Bio Lec3 PDF
Document Details
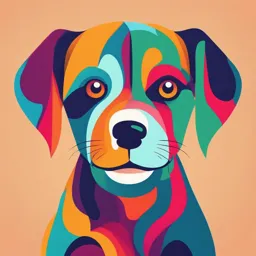
Uploaded by CuteLeaningTowerOfPisa1381
Tags
Summary
This document is a lecture about life and chemistry: large molecules, covering topics such as proteins, nucleic acids, carbohydrates, and lipids. The document provides definitions, structures, and functions of each compound. It also includes discussions on protein folding, and polymerization of nucleic acids.
Full Transcript
3 Life and Chemistry: Large Molecules 3 Intended Learning Outcomes (cont.) A1. Be familiar with how polymers are assembled and dismantled A2. Describe the properties of a Protein A3. Explain how proteins are structured. Be able to explain each level of...
3 Life and Chemistry: Large Molecules 3 Intended Learning Outcomes (cont.) A1. Be familiar with how polymers are assembled and dismantled A2. Describe the properties of a Protein A3. Explain how proteins are structured. Be able to explain each level of organization affects the shape and specificity of the protein A4. Describe the properties of a Nucleotide A5. Describe the differences in structure between major nucleic acids and how the structure correlates with function A6. Describe the properties of a carbohydrate A7. Explain how carbohydrates are categorized and be able to give examples of each kind of carbohydrate C1. Recognize that most organic macromolecules are polymers of smaller units 3-2 3 Macromolecules: Giant Polymers These macromolecules are made the same way in all living things, and are present in all organisms in roughly the same proportions. Macromolecules are giant polymers. Polymers are formed by covalent linkages of smaller units called monomers. Molecules with molecular weights greater than 1,000 Daltons (atomic mass units; 1kDa = 1000 Daltons) are usually classified as macromolecules. 3-3 3 Macromolecules: Giant Polymers The functions of macromolecules are related to their shape and the chemical properties of their monomers. Some of the roles of macromolecules include: - Energy storage - Structural support - Transport - Protection and defense - Regulation of metabolic activities - Means for movement, growth, and development - Heredity 3-4 Condensation and Hydrolysis of Polymers Macromolecules are made from The reverse reaction, in which smaller monomers by means of polymers are broken back into a condensation or dehydration monomers, is a called a reaction in which an OH from hydrolysis reaction. one monomer is linked to an H from another monomer. Energy must be added to make or break a polymer. 3 Macromolecules: Giant Polymers There are four major types of biological macromolecules: Ø Proteins Ø Nucleic acids Ø Carbohydrates Ø Lipids Of the four types only three form polymers: proteins – nucleic acids – carbohydrates. 3-6 3 3-7 3 1. Proteins 3 Proteins: Polymers of Amino Acids Proteins are polymers of amino acids. They are molecules with diverse structures and functions. Each different type of protein has a characteristic amino acid composition and order. Proteins range in size from a few amino acids to thousands of them. Folding is crucial to the function of a protein and is influenced largely by the sequence of amino acids. 3-9 3 Proteins: Polymers of Amino Acids An amino acid has four groups attached to a central carbon atom: - A hydrogen atom - An amino group (NH3+) - The acid is a carboxyl group (COO–). - Differences in amino acids come from the side chains, or the R groups. 3-10 3 Amino acids 20 different amino acids make up proteins At the pH found in cells (about 7.0), the 20 major amino acids found in organisms have the structural formulas shown here. The side chains are highlighted, and standard single-letter and three-letter abbreviations for each amino acid are given. 3-11 3 Amino acids For clarity, the carbon atoms in the ring structures of phenylalanine, tyrosine, tryptophan, and histidine are not shown; each bend in a ring is the site of a carbon atom. The hydrogen atoms in these structures are also not shown. A double line inside a ring indicates a double bond. 3-12 3 Amino Acid Polymers Amino acids are linked by peptide bonds Making a polypeptide chain. Peptide bonds are formed by dehydration reactions, which link the carboxyl group of one amino acid to the amino group of the next. The peptide bonds are formed one at a time, starting with the amino acid at the amino end (N-terminus). The polypeptide has a repetitive backbone (purple) to which the amino acid side chains (yellow and green) are attached. Note that the two amino acids linked by such a bond are not free to rotate around it; but the single bonds on either side of the peptide bond can rotate. Thus, the structure as a whole is flexible. 