like a metal ions (mg++) that are requires for some enzymes to function. Co-enzymes: small organic molecules (ATP,NADH) which are required for some enzymes to function. Apo-enzyme: an enzyme that requires a co-factor/co-enzyme, in the absence of cofactor. Holo-enzyme: apoenzyme cofactor/coenzyme Enzyme Classification Oxidoreductases- catalyze oxidation/reduction reactions like dehydrogenases such as ADH Transferases- transfers a functional group such as acetyl,methyl or a phosphate group (kinases,methylases,polymerases Hydrolases- addition or removal of water (nucleases, proteases, enolase) Lyases (synthases)-don’t use water or oxidatation to cleave (or form) bonds (decarboxylases or aldolases) Isomerases – rearrange molecules (phosphohexone isomerase) Ligases (synthetases) – ligate 2 molecules togther by reaction coupled to ATP hydrolysis (DNA ligase, RNA ligase aminoacyl-tRNA synthetase) 1.) Enzymes lower the activation energy of reaction How does this work? - The enzyme: 1. Binds reactants 2. Stabilizes the deformation of chemical bonds as they are rearranged to form a product. 3. Releases the product 4. Repeats at 1 2.) Emnzymes are specific - - Active site: the site where the substrate binds The active site is complemetray to the substrate o It has the right shape and charge for the susbtrate to bind The active site is specific to the substrate o Only 1 or few substrates are able to bind properly. Other molecules are excluded because they are not complementary to the size,shape,charge of the active site Substrate binding: induced fit Induced fit: change in shape of enzyme upon substrate binding-making the interaction even stronger. 3) enzymes are not used up or changed by reaction - An enzyme speeds up a reaction without being used up or destroyed. Thus, one enzyme can convert MANY substrates to product. Enzymes (protwins) have two main attributes that set them apart from nonbiological catalysts: 1. Enxymes are very specific for the reactions they catalyze (onluy bind 1-2 substrates) 2. Enzyme activity is regulated which means it can turn on and off How do enzymes work? How to speed reactions up – in a test-tube vs in the cell - - Higher temperatures o NO: not goof in the cell (macromolecules would fall apart) Higher concentration of reactants o NO/sometimes: wasteful and frequntluy too costly in the cell-starting materials (glucose) can be limited/valuable/rare) Couple an unfavorable reaction to a favorable reactiom o YES: Energy coupling is universally used by living organisms Lower activation barrier by catalysis o YES: enzymes are universally used by living organisms Energy coupling - - Many enezymes function by allowing for chemical coupling of exergonoic and endergonic reactions in thei active site o In phosphorylation of glucose by hexokinase, hydrolysis of ATP (favorable) drives the phosphorylation of glucose (unfavorable) Use of ATP hydrolysis to “do work” is a common motif in biology o Many enzymes have ATP binding sites o Thus ATP is a “shared intermediate” o “energy currency” An enzyme affe ts the rate of reaction, NOT ist equilibrium Reactions – Equilobrium vs Rates S-> P is this reaction faveorable? DG says nothingh about how fast a reaction will occur Keq= Equilibrium onstant for this reaction DG= standard state free energy change of reaction at (pH 7, 25°C) - RT at 25°C = 2.5 kj/mol ΔG’o = -RT ln K’ eq Reaction rate: V=k[S] The smaller DG the faster k Transition State (‡) à A ppoint at which decay to S or P is equally likely - Transient and highly unstable Example of transistion state Linus Pauling found oyt how to lower DG - Enzyme active site is complementary to the transition state of the reaction Enzyme have some affinity for its substrate BUT Enzymes bind transition states much better. Stronger/additional interactions with the transition state as compared to the ground state lower the activation barrier Stickase: Enzyme catalytic mechanisms General idea: Transition state stabilization Enzyme mechanism examples - Chymotrypsin (catalytic, acid/base, induced fit) Lysozyme (catalytic & acid/base) Hexokinase (induced fit) Alcohol dehydrogenase (acid/base and metal) Electrophile: An electron-deficient group with a strong tendency to accept electrons from an electron-rich group (nucleophile). Nucleophile: An electron-rich group with a strong tendency to donate electrons to an electron-deficient nucleus (electrophile); the entering reactant in a bimolecular substitution reaction. Lewis base: Presence of high electron density centers (Donor) Lewis Acid: Prescence of low electron density centers (Acceptor) Acids: Proton (H+) donors, electron pair acceptors Bases: Proton acceptors, electron pair donors. Acid base catalysis - Without catalysis unstable (charged) intermediate breaks down rapidly to form reactants. Intermediate has very high energy (unstable because of charge) Water catalyzes the reaction by acting as acid and base and thus neutralizing the charge on the intermediate. When acid or base contributed by something else (ie amino-acid functional group or by buffer) General acid-base catalysis is needed when proton transfer from H2O is slower that the rate of the breakdown of intermediates (back to substrate). The presence of another proton donor (HA) or acceptor (B): is then needed to catalyze the reaction. Why use General vs Specific acid-base cafalysis? - Specific acid base catalysis: pH dependent. In cells the pH is around 7. Perhaps that’s not enough hydroxyls or protons for catalysis. Enzyme cobcentration may be close to 10-4 and 10-5 M. So use genral acid-base catalysis, where amino acid group in enzyme is the catalyst. Amino acids commonly involved in Genergal A-B catalysis - Glu, Asp, Lys, Arg, Cys, His, Ser, Tyr - Serines side chain is not usually ionizable- its hard to deportanate an alcohol. o So, the R-OH of single Serine in solution has pKa = 15-19 o However inside certain proteins these pKa values sometimes can be shifted dramatically ( the active sit serine in chymotrypsin has pKa 7) Enzyme catalytic mechanisms How do genral acids and bases help increase rate of reaction? - General acid and base catalysis avoids need for extremely high or low pH Make a reactive group more reactive by increasing nucleophilic and electrophilic character of another atom. Set up formation of intermediate and then also help break it down pH profile of Enzyme Kinetics There are two reasons for the decrease in activity of this enzyme at pHs above and below pH 8. What are they? - Structural: The amino group has to stay protonated as to not denature the protein. Functional: Changes the charges of the side chains (cant bind ligand). Covalent catalysis: Make transient covalent bonds - Bond between the enzyme and the substrate Changes the reaction pathway. - Requires a nucleophile on the enzyme. o Can be reactive serine, thiolate, amine or carboxylate. Example of Acid-Base and Covalent Catalysis in chymotrypsin Metal ion catalysis: use redox cofactors, pKa shifters. - Involves a metal ion bound to the enzyme. Interacts with substrate to facilitate binding. o Stabilizes negative charges. Participates in redox reactions. Chymotrypsin - serine protease - it accelerates peptide bond hydrolysis by 109. Specificity: Armoatic residues (Trp, Phe, Tyr) – cleaves them in proteins at their carboxyl end. Catalytic Triad: Hydrogen-onded network of 3 residues in active site that are critical for mechanism: Ser, His, and Asp Serine Proteases - - - Part 1 (fast) o Serine made more reactive by removal of proton by histidine o Ser attacks peptide bond to make aceyl-enzyme intermediate with N-terminal portion of peptide (ending in the aromic aa) o C-terminal portion of substrate hydrolyzed off Part 2 (slow) o Water made more reactive by removal of proton His o Water attacks acyl-enzyme intermediate at carbonyl and cleaves peptide, resulting in Ser-OH and released peptide (attacking water forms the carboxyl group) Burst phase: very fast release of p-nitrophenol = rapid 1st step. (first product of reaction) Overview step 1: - Asp: H-bond stabilization of His His pulls H from Ser OH (general base