Bio 120 Midterm Review PDF
Document Details
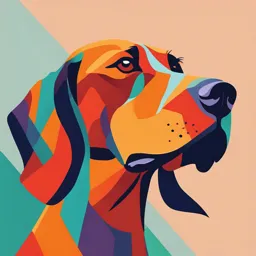
Uploaded by HeroicCherryTree8922
University of Saskatchewan
Tags
Summary
This document is a midterm review for Biology 120 at the University of Saskatchewan. It covers the cell theory, cell structure, and different types of microscopy.
Full Transcript
lOMoARcPSD|47304420 Bio 120 Midterm Review Biology 120 (University of Saskatchewan) Scan to open on Studocu Studocu is not sponsored or endorsed by any college or university Downloaded by isabella denoon ([email protected]) ...
lOMoARcPSD|47304420 Bio 120 Midterm Review Biology 120 (University of Saskatchewan) Scan to open on Studocu Studocu is not sponsored or endorsed by any college or university Downloaded by isabella denoon ([email protected]) lOMoARcPSD|47304420 BIOLOGY 120.3 – Chapter Midterm Review The Cell: An Overview KEY CONCEPTS: All organisms are made up of cells. All cells share some common structures/characteristics (unity), but there are some differences between cells within and between different organisms (diversity). LEARNING OBJECTIVES: The Cell Theory: All living organisms are composed of cells. The cell is the most basic unit of life. All cells arise only from pre-existing cells. How the cell theory underlies topics in biology: The cell theory helps define life—all living things are made of cells, and the cell is the basic unit of structure and function of a living thing. The cell has all the processes of an organism in miniature—digestion, respiration and reproduction. Basic features of cell structure and function shared by all living cells: DNA: the genetic material contained in one or more chromosomes and located in a nonmembrane bound nucleoid region in prokaryotes and a membrane-bound nucleus in eukaryotes. Plasma membrane: a phospholipid bilayer with proteins that separates the cell from the surrounding environment and functions as a selective barrier for the import and export of materials. Cytoplasm: the rest of the material of the cell within the plasma membrane, excluding the nucleoid region or nucleus, that consists of a fluid portion called the cytosol and the organelles and other particulates suspended in it. Ribosomes: the organelles on which protein synthesis takes place. Types of microscopy: 1. Light microscopy: Bright field – transmitted white light is used to illuminate samples; achieve magnifications of up to 1000x; can observe living specimens and stained sections of tissues. Differential interference contrast and phase contrast microscopes – use special lenses to enhance differences in density, giving a cell a 3D appearance; improves contrast when observing unstained living cells. Fluorescence microscopy – fluorescent dyes of antibody-dye complexes are used to label specific molecules or organelles within the cell; the microscope has filters that illuminate the cells with the particular wavelength of light that the fluorescent dye or protein can absorb. Downloaded by isabella denoon ([email protected]) lOMoARcPSD|47304420 2. Electron microscopy: Transmission electron microscopy – a beam of electrons is transmitted through an extremely thin section of a specimen; smaller organelles and other cell structures can be studied in detail using this microscope; magnification of up to 500,000x. Scanning electron microscopy – a beam of electrons is scanned over the surface of a specimen which results in a 3D image of the surface of a specimen; the object is coated with gold; can be used for intact specimens such as insects. 3. Microscope characteristics: Magnification – ratio between the size of an image produced by a microscope and its actual size Resolution – the minimum distance by which two points (object) can be separated and still be seen as two points Contrast – how different one structure looks from another (can be enhanced by the use of dyes) Why cells are small: Cells are subject to the surface to volume ratio; as cells get larger, their surface/volume ratio decreases For nutrient absorption since the speed of diffusion is faster It is easier to replace damaged/old/dying cells when there are many of the, Won’t disrupt functions when damaged Cells can reduce the limitations of the S/V ratio by: - Flattening, elongating or branching the cell, or having microvilli on the cell surface (they can increase the surface area of cells while minimizing an increase in cell volume. - Streaming of the cytoplasm in some cells can distribute nutrients around the cell without having to rely solely on diffusion to get nutrients to the cell center. Structure and function of the plasma membrane: The plasma membrane is made of a bilayer of lipid molecules called phospholipids as well as protein molecules. Downloaded by isabella denoon ([email protected]) lOMoARcPSD|47304420 It is selectively permeable to ions and organic molecules; it allows certain substances to cross the membrane more readily than others. The plasma membrane regulates the passage of substances into and out of the cell. Structures and activities that occur in a cell’s cytoplasm: The cytoplasm is the fluid region of the cell contained within the plasma membrane containing cytosol, organelles and cytoskeleton (which is made of protein fibres) Responsible for movement of cell contents and metabolic pathways such as glycolysis Purpose and significance of organelles in eukaryotic cells: Organelles are structures within a cell that perform specific functions like controlling cell growth and producing energy Examples of organelles found in eukaryotic cells include: the endoplasmic reticulum (smooth and rough ER), the Golgi complex, lysosomes, mitochondria, chloroplasts, cell wall, vacuoles, etc. Prokaryotic cells do not have membrane-based organelles. These cells can contain some non- membranous organelles like flagella, ribosomes and circular DNA structures called plasmids. Structure of a ribosome: Ribosomes are tiny cytoplasmic