3-13 3 Proteins: Polymers of Amino Acids Proteins are synthesized by condensation reactions between the amino group of one amino acid and the carboxyl group of another. This forms a peptide linkage. Proteins are also called polypeptides. A dipeptide is two amino acids long; a tri-peptide, three. A polypeptide is multiple amino acids long. 3-14 3 Hemoglobin structure and sickle-cell disease Sickle-cell disease results from a single amino acid substitution (Glu 6 Val or E6V) in the protein hemoglobin Normal hemoglobin Sickle-cell hemoglobin Primary Val His Leu Thr Pro Glul Glu... Primary Val His Leu Thr Pro Val Glu... Exposed structure 1 2 3 4 5 6 7 structure 1 2 3 4 5 6 7 hydrophobic region Secondary Secondary and tertiary b subunit and tertiary b subunit structures structures a b Quaternary Hemoglobin A Quaternary a b structure structure a Hemoglobin S b a b Molecules interact with Function Molecules do Function one another to not associate crystallize into a with one fiber; capacity to another, each carry oxygen is carries oxygen. 10 µm 10 µm greatly reduced. Red blood Normal cells are Fibers of abnormal cell shape Red blood full of individual cell shape hemoglobin hemoglobin deform cell into molecules, each sickle shape. carrying oxygen 3-15 3 Proteins: primary level of organization There are four levels of protein structure: primary, secondary, tertiary, and quaternary. The precise sequence of amino acids is called its primary structure. Enormous numbers of different proteins are possible (20n, where n is the number of amino acids in the polypeptide chain). 3-16 3 In Overall Shape, Proteins Are the Most Diverse Class of Molecules Known. Remember, function follows from structure! Gene expression Transport Enzyme (catalysis) Structural support 3-17 3 Secondary level of organization Secondary structure - Is the folding or coiling of the polypeptide into a repeating configuration - Includes the a helix and the b pleated sheet (a) Hydrogen bonds can form between nearby amino and carbonyl groups on the same polypeptide chain. (b) Secondary structures of proteins result. (c) Ribbon diagrams of secondary structure 3-18 3 The a helix The a helix is a right-handed coil. The peptide backbone takes on a helical shape due to hydrogen bonds between every fourth amino acid. The R groups point away from the peptide backbone. Fibrous structural proteins have a-helical secondary structures, such as the keratins found in hair, feathers, and hooves. 3-19 3 The b pleated sheet b pleated sheets form from peptide regions that lie parallel to each other. Sometimes the parallel regions are in the same peptide, sometimes the parallel regions are from different peptide strands. This sheet-like structure is stabilized by hydrogen bonds between N-H groups on one chain with the C=O group on the other (NOT between side chains). Spider silk is made of b-pleated sheets from separate peptides. 3-20 3 Tertiary level of organization Tertiary Structure of Proteins Results from Interactions Involving R-Groups. (a) The overall shape of a single polypeptide is called its tertiary structure. This level of structure is created by bonds and other interactions that cause it to fold. (b) The tertiary structure of these proteins includes interactions between α-helices and β-pleated sheets. 3-21 3 Quaternary level of organization Quaternary structure - Is the overall protein structure that results from the Polypeptide chain aggregation of two or more polypeptide subunits Collagen b Chains Motifs and domains are additional structural characteristics: Amino acids are letters in the protein language, Iron motifs are words or phrases, and domains Heme are paragraphs. a Chains Hemoglobin 3-22 3 Levels of protein organization: Summary 3-23 3 Folding and Function Changes in temperature, pH, salt concentrations, and oxidation or reduction conditions can change the shape of proteins. This loss of a protein’s normal three-dimensional structure is called denaturation. 