catalysis) Substrate binds (aromatic side-chain binds. Induced fit of hydrophobic pocket (binding) induced fit) Activated O- nucleophilic attack (covalent catalysis) Oxyanion hold stabilization of transition state. Amine leaving group stabilized by H from His (general acid) Cleaved off c-term segment substrate exits the active site Serine is still covalently attached to the C-term end of N-term segment of the substrate Overview step 2: - H2O enters the active site and forms a H-bond with His His pulls proton off the H2O (general base catalysis) thus creating reactive OHOH- nucleophilic attack at Ser-substrate bond. Ser is no longer covalently attached to substrate. Ser O- is reprotonated by His. Product floats away. Reaction is complete Chymotrypsin take home questions: 1. Know the roles of the various amino-acids in the catalytic triad and why they behave the way they do Asp – 102 à interacts with the histidine residue and positions it in the proper orientation Ser – 195 à Can nucleophilic attack the carbon of the carbonyl group found on the substrate molecule. His – 57à Can interact with the alcohol group of serine, by pulling away the hydrogen ion from the alcohol, histidine transforms serine from a poor nucleophile (alcohol) into a good nucleophile (alkoxide). They all carry out the catalytic function of breaking peptide bonds 2. Demonstrate uses of general acid-base catalysis and covalent catalysis. General-acid base catalysis: - His pulls H from Ser OH His pulls proton off the H2O thus creating reactive OH- Covalent catalysis: - Activated O- nucleophilic attack Formation of a covalent bond between the enzyme and at least one of the substrates involved in the reaction. 3. Explain selectivity - roles for hydrophobic pocket. - The hydrophobic pocket in chymotrypsin contributes to its selectivity by providing a favorable environment for the binding of substrates containing bulky hydrophobic residues. This selective binding allows it to efficiently cleave peptide bonds adjacent to these residues, demonstrating its role in substrate specify and enzymatic function. 4. Explain role of oxyanion pocket. The oxyanion pocket stabilizes reactions intermediates during catalysis. 5. What’s the slow step? a. Water made more reactive by removal of proton His b. Water attacks acyl-enzyme intermediate at carbonyl and cleaves peptide, resulting in Ser-OH and released peptide (attacking water forms the carboxyl group) 6. What’s the role of water - Serves as nucleophiles, forming hydrogen bonds, facilitating hydrolysis reactions, and contributing to the solvent environment necessary for enzyme function. Alcohol Dehydrogenase Liver ADH catalysis of oxidation of EtOH to Acetaldehyde: ADH has a co-enzyme (NAD+) - His abstracts proton from Ser (base) - Ser-O- abstracts proton from ethanol (base). Zn 2+ (bound metal) stabilizes TS negative charge. NAD+ oxidizes ethanol to acetaldehyde. NAD+ is reduced to NADH. Enolase Metal ions that stabilize binding of substrate and increase acidity of proton: Enolsae (penultimate step of glycolysis) Reaction catalyzed by Enolsae: Mechanism of enolase catalysis using Acid-Base and Metal Ion catalysis Peptidoglycan and Lysozyme - Peptidoglycan is a polysaccharide found in many bacterial cell walls. Lysozyme- antibacterial enzyme (in tears) that breaks the glycosidic bonds of the cell wall. Cleavage of the cell wall leads to the lysis of bacteria (by osmotic shock). Cleavage of Peptidoglycan by Lysozyme: Two successive steps Step 1: - Asp 52 acts as a nucleophile in the first step Glu 35 is protonated (b/c of a shifted pKa in the active site) and acts as a general acid to protonate the leaving group in the trasnsition state. Step 2 - Water hydrolyzes the covalent glycosyl-enzyme intermediate. Glu 35 acts as a general base to deprotonate water in the second step. Induced Fit – hexokinase. - Glucose +ATP ó Glucose-6-phosphate +ADP Phosphorylation of glucose catalyzed hexokinase. Which undergoes conformational change, closing active site, upon binding glucose and ATP, triggering catalysis.