structures that are macromolecular machines, not organelles They are the site of protein (polypeptide) synthesis Each ribosome consists of two subunits that join together in the cytosol at the beginning of protein synthesis Synthesis of a protein on a ribosome is called translation Can be found on the nuclear envelope, rough ER, mitochondria, chloroplasts, cytosol, and floating free in the cytoplasm Structure and functions of organelles: Cell wall: an external layer of material surrounding the plasma membrane of cells in plants, fungi, bacteria and some protists; provides cell protection and support. Central vacuole: membrane bound organelle in plant cells that functions in storage of water, enzymes, ions, proteins, pigments and toxins; provides support to plant cells. Chloroplast: a chlorophyll-containing organelle where photosynthesis occurs; found only in plants and algae. Chromoplast: a plastid that contains pigments giving colour to flowers and fruits, making them attractive to animals. Cilia: short, hair like appendages that help with locomotion and sweeping fluid over the surface of the cell; made up of microtubules. Contractile vacuole: a membrane-bound organelle involved in osmoregulation in animal-like protists. Cytoplasm: fluid region of the cell contained within the plasma membrane containing cytosol, organelles and cytoskeleton; responsible for movement of cell contents and metabolic pathways. Cytoskeleton microtubules: hollow protein filaments; responsible for structure and movement of cilia and flagella, cell organization and shape; composed of the protein tubulin. Downloaded by isabella denoon ([email protected]) lOMoARcPSD|47304420 Microfilaments: the protein filaments that forms parts of the cytoskeleton; important in cell strength, shape and movement; responsible for pseudopodia formation in amoeba; composed of the protein actin. Flagella: long cellular appendage responsible for locomotion; made up of microtubules. Food vacuole: vacuole present in animal-like protists and some cells of multicellular animals; contains food particles engulfed by cell. Golgi apparatus: organelle made up of flattened, membrane bound compartments that modify, store, and ship products of the endoplasmic reticulum. Glycocalyx: a gelatinous outer covering which traps water and protects a bacterium form desiccation. Leucoplast: a type of plasmid that lacks pigment; different kinds of leucoplasts produce and store different macromolecules (starch, protein, fat). Lysosome: small membrane bound organelle that contains hydrolytic enzymes that digest a cells food and waste. Mitochondria: organelle that carries out cellular respiration and supplies most of the cell’s ATP. Nucleolus: a region in the nucleus of nondividing cells where rRNA transcription, processing, and ribosome assembly in eukaryotes occurs. Downloaded by isabella denoon ([email protected]) lOMoARcPSD|47304420 Nucleus: membrane bound organelle containing most of the cell’s genetic material; controls cell function; site of DNA synthesis and RNA transcription. Plasma membrane: the outer limit of the cytoplasm responsible for the regulation of substances moving into and out of the cell. Ribosome: a structure made up of rRNA and protein, found in the cytoplasm, synthesis protein. Rough ER: part of the ER that is studded with ribosomes; plays a role in the synthesis and sorting of proteins for export. Smooth ER: ER with no ribosomes that has various functions, including synthesis of lipids that become part of the cell membrane. Vesicle: a small, membrane bound structure that transfers substances between parts of the endomembrane system. The endomembrane system: The endomembrane system is a collection of internal membranes that form a single functional and developmental unit. Parts of the system are physically connected or indirectly connected by the movement of vesicles between organelles. Includes the endoplasmic reticulum, golgi apparatus, lysosomes, secretory vesicles, nuclear envelope, vacuolar membranes and plasm membrane New membrane is produced by the ER Membrane flows between parts of the system and to and from the plasma membranes via tiny vesicles. Membrane flows from the rough ER to the nuclear envelope through direct connections between these two organelles. Cell junctions: Connects cells and allows transfer of molecules Reinforce cell adhesion and provide avenues of communication. Anchoring junctions – common in cells subject to mechanical forces. - Desmosomes: linked to intermediate filaments - Adherents junctions: linked to actin filaments Tight junctions – direct fusion of proteins; tight connections form between adjacent cells by fusion of plasma membrane proteins on their outer surface. - seal space between cells where molecule absorption or leaking should be prevented Gap junctions – open channels that provide direct communication to cells of the same tissue; allows passage of ions and small molecules to flow between the cytoplasm of adjacent cells. Eukaryotic cytoskeletal elements: Downloaded by isabella denoon ([email protected]) lOMoARcPSD|47304420 Cytoskeleton: Network of protein fibres crisscrossing the cytoplasm Cytoskeletons are the movers and shapers within cells Provide mechanical support to the cell and help it maintain its shape (“cell bones”) Involved in cell motility, including movement of the whole cell and movement of organelles within the cell Cytoskeletal fibers are well-ordered polymers built from smaller protein subunits held together by noncovalent bonds Composed of three types of fibers: actin filaments, intermediate filaments, and microtubules Intermediate filaments: Assembled from a variety of proteins, collectively referred to as intermediate filament proteins Consist of eight protein chains wound together Provide structural support in many different kinds of cells and are associated with some types of anchoring junctions in animal cells Microfilaments: Two