3-24 3 Normal Folding is Crucial to Function Protein Folding Is Often Regulated Misfolding Can Be “Infectious” Prion Infectivity Is Linked to Structure. Ribbon model of (a) a normal, noninfectious prion protein; and (b) the infectious form that causes mad Calmodulin Requires Calcium to Fold cow disease in cattle. Secondary Properly. Many proteins, like calmodulin, do not structure is represented by coils (α- complete their folding until after interacting with helices) and arrows (β-pleated sheets). ions or other molecules. Once calmodulin binds to calcium, it assumes its functional shape. 3-25 3 Chaperonins Chaperonins are protein molecules that assist in the proper folding of other proteins Correctly Polypeptide folded Cap protein Hollow cylinder Chaperonin (fully assembled) Steps of Chaperonin 2 The cap attaches, causing 3 The cap comes Action: the cylinder to change shape in off, and the properly 1 An unfolded poly- such a way that it creates a folded protein is peptide enters the hydrophilic environment for the released. cylinder from one end. folding of the polypeptide. 3-26 3 X-ray crystallography X-ray crystallography is used to determine a protein’s three-dimensional structure X-ray diffraction pattern Photographic film Diffracted X-rays X-ray X-ray source beam Crystal Nucleic acid Protein (a) X-ray diffraction pattern (b) 3D computer model 3-27 3 2. Nucleic Acids 3 What is a nucleic acid? A nucleic acid is a polymer of nucleotide monomers. Nucleotides are each composed of a phosphate group, a sugar, and a nitrogenous base. § The sugar is ribose in ribonucleotides and deoxyribose in deoxyribonucleotides. There are two groups of nitrogenous bases: § purines (adenine, guanine) § pyrimidines (cytosine, uracil, and thymine) Uracil (U) is found only in ribonucleotides, and thymine (T) is found only in deoxyribonucleotides. 3-29 3 Nucleotides, sugars and bases 3-30 3 Nucleotides Polymerize to Form Nucleic Acids Nucleic acids form when nucleotides polymerize. A condensation reaction forms a phosphodiester linkage (phosphodiester bond) between the phosphate group on the 5′ carbon of one nucleotide and the –OH group on the 3′ carbon of another. Types of nucleotides involved: 1. Ribonucleotides, which contain the sugar ribose and form RNA 2. Deoxyribonucleotides, which contain the sugar deoxyribose and form DNA 3-31 3 Nucleotides Polymerize to Form Nucleic Acids 3-32 3 The Sugar-Phosphate Backbone Is Directional The sugar-phosphate backbone of a nucleic acid is directional—one end has an unlinked 5′ carbon, and the other end has an unlinked 3′ carbon. The nucleotide sequence is written in the 5′ ® 3′ direction. This reflects the sequence in which nucleotides are added to a growing molecule. This nucleotide sequence comprises the nucleic acid’s primary structure. 3-33 3 The Polymerization of Nucleic Acids Is Endergonic Polymerization of nucleic acids is an endergonic (requires energy input) process catalyzed by enzymes. Energy for polymerization comes from the phosphorylation of the nucleotides. § Phosphorylation is the transfer of one or more phosphate groups to a substrate molecule. This raises the potential energy of the substrate and enables endergonic reactions. In nucleic acid polymerization, two phosphates are transferred, creating a nucleoside triphosphate. Activated Monomers Drive Polymerization Reactions. Polymerization reactions are generally nonspontaneous, but those reactions involving nucleoside triphosphates, such as ATP, are spontaneous. The potential energy stored in activated nucleotides is released when the pyrophosphate (PPi) is removed before the polymerizing condensation reaction. 3-34 3 Watson and Crick’s Model of DNA’s Secondary Structure James Watson and Francis Crick determined: 1. DNA strands run in an antiparallel configuration. 2. DNA strands form a double helix. q The hydrophilic sugar-phosphate backbone faces the exterior. q Nitrogenous base pairs face the interior. 3. Purines always pair with pyrimidines. q Specifically, strands form complementary base pairs A-T and G-C. Ø A-T have two hydrogen bonds. Ø C-G have three hydrogen bonds. 4. DNA has two different sized grooves: the major groove and the minor groove. 3-35 3 Complementary Base Pairing Is Based on Hydrogen Bonding. 3-36 3 The Secondary Structure of DNA Is a Double Helix DNA’s secondary structure consists of two antiparallel strands twisted into a double helix. The molecule is stabilized by hydrophobic interactions in its interior and by hydrogen bonding between the (a) The cartoons illustrate complementary base complementary base pairing and how strands are twisted into a double pairs A-T and G-C. helix. (b) The space-filling model shows tight packing of the bases inside the double helix. The double-helix structure explains the measurements inferred from X-ray analysis of DNA molecules. 