Molecular Biology of the Cell - Chapter 10 - Membrane Structure PDF
Document Details
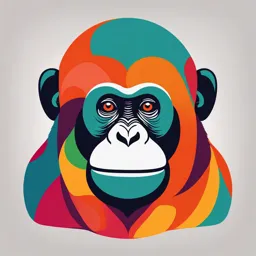
Uploaded by PreeminentZeugma
2008
Alberts, Johnson, Lewis, Raff, Roberts, Walter
Tags
Summary
This document is a chapter from a textbook entitled Molecular Biology of the Cell, fifth edition. It focuses on membrane structure, function, and its components, such as lipids and proteins, providing a detailed overview for students.
Full Transcript
Alberts Johnson Lewis Raff Roberts Walter Molecular Biology of the Cell Fifth Edition Chapter 10 Membrane Structure Copyright © Garland Science 2008 Biological membranes tend to form bilayers studded...
Alberts Johnson Lewis Raff Roberts Walter Molecular Biology of the Cell Fifth Edition Chapter 10 Membrane Structure Copyright © Garland Science 2008 Biological membranes tend to form bilayers studded with various membrane-bound & transmembrane proteins The fluid nature of the cell membrane allows lateral movement of many lipid and protein components; a feature which is described in the Fluid Mosaic Model Figure 10-1 Molecular Biology of the Cell (© Garland Science 2008) Overview of Membrane Functions Compartmentalization – Membranes form continuous sheets that enclose intracellular compartments. Scaffold for biochemical activities – Membranes provide a framework that organizes enzymes for effective interaction. Selectively permeable barrier – Membranes allow regulated exchange of substances between compartments. Overview of Membrane Functions Transporting solutes – Membrane proteins facilitate the movement of substances between compartments. Responding to external signals – Membrane receptors transduce signals from outside the cell in response to specific ligands. Intercellular interaction – Membranes mediate recognition and interaction between adjacent cells. Energy transduction – Membranes transduce photosynthetic energy, convert chemical energy to ATP, and store energy. Membrane & storage lipids Storage lipids = energy storage; dietary lipids (i.e., triglycerides) Phospholipid structure Phospholipids are the major lipid found in biological membranes Figure 10-2 Molecular Biology of the Cell (© Garland Science 2008) Fatty acids Saturated Unsaturated Effects on membrane fluidity?……. Lipid-ordered state Lipid-disordered state What effect does saturation vs. unsaturation of FA have on natural fats? On phospholipid membrane structure? Steroids Shape of lipids can determine Overall structure of “membrane” Figure 10-8 Molecular Biology of the Cell (© Garland Science 2008) Lipid content reflects function Membrane asymmetry Membrane Proteins What kind of structures allow for transmembrane domains? Membrane Proteins Alpha-helix or b-barrel are found in membrane proteins TM: a-hemolysin Transmembrane toxin Domain (b-barrel) Glycophorin Bacteriorhodopsin Hydrophobicity plot: predicts location of transmembrane domains in proteins a-helices b-sheets, i.e. barrels Some types of protein lipidation: importance is in localization of specific proteins to the membrane (i.e., during signal transduction pathway activation) Cytosol ER lumen Microdomains (lipid rafts) in plasma membrane Idea of localized signal transduction modules Alberts Johnson Lewis Raff Roberts Walter Molecular Biology of the Cell Fifth Edition Chapter 11 Membrane Transport of Small Molecules and the Electrical Properties of Membranes Copyright © Garland Science 2008 For simple diffusion: solutes will have different rates of diffusion depending upon solute polarity, size, & solute concentration gradient Figure 11-1 Molecular Biology of the Cell (© Garland Science 2008) Figure 11-2 Molecular Biology of the Cell (© Garland Science 2008) Types of membrane transport proteins Figure 11-3a Molecular Biology of the Cell (© Garland Science 2008) Types of membrane transport proteins Figure 11-3b Molecular Biology of the Cell (© Garland Science 2008) Active & Passive Transport Figure 11-4a Molecular Biology of the Cell (© Garland Science 2008) Figure 11-5 Molecular Biology of the Cell (© Garland Science 2008) Movement of electrically neutral solutes occurs “down” its concentration gradient (from