polymers of actin molecules wound around each other Have polarity Contractive elements in muscle cells Responsible for dividing the cytoplasm during cytokinesis in animal cells Actin and myosin are involved in producing amoeboid movement in amoeboid protozoans; also have been shown to be involved in producing cytoplasmic streaming in some cells Microtubules: Rudlike hollow cylinders formed from the helical polymerization of tubulin dimers formed from two different globular proteins called tubulin Originate from the centrosome Responsible for maintaining cell shape and organization, movement of certain cells such as flagella, and providing “tracks” for transport proteins to travel down Plasmodesmata: Downloaded by isabella denoon ([email protected]) lOMoARcPSD|47304420 These are tiny cytoplasmic connections that connect the cytoplasm of adjoining plant cells to each other The plasma membrane of one cell is continuous with the plasma membrane of the other cell through each plasmodesmata They are lined by plasma membrane Cytosol of the adjacent cells connect through the plasmodesmata Plasmodesmata are sites of rapid inter-cellular exchange between plant cells Motor proteins: Motor proteins are the driving force behind muscle contraction and are responsible for the active transport of most proteins and vesicles in the cytoplasm. Myosin motors act upon actin filaments to generate cell surface contractions and other morphological changes, as well as vesicle motility, cytoplasmic streaming and muscle cell contraction. The kinesin and dynein microtubule based motor superfamilies move vesicles and organelles within cells, cause the beating of flagella and cilia, and act within the mitotic and meiotic spindles to segregate replicated chromosomes. Prokaryotic vs eukaryotic cells: Downloaded by isabella denoon ([email protected]) lOMoARcPSD|47304420 Prokaryotic cells: simply organized, small and without membrane bound organelles; contain bacteria and archaea Eukaryotic cells: have a more complex organization, are larger and possess a variety of membrane bound organelles; contain plants, animals and fungi Subcellular fractionation: Subcellular fractionation is the process used to separate cellular components while preserving individual functions of each component Separation of cellular structures from one another is an important step for studying separate parts of the cell. This involves two steps: 1. Breaking open the membrane of the cell to get to the content inside 2. Separating the different organelle populations Differential centrifugation is also used to sort organelles into different fractions containing distinct organelle sub-populations characterized by their size, density and charge Plastids: 1. Leucoplast – colorless plastid Does not contain pigments One type of leucoplast, the amyloplast, stores starch Common in roots and tubers There are also leucoplasts that store proteins or lipids 2. Chromoplast – colored plastid Contain red, yellow and orange carotenoid pigments Important in coloring cells of ripening fruit and flowers Orange pigments are carotenes, yellow ones are xanthophylls Found in cells of some roots and in aging leaves 3. Chloroplast – colored plastid Plastids containing the green pigment, chlorophyll Chloroplasts are factories that use solar energy, carbon dioxide, water, and a few minerals to make carbs by a process called photosynthesis In plants they are most common in cells of leaves and young stems They contain a third set of internal membranes that surround sacs called thylakoids Downloaded by isabella denoon ([email protected]) lOMoARcPSD|47304420 The thylakoids are organized into stacks called grana; the liquid compartment around the thylakoids is the stoma Chloroplasts can also store carbs as starch grains in their stroma Cell Cycles KEY CONCEPTS: Cell division is essential for growth/repair of cells in multicellular organisms, and reproduction in bacteria, archaens, and some eukaryotes (e.g., asexual reproduction in yeast, amoebae, etc.). A parent cell yields essentially identical daughter cells in mitotic and bacterial cell divisions; these processes provide continuity of genetic information LEARNING OBJECTIVES: Mitosis: Mitosis is the division of the nucleus, resulting in the formation of two genetically identical daughter nuclei. The major functions of mitosis include the production and maintenance of multicellularity, asexual reproduction and replacement of old and/or damaged tissues. Bacterial cell division: Prokaryotic cells divide by a process called binary fission Downloaded by isabella denoon ([email protected]) lOMoARcPSD|47304420 Initially, the cell’s single circular chromosome will replicate; the two resulting chromosomes then separate, and the plasma membrane and cell wall grow inward, between the chromosomes, to divide the original cell into two identical daughter cells Eukaryotic nuclear division, mitosis, is more complicated than that of prokaryotic cell division largely because of the amount of nuclear material that must be divided among the new daughter cells The cell cycle: G1 Stage: Cell growth – new organelles and proteins are synthesized, and the plasma membrane increases in size S-stage: DNA synthesis – each single chromosome produces an exact copy of itself by DNA replication, forming a replicated chromosome (each copy is called a sister chromatid) G2 Stage: Cell growth – a second period of cell growth and final preparations for division M Stage: prophase, metaphase, anaphase, telophase and cytokinesis Downloaded by isabella denoon ([email protected]) lOMoARcPSD|47304420 Meiosis: Meiosis 1: Halves the number of chromosomes, i.e., diploid -> haploid Meiosis 2: Separates replicated chromosomes into single chromosomes (these cells are already haploid) Two situations occur in prophase 1 of meiosis that do not occur in prophase of mitosis: 1. Synapsis: when homologous chromosomes pair up to form tetrads 