3-37 3 DNA Contains Biological Information Closely related living species have DNA base sequences that are more similar than distantly related species. The comparative study of base sequences has confirmed many of the traditional classifications of organisms. DNA comparisons confirm that our closest living relatives are chimpanzees: We share more than 98 percent of our DNA base sequences. 3-38 3 RNA structure and function Like DNA, RNA has a primary structure consisting of a sugar- phosphate backbone formed by phosphodiester linkages and, extending from that backbone, a sequence of four types of nitrogenous bases. The primary structure of RNA differs from DNA in two ways: 1. RNA contains uracil instead of thymine. 2. RNA contains ribose instead of deoxyribose. q The presence of the –OH group on ribose makes RNA much more reactive and less stable than DNA. 3. Hydrogen bonding between ribonucleotides in RNA can result in complex three-dimensional shapes that can have catalytic functions! 3-39 3 RNA Secondary Structure RNA’s secondary structure results from complementary base pairing. The bases of RNA typically form hydrogen bonds with complementary bases on the same strand. The RNA strand folds over, forming a hairpin structure: the bases on one side of the fold align with an antiparallel RNA segment on the other side of the fold. RNA molecules can have tertiary and quaternary structures. Hydrogen Bonding in RNA Certain RNA molecules called ribozymes can act as catalysts. Since RNA can be The discovery of ribozymes provided a informational and catalytic, solution to the question of whether proteins or it could have acted as a nucleic acids came first when life originated. catalyst for its own replication as well as for the synthesis of proteins. 3-40 3 3. Carbohydrates 3 Carbohydrates Carbohydrate polymers are called glycans Carbohydrates serve as fuel and building material Carbohydrates - Include both sugars and their polymers 3-42 3 Sugars: 1. Monosaccharides Monosaccharides Triose sugars (C3H6O3) Pentose sugars (C5H10O5) Hexose sugars (C6H12O6) - Are the simplest H C O H C O H C O H C O sugars H C OH H C OH H C OH H C OH Aldoses H C OH H C OH HO C H HO C H H H C OH H C OH HO C H - Can be used for Glyceraldehyde H C H OH H H C C OH OH H H C C OH OH fuel Ribose H H Glucose Galactose H H H - Can be converted H C OH C O H C OH C O H C OH C O into other organic H C OH H C OH HO C H Ketoses molecules H Dihydroxyacetone H H C OH C OH H H C OH C OH H H C OH Ribulose H - Can be combined Fructose into polymers 3-43 3 Sugars: 1. Monosaccharides Monosaccharides - May be linear - Can form rings that predominate in aqueous solutions H O 1C 6 CH2OH 6CH OH 2 2 CH2OH H C OH 5C O H 5 C O 6 3 H H H H H O HO C H 5 H 4C H 1 4C H 1C H 4 OH C 4 1 H OH H OH H C OH O HO OH 3 2 OH 2C OH 3 2 OH H 5 C OH 3 C C C H OH 6 H OH H OH H C OH H (a) Linear and ring forms. Chemical equilibrium between the linear and ring structures greatly favors the formation of rings. To form the glucose ring, carbon 1 bonds to the oxygen attached to carbon 5. 3-44 3 Sugars: 2. Disaccharides § Consist of two monosaccharides § Are joined by a glycosidic linkage (a) Dehydration reaction in the synthesis of maltose. The bonding CH2OH CH2OH CH2OH CH2OH of two glucose units O H O O O forms maltose. The H H H H H H 1–4 H H H H H 1 glycosidic 4 glycosidic link joins O O the number 1 carbon OH H OH HO OH H H linkage H HO OH H H of one glucose to the HO O OH number 4 carbon of the second glucose. H OH H OH H OH H OH Joining the glucose H2O monomers in a Glucose Glucose Maltose different way would result in a different disaccharide. CH2OH CH2OH CH2OH CH2OH H O H O H O H 1–2 O H H H glycosidic H O O 1 2 H O HO H linkage H HO (b) Dehydration reaction H H H in the synthesis of H H HO HO O CH2OH CH2OH sucrose. Sucrose is O a disaccharide formed H O OH H H OH OH H from glucose and fructose. H Notice that fructose, H2O though a hexose like Glucose Fructose Sucrose glucose, forms a five-sided ring. 3-45 3 Polysaccharides Polysaccharides - Are polymers of sugars - Serve many roles in organisms - Storage polysaccharides, e.g. starch and glycogen - Structural polysaccharides, e.g. cellulose and chitin - Glycoproteins – proteins joined to carbohydrates by covalent bonds. Glycoproteins are key molecules in cell-cell recognition and cell-cell signaling. Each cell in your body has glycoproteins on its surface that identify it as part of your body. 3-46 3 Storage Polysaccharides: 1. Starch Starch Chloroplast Starch - Is a polymer consisting entirely of a-glucose monomers (see figure below) - Is the major storage form of glucose in plants 1 µm Amylose Amylopectin α and β glucose ring structures. These two (a) Starch: a plant polysaccharide interconvertible forms of glucose differ in the placement of the hydroxyl group (highlighted in blue) attached to the number 1 carbon. 