high [S] to low [S]) until equilibrium is reached Net movement of electrically charged solutes is determined by a combination of electrical potential (Vm) & the chemical concentration difference across the membrane Equilibrium without an electrical potential across the membrane has equal particles and equal charge on both sides Simple diffusion: process of hydration shell removal is endergonic, so activation energy for diffusion through the membrane is high Just like M&M view of enzymes: T+Sout↔TS↔T+Sin T = transporter S = solute Transporter protein reduces activation energy for solute transport by forming noncovalent bonds with dehydrated solute to replace H-bonding with water and by creating a hydrophilic transmembrane passageway Figure 11-6 Molecular Biology of the Cell (© Garland Science 2008) Membrane Potentials Figure 11-4b Molecular Biology of the Cell (© Garland Science 2008) 3 ways of driving active transport Figure 11-7 Molecular Biology of the Cell (© Garland Science 2008) 3 types of transporter- mediated movement Figure 11-8 Molecular Biology of the Cell (© Garland Science 2008) Transporters (consider 3 examples) Glucose transporters: –GLUT1 uniporter –Facilitated diffusion (works with [glucose] gradient) Na+/K+ ATPase: –P-Type ATPase (channel becomes phosphorylated during transport) –Antiporter –Primary active transport (couples ATP hydrolysis [exergonic] with simultaneous movement of Na+ & K+ against their electrochemical gradients [endergonic]) ATP synthase: –F-Type ATPases (reversible, ATP-driven proton pumps: protons can either move against concentration gradient (i.e. certain bacteria) or with gradient (i.e., ATP synthesis through oxidative phosphorylation in mitochondria) –ATP synthase refers to scenario in which protons movement occurs with its gradient! GLUT1: glucose transporter A helical wheel diagram reveals an amphipathic a-helix Amphipathic a-helices a-helical supermolecular structures. Uniporter: glucose permease [Glucose] [Glucose] Low inside cell high outside cell No energy needed! Facilitated diffusion Insulin regulates glucose transporter expression on the cell surface Vm is the electrostatic potential difference across the membrane. Also denoted Dy or Df Equilibrium without an electrostatic potential across the membrane has equal particles and equal charge on both sides. However, if there is an electrostatic potential difference, then the steady state will have unequal number of particles and charges on each side. Na+/K+-ATPase: The Electrogenic Pump The term, electrogenic, refers to a transport-generated electrical potential. This usually results when movement of an ion without an accompanying counterion takes place. The Na+/K+ ATPase pumps Na+ outward to maintain the Na+ gradient that drives glucose uptake into the bloodstream Energy required To pump glucose From two sources: 1) [Na+]outside>>[Na+]inside 2) Transmembrane potential (inside-neg., so draws Na+ inward) …this means [glucose]inside/[glucose]outside ~ 9,000! Figure 11-11 Molecular Biology of the Cell (© Garland Science 2008) K+ binding Na+ binding constant constant Low High Low High High Low Low High ATP Synthase is the last step in the electron transport chain ATP Synthase A multisubunit transmembrane protein (450 kD) Two functional units, F1 and Fo Fo is a water-insoluble transmembrane proton pore F1 is a water-soluble peripheral membrane protein complex ATP Synthase Generates 1 ATP for every 3 protons that pass through it ENERGY COUPLING! Couples ATP synthesis (endergonic) with passive diffusion of protons through inner mito. Membrane (exergonic). Visualizing ATP Synthase Atomic C-subunits of Force F0 complex Microscopy From chloroplasts Norbert Dencher and Andreas Engel Hibernation The uncoupling of ETC from ATP synthesis Occurs in brown fat: many mitochondria and cytochromes Oxidation of NADH uncoupled from ATP synthesis The energy of ETC is released as heat! Also found in most newborn mammals The uncoupling of ETC from ATP synthesis Oxidation of NADH uncoupled from ATP synthesis Pore protein called thermogenin allows protons to flow down gradient The energy of ETC is released as heat! (instead of ATP)