2. Crossing over: when genetic exchange occurs between homologous chromosomes Downloaded by isabella denoon ([email protected]) lOMoARcPSD|47304420 Chromosome numbers: Chromosome segregation: A major function of the centromere is attaching the microtubules in the mitotic spindle. In this function, the centromere directs the formation of the kinetochore, which is a special protein structure that attaches to the microtubules in the mitotic spindle. On each chromatid, the kinetochore forms at the centromere region of the DNA. Once all of the chromatids are attached to the mitotic spindle, the microtubules pull the sister chromatids apart into the two future daughter cells. Mitosis in animal vs plant cells: During cytokinesis, animal cells form a cleavage furrow that gives way to the formation of daughter cells. Plant cells do not form furrows, they form a cell plate instead to divide the cell Animal cells have centrioles that support the organization of mitotic spindle and completion of cytokinesis; plant cells do not have centrioles. How mitosis and bacterial cell division support the cell theory: The cell theory states that all cells arise from pre-existing cells; this is true because in mitosis and bacterial cell division, the cells must come from pre-existing cells. Mechanisms of chromosome movement: At one time, it was thought that microtubules pulled chromosomes toward the poles of the spindle Downloaded by isabella denoon ([email protected]) lOMoARcPSD|47304420 New information suggests chromosomes, themselves, walk along kinetochore microtubules using motor proteins on the kinetochore The tubulin subunits of the kinetochore microtubules are then disassembled behind the moving kinetochore Thus, kinetochore microtubules become shorter as the chromosomes are moved towards the poles of the spindle Definitions: Chromosome: thread-like structures in which DNA is tightly packaged within the nucleus. Ploidy: the number of sets of chromosomes found in a cell. Haploid: one of each type of chromosome in the nucleus (n) Diploid: two identical sets of chromosomes (2n) Chromatid: a chromosome that has been newly copied or the copy of such a chromosome. Centrosome: an organelle near the nucleus of a cell which contains the centrioles (in animal cells) and from which the spindle fibers develop in cell division. Centromere: structure in a chromosome that holds together the two chromatids Genetic Recombination KEY CONCEPTS: During sexual reproduction, mechanisms exist for the rearrangement of DNA which leads to genetic variability. After meiosis, the parent cell yields daughter cells containing half the chromosomes of the original cell. There are several mechanisms relating to creation of gametes that can lead to genetic diversity in eukaryotic offspring. LEARNING OBJECTIVES: Events of the meiotic phase: Prophase I: Downloaded by isabella denoon ([email protected]) lOMoARcPSD|47304420 Replicated chromosomes condense Nuclear envelope disintegrates Spindle microtubules begin to form Homologous chromosomes pair up to form tetrads by a process called synapsis Genetic exchange occurs between homologous chromosomes during a process called crossing over Metaphase I: Spindle microtubules attach to the centromeres attach to the centromeres of each replicated chromosome Tetrads line up along the mid-region (metaphase plate) of the cell Anaphase I: The homologous chromosomes separate from each other and are moved to opposite poles of the cell by the spindle apparatus Telophase I: Replicated chromosomes arrive at poles and begin to uncoil Spindle apparatus disappears Nuclear envelopes start to re-form Cytokinesis begins (cleavage furrow in animal cells or cell plate in plant cells) At the end of telophase 1, each daughter cell contains the haploid number of replicated chromosomes Prophase II: Replicated chromosomes condense again Nuclear envelope disintegrates again New spindle apparatus forms Metaphase II: Spindle microtubules attach to the centromeres of each replicated chromosome Replicated chromosomes line up individually along the metaphase plate of each of the daughter cells Anaphase II: Centromeres of each replicated chromosome separate, and the resulting single chromosomes are moved to opposite poles by the spindle apparatus Telophase II: Single chromosomes arrive at opposite poles and start to uncoil Spindle apparatus disappears Nuclear envelopes will re-form Nucleoli will become visible Cytokinesis begins Downloaded by isabella denoon ([email protected]) lOMoARcPSD|47304420 At the end of telophase II, there will be four daughter cells, each containing the haploid number of single chromosomes (n=2) Ploidy: Independent assortment: Describes how different genes independently separate from one another when reproductive cells develop; first discovered by Gregor Mendel Another feature of independent assortment is recombination. Recombination occurs during meiosis and is a process that breaks and recombines pieces of DNA to produce new combinations of genes. Contributions to genetic variability: Crossing over: Downloaded by isabella denoon ([email protected]) lOMoARcPSD|47304420 In this event, corresponding breaks occur on non sister chromatids of the bivalents; the corresponding pieces are then exchanged between the two chromatids Crossing over results in a piece of the maternal chromosomes added to the paternal chromosomes, and a piece of the paternal chromosome is added onto the maternal chromosomes Crossing over creates new genetic variation by combining some of the paternal and maternal characteristics together on the same chromatid Random assortment of chromosomes: When homologous chromosomes line up in metaphase I, their orientation towards the opposing poles is random The orientation of each bivalent occurs independently, meaning different combinations of maternal / paternal chromosomes can be inherited when bivalents separate in anaphase I Random fusion of gametes