3-47 3 Storage Polysaccharides: 2. Glycogen Mitochondria Glycogen granules Glycogen - Consists of a- glucose monomers 0.5 µm - Is the major Glycogen storage form of glucose in animals (b) Glycogen: an animal polysaccharide 3-48 3 Structural Polysaccharides: 1. Cellulose Cellulose H O CH2O C CH2O H H O H H C OH H O OH H H H 4 OH H HO C H 4 1 OH H HO OH HO H H C OH - Is an unbranched H OH H C OH H OH polymer of b-glucose a glucose H C OH b glucose (a) a and b glucose ring structures CH2O CH2O CH2O CH2O - Has different H O H O H O H O glycosidic linkages HO OH 1 O 4 OH 1 O 4 OH 1 O 4 OH 1 O than starch OH OH OH OH (b) Starch: 1– 4 linkage of a glucose monomers CH2O CH2O OH OH H H O O O OH O OH OH 1 4 O OH HO OH O O CH2O CH2O OH OH H H (c) Cellulose: 1– 4 linkage of b glucose monomers 3-49 3 Structural Polysaccharides: 1. Cellulose § Is a major component of the tough walls that enclose plant cells 3-50 3 Structural Polysaccharides: 1. Cellulose Cellulose is difficult to digest - Cows have microbes in their stomachs to facilitate this process 3-51 3 Structural Polysaccharides: 2. Chitin Chitin, another important structural polysaccharide - Is found in the exoskeleton of arthropods - Can be used as surgical thread CH2O H H O OH H OH H OH H H NH C O CH3 (a) The structure of the (b) Chitin forms the exoskeleton (c) Chitin is used to make a chitin monomer. of arthropods. This cicada strong and flexible surgical is molting, shedding its old thread that decomposes after exoskeleton and emerging the wound or incision heals. in adult form. 3-52 3 Representative Polysaccharides 3-53 3 4. Lipids 3 Lipids are a diverse group of hydrophobic molecules Lipids - Are the one class of large biological molecules that do not consist of polymers - Share the common trait of being hydrophobic - Lipids store large amounts of energy - 9 kcal/gram due to energy rich fatty acid (FA) chain - Characteristics of fat - Hydrophobic because of nonpolar FA chain - Saturated versus unsaturated fats. - Saturated FA chains contain no double bonds; unsaturated FA chains can form more, cis- or trans- double bonds - Functions of fat - energy storage, insulation, cushioning 3-55 3 Lipids: Water-Insoluble Molecules Roles for lipids in organisms include: § Energy storage (fats and oils) § Cell membranes (phospholipids) § Capture of light energy (carotinoids) § Hormones and vitamins (steroids and modified fatty acids) § Thermal insulation § Electrical insulation of nerves § Water repellency (waxes and oils) 3-56 3 Kinds of lipids: 1. Fats (triglycerides) Triglycerides are constructed from two types of smaller molecules, a single glycerol and usually three fatty acids 3-57 3 Kinds of lipids: 1. Fats (triglycerides) Fatty acids vary in the length and number and locations of double bonds they contain 3-58 3 Kinds of lipids: 2. Phospholipids Phospholipids § Have only two fatty acids § Have a phosphate group instead of a third fatty acid CH22 + Hydrophilic head CH N(CH ) 3 3 Choline CH2 O O P O– Phosphate Phospholipids CH2 O consist of a CH CH2 Glycerol O O hydrophilic „head“ C O C O and a hydrophobic „tail“. Fatty acids Hydrophobic tails Hydrophilic head Hydrophobic tails (c) Phospholipid (a) Structural formula (b) Space-filling model symbol 3-59 3 Kinds of lipids: 2. Phospholipids The structure of phospholipids results in a bilayer arrangement found in cell membranes 3-60 3 Kinds of lipids: 3. Steroids § Steroids are signaling molecules § Are lipids characterized by a carbon skeleton consisting of four fused rings; steroid types vary depending on functional groups attached to ring system § One steroid, cholesterol, q Is found in cell membranes q Is a precursor for some hormones H3C CH3 CH3 CH3 CH3 Figure 5.15 HO 3-61 3 All Steroids Have the Same Ring Structure 3-62 3 Lipids: 4. Carotenoids Carotenoids are light-absorbing pigments found in plants and animals. One, b-carotene, is a plant pigment used to trap light in photosynthesis. In animals, this pigment, when broken into two identical pieces, becomes vitamin A. 3-63