in fertilization: The fusion of two haploid gametes results in the formation of a diploid zygote This zygote can then divide by mitosis and differentiate to form a developing embryo As meiosis results in genetically distinct gametes, random fertilisation by egg and sperm will always generate different zygotes Defining Life and Its Origins KEY CONCEPTS: All living organisms share a number of basic characteristics we consider to be attributes of life. There Is evidence for life having potentially originated from the simple chemicals that make up biological macromolecules. LEARNING OBJECTIVES: Seven properties of life: Display order Harness and utilize energy Ability to reproduce Respond to stimuli Exhibit homeostasis Growth and development Ability to evolve Why viruses are not true living organisms: Viruses lack the properties of living things; they have no energy or metabolism, they do not grow, they produce no waste products and they do not respond to stimuli Since viruses don’t have organelles, nuclei, or even ribosomes, they don’t have the tools they need to copy their genes, much less create whole new virions Emergent characteristic of life: Downloaded by isabella denoon ([email protected]) lOMoARcPSD|47304420 Attributes of life result from emergent properties arising from a combination of simple interactions which, themselves do not have the properties of the higher levels of organization An emergent property is new and cannot be broken down into or analyzed solely in terms of its component parts (ex. The liver) Characteristics of Earth that made it suitable for development of life: The right ingredients: Earth has liquid water, an energy source and chemical building blocks like carbon, oxygen, hydrogen and nitrogen for life to thrive Earth is in a habitable zone: it is in the orbital region around a star where temperatures experienced on a planet in the zone would allow for the existence of liquid water Five overlapping stages proposed for the origin of life: Formation of organic molecules form from inorganic molecules occurred on the primordial Earth Linking together of monomers to form large organic molecules called polymers Enclosing of organic molecules into membrane-bound protobionts Initial information storage molecules in protobionts were RNA molecules initially but later DNA appeared and took over this role Appearance of first living cells Hypotheses for the origin of life: Reducing atmosphere hypothesis: Proposed by Aleksandr Oparin and J.B.S. Haldane They suggested there were more sources of high energy on the primordial Earth available to encourage reactions that built energy rich organic molecules from energy polar inorganic molecules Lacked molecular oxygen, but supposedly had an abundance of electrons and hydrogens that could enter into reactions with other molecules and create complex organic molecules No ozone layer; allowed UV rays from the sun into the lower atmosphere which helped provide energy to catalyze reactions They envisioned that these organic rich waters served as vast chemical laboratories powered by the ultraviolet light from solar energy forming polymers from the accumulating monomers Deep-sea vents: Deep sea ocean hydrothermal vents were discovered in 1977 along mid-oceanic ridges and scientists proposed that these vents were a likely location for the abiotic formation of life Gunter Wachershauser is a prominent advocate for the deep-sea hydrothermal vent hypothesis; he argues that the early chemistry necessary for the formation of organic molecules of life did not occur in bulk solutions in the surface oceans, but on mineral surfaces within recently discovered deep-sea hydrothermal vents Two kinds of vents have been identified: 1. Black smokers: - Hot, anoxic, acidic - Rich in inorganic molecules such as H2S, CH4 and NH3 (reducing atmosphere) 2. White smokers: - Not as hot. Anoxic, alkaline, water around these vents has slightly acidic - Rich in H2 and CH4 (reducing environment) - Electrochemical gradient created due to differences in vent water and surrounding water Downloaded by isabella denoon ([email protected]) lOMoARcPSD|47304420 - Considered to be the most favourable for life to originate in Extraterrestrial origin: Some scientists have hypothesized that the Earth was salted with an abundance of organic molecules from carbonaceous chondrite meteorites during the origin of the primordial earth Oxygenic photosynthesis on evolution of life: The origin of oxygenic photosynthesis was the most important metabolic innovation in Earth history. It allowed life to generate energy and reducing power directly from sunlight and water, freeing it from the limited resources of geochemically derived reductants. The release of O2 as an end product of water oxidation led to the rise of oxygen, which dramatically altered the redox state of Earth's atmosphere The biological availability of O2 also allowed for the development of complex multicellularity and aerobic respiration Endosymbiotic theory: One prokaryotic cell engulfed another prokaryotic cell These cells co-evolved; the engulfed cell was protected and would produce energy that could be utilized by the host cell The increased energy production led the way to the evolution of new cellular functions and organelles Both mitochondria and chloroplasts have their own genome, separate from that of the cells they occupy - mitochondria probably descended from an aerobic species of bacteria, while chloroplasts arose from Cyanobacteria - mitochondria and chloroplasts have a double membrane surrounding them - mitochondria are maternally inherited Evidence that supports this idea is the similarity between prokaryotic cells and mitochondria - Mitochondria have their own cell membranes, just like a prokaryotic cell does - Each mitochondrion has its own circular DNA genome, like a bacteria's genome, but much smaller. This DNA is passed from a mitochondrion to its offspring and is separate from the "host" cell's genome in the nucleus. - Mitochondria multiply by pinching in half — the same process used by bacteria. Every new mitochondrion must be produced from a parent mitochondrion in this way; if a cell's mitochondria are removed, it can't build new ones from scratch. Downloaded by isabella denoon ([email protected]) lOMoARcPSD|47304420 THE CELL: AN OVERVIEW SUMMARY Scientist contributions Robert Hooke: first observed the existence of cells from dead cork tissue; “Father of the Microscope.” Anton Von Leeuwenhoek: discovered the cells of protists which he called animalcules; build a series of single lens microscopes and observed many kinds of cells; “Father of Microbiology.” Mattias Schleiden and Theodor Schwann: Both realized that living organisms were made of cells; Schleiden saw components in plant tissues; Schwann saw cells in animal tissues. Rudolph Virchow: proposed that all cells must arise from previously existing cells (biogenesis). Robert Brown: first observed the nucleus. Basic features of cell structure and function Cells carry out the essential processes of life Cells contain highly organized systems of molecules, including the nucleic acids DNA and RNA, which carry hereditary information and direct the manufacture of cellular molecules. All forms of life can be divided into Bacteria, Archaea, and Eukarya. Some organisms in each domain are unicellular; this includes almost all bacteria and archaeans, some eukaryotes including protists such as the green algae Chlamydomonas, and some fungi such as yeast. Each of these cells is a functionally independent organisms capable of carrying out all activities necessary for living. If cells are broken open, the property of life is lost and they are unable to grow, reproduce, or respond to outside stimuli. Life as we know it does not exit in units more simple than individual cells. Viruses, which consist only of a nucleic acid molecule surrounded by a protein coat, cannot carry out most activities of life. Their only capacity is to infect living cells and direct them to make more virus particles of the same kind. Cells are visualized using a microscope Downloaded by isabella denoon ([email protected]) lOMoARcPSD|47304420 The two common types of microscopes are light microscopes, which use light to illuminate the specimen, and electron microscopes, which use electrons to illuminate the specimen. Magnification is the ratio of the object as viewed to its real size. Resolution is the minimum distance by which two points in the specimen can be separated and still be seen as two points. Contrast is how different one structure looks from another; can be enhanced by the use of dyes (stains). Why are most cells so small? The reason is because of how the surface area-to-volume ratio of a cell changes as it gets bigger. As cells increase in size, there is a point where the plasma membrane can not pass enough material across itself to meet the physiological needs of the increased cytoplasmic volume. Cells have a DNA-containing central region that is surrounded by cytoplasm All cells are bounded by the plasma membrane, a bilayer made of lipids with embedded protein molecules. The lipid bilayer is a hydrophobic barrier to the passage of water soluble substances, but selected water soluble substances can penetrate cell membranes through transport protein channels. The central region of all cells contains DNA molecules, which store hereditary information. The hereditary information is organized in the form of genes—segments of DNA that code for individual proteins. The central region also contains proteins that help maintain the DNA structure and enzymes that duplicate DNA and copy its information into RNA. All parts of the cell between the plasma membrane and the central region make up the cytoplasm. The cytoplasm contains the organelles, the cytosol, and the cytoskeleton. The cytosol is an aqueous solution containing ions and various organic molecules. The cytoskeleton is a protein-based framework of filamentous structures that helps maintain proper cell shape, and plays key roles in cell division and chromosome segregation. Many of the cell’s vital activities occur in the cytoplasm, including the synthesis and assembly of most of the molecules required for growth and reproduction and the conversion of chemical and light energy into forms that can be used by cells. Cells occur in prokaryotic and eukaryotic forms, each with distinctive structures and organization Within the prokaryotic cell that is a characteristic of both Bacteria and Archaea, the DNA- containing central region of the cell, the nucleoid, has no boundary membrane separating it from the cytoplasm. The eukaryotes make up the domain Eukarya and are defined by having cells in which DNA is contained within a membrane-bound compartment called the nucleus. STUDY BREAK QUESTIONS 1. What is the plasma membrane, and what are its main functions? The plasma membrane is a bilayer made of lipids with embedded protein molecules; the outer limit of the cytoplasm. It is responsible for the regulation of substances moving into and out of the cell. Downloaded by isabella denoon ([email protected]) lOMoARcPSD|47304420 2. When a cell gets bigger, does its surface area-to-volume ratio go up or down? When a cell gets bigger, the surface area-to-volume ratio gets smaller. This is because cells need to be able to get nutrients in and the waste out quickly, so if the cell is too big, it will not be able to function properly. 3. What is the difference between the word prokaryote and the term prokaryotic cell? The term prokaryotic cell refers to a particular cell architecture, that is, one lacking a nucleus, and not to a single group of organisms. Prokaryotes refers to any of the typically unicellular microorganisms that lack a distinct nucleus and membrane-bound organelles. 4. In what way is scanning electron microscopy different than transmission electron microscopy? In SEM, a beam of electrons is scanned over the surface of a specimen, resulting in a detailed 3D image of the specimen; used to look at intact specimens such as insects. In TEM, a beam of electrons is transmitted through an extremely thin section of a specimen, resulting a detailed image of the internal organization of cells and their organelles. Structure and organization of prokaryotic cells In almost all prokaryotic cells, the plasma membrane is surrounded by the cell wall, which provides rigidity to prokaryotic cells and protects them from physical damage. The cell wall is coated with an external layer of polysaccharides called the glycocalyx, which helps protect prokaryotic cells from physical damage and desiccation, and may enable a cell to attach to a surface such as other prokaryotic cells to form a colony. Prokaryotic cells have filamentous cytoskeletal structures with functions similar to those in eukaryotes. Prokaryotic cytoskeletons play important roles in creating and maintaining the proper shape of cells. Many bacteria and archaea use flagella to move through liquids and across wet surfaces. Some bacteria and archaea have hairlike shafts of protein called pili, which attaches the cell to surfaces or other cells. STUDY BREAK QUESTIONS 1. What is a nucleoid? A nucleoid is a region within the cell of a prokaryote that contains all or most of the genetic material. In contrast to the nucleus of a eukaryotic cell, it is not surrounded by a nuclear membrane. Eukaryotic cells have a nucleus and cytoplasmic organelles enclosed within a plasma membrane The cells of all eukaryotes have a membrane-bound compartment called the nucleus. The cytoplasm surrounding the nucleus contains a system of membranous organelles that you don’t find in prokaryotic cells. Each of these organelles is specialized to carry out one or more major functions of energy metabolism and molecular synthesis, storage, and transport. A supportive cell wall surrounds the plasma membrane of fungal, plant, and many protist cells. It is an extracellular structure. Animal cells do not have cell walls. The eukaryotic nucleus is more complex than the nucleoid of the prokaryotic cell The nucleus is separated from the cytoplasm by the nuclear envelope, which consists of two membranes, one layered just inside the other and separated by a narrow space. Downloaded by isabella denoon ([email protected]) lOMoARcPSD|47304420 Embedded in the nuclear envelope are nuclear pore complexes. A nuclear pore complex is a large, cylindrical structure formed of many types of proteins, called the nucleoporins. It exchanges molecules between the nucleus and the cytoplasm and prevents the transport of material not meant to cross the nuclear membrane. A nuclear pore is a channel through the nuclear pore complex, and it is the path for the assisted exchange of large molecules such as proteins and RNA molecules with the cytoplasm. Most of the space inside the nucleus is filled with chromatin, a combination of DNA and proteins. A eukaryotic nucleus also contains one or more nucleoli, which code for the rRNA molecules of ribosomes. Eukaryotic ribosomes are either free in the cytosol or attached to membranes Ribosomes consist of a large and small subunit. Some eukaryotic ribosomes are freely suspended in the cytosol; others are attached to membranes. Proteins that are made on free ribosomes in the cytosol may remain in the cytosol, or pass through the nuclear pores into the nuclear, or become parts of the mitochondria, chloroplasts, the cytoskeleton, or other cytoplasmic structures. Proteins that enter the nucleus become part of the chromatin, or line the nuclear envelope, or remain in solution in the nucleoplasm. An endomembrane system divides the cytoplasm into functional and structural components The endomembrane system is a collection of interrelated, internal, membranous sacs that divide the cell into functional and structural components. The endomembrane system has a number of functions, including the synthesis and modification of proteins and their transport into membranes and organelles or to the outside of the cell, the synthesis of lipids, and the detoxification of some toxins. The membranes of the system are connected either directly in the physical sense or indirectly by vesicles, which are small membrane bound compartments that transfer substances between parts o the system. Components of the endomembrane system: nuclear envelope, endoplasmic reticulum, golgi complex, lysosomes, vesicles and plasma membrane. The endoplasmic reticulum The ER is an extensive interconnected network of membranous channels and vesicles called cisternae. Each cisternae is formed by a single membrane that surrounds an enclosed space called the ER lumen. The rough ER is ER with ribosomes attached to it. The proteins made on ribosomes attached to the ER enter the ER lumen, where they fold into their final form. Chemical modifications of these proteins, such as the addition of carbohydrate groups (glycolysis) occur in the lumen. The proteins are then delivered to other regions of the cell within small vesicles that pinch off from Downloaded by isabella denoon ([email protected]) lOMoARcPSD|47304420 the ER, travel through the cytosol, and join with the organelle that performs the next steps in their modification and distributions. The golgi complex is the next destination, which packages and sorts them for delivery. The smooth ER is called that because its membranes have no ribosomes attached to their surfaces. It functions in the synthesis of lipids, the detoxification of drugs and poisons in your body, and the production of calcium for muscle contractions. The golgi complex The golgi complex receives proteins that were made in the ER and transported to the complex in vesicles. Has the cis face, which faces the nucleus, and the trans face, where vesicles leave for other cell locations. The golgi complex regulates the movement of several types of proteins. Some are secreted from the cell, others become imbedded in the plasma membranes as integral membrane proteins, and others are placed in lysosomes. Exocytosis: the process of capturing a substance or particle from outside the cell by engulfing it with the cell membrane and bringing it into the cell. Endocytosis: describes the process of vesicles fusing with the plasma membrane and releasing their contents to the outside of the cell. Lysosomes Found in animal cells but not in plant cells These are small membrane bound vesicles that contain more than 30 enzymes for the digestion of many complex molecules, including proteins, lipids, nucleic acids, and polysaccharides. Play a role in phagocytosis, a process in which some types of cells engulf bacteria or other cellular debris to break them down. Mitochondria are the organelles in which cellular respiration occurs Mitochondria are membrane bound organelles in which cellular respiration occurs. Supplies most of the cell’s ATP. Mitochondria are enclosed by two membranes: the outer membrane is smooth and covers the outside of the organelle. The surface area of the inner membrane is expanded by the folds called cristae. Both membranes surround the innermost compartment of the mitochondrion, called the mitochondrial matrix. The cytoskeleton supports and moves cell structures The cytoskeleton of animal cells contains structural elements of three major types: microtubules, intermediate filaments, and microfilaments. Each cytoskeletal element is assembled from proteins: microtubules from tubulins, intermediate filaments from intermediate proteins, and microfilaments from actins. Microtubules: plays a role in separating and moving chromosomes during cell division, determining the orientation for growth of the new cell wall during plant cell division, maintaining the shape of animal cells, and moving animal cells (flagella and cilia). Intermediate filaments: provide structural support in many cells and tissues. Downloaded by isabella denoon ([email protected]) lOMoARcPSD|47304420 Microfilaments: involved in the flowing motion of cytoplasm called cytoplasmic streaming, which can transport nutrients, proteins, and organelles in both animal and plant cells, and which is responsible for amoeboid movement. Also responsible in dividing the cytoplasm. Myosin’s: motor proteins that walk along microfilaments. Dynein’s and kinesins: motor proteins that walk along microtubules. Chloroplasts are biochemical factories powered by sunlight Chloroplasts are the sites of photosynthesis in plant cells. They are members of a family of plant organelles called plastids. Chloroplasts are surrounded by a smooth outer boundary membrane and an inner boundary membrane that lies just inside the outer membrane. These two boundary membranes completely enclose an inner compartment called stroma. Within the stroma are thylakoids and in higher plants, the thylakoids are stacked one on top of another, forming grana. The primary molecule that absorbs light is chlorophyll, a green pigment that is present in all chloroplasts. Responsible for photosynthesis. Central vacuoles have diverse roles in storage, structural support, and cell growth Central vacuoles are membrane bound organelles in plant cells that function in storage of water, enzymes, ions, proteins, pigments and toxins; produces support to plant cells. The membrane that surrounds the central vacuole is called the tonoplast, and it contains transport proteins that move substances into and out of the central vacuole. Animal cell junctions Cell adhesion molecules bind cells together and more complex cell junctions seal the spaces between cells and provide direct communication between cells. The extracellular matrix supports and protects cells and provides mechanical linkages. Anchoring junctions: form buttonlike spots, or belts, that run entirely around cells, “welding” adjacent cells together. For some anchoring junctions known as desmosomes, intermediate filaments anchor the junction in the underlying cytoplasm. In other anchoring junctions, known as adherens junctions, microfilaments are the anchoring cytoskeletal component. Tight junctions: regions of tight connections between membranes of adjacent cells. Seal the spaces between cells in the cell layers that cover internal organs and the outer surface of the body, or the layers that line internal cavities and ducts. Gap junctions: open direct channels that allow ions and small molecules to pass directly from one cell to another. The flow of ions and small molecules through the channels provides almost instantaneous communication between animal cells. Prokaryotic vs eukaryotic cells summary Eukaryotic Cell Prokaryotic Cell Example Animal, plants, fungi Bacteria, archaea Cell number Usually multicellular Usually unicellular Nucleus Present Absent Cell wall Only in plant cells and fungi Present in all Genetic recombination Meiosis and fusion of gametes DNA transfer between organisms Microtubules Present Absent Endoplasmic reticulum Present Absent Downloaded by isabella denoon ([email protected]) lOMoARcPSD|47304420 Cytoskeleton Extensive and complex Minimal Mitochondria Present Absent Ribosomes Present Present Vesicles Present Absent Golgi apparatus Present Absent Chloroplasts Present in plants Absent Vacuoles Present Present Flagella Complex Simple CELL CYCLES SUMMARY The cell cycle in prokaryotic organism Prokaryotic cells divide by a process called binary fission, where they split and divide into two parts, resulting in two identical daughter cells. Once daughter cells are formed, they may grow for some time (B period) before initiating DNA synthesis. Once the chromosomes are replicated and separated to opposite ends of the cell (C period), the membrane pinches together between them and two daughter cells are formed (D period). All bacteria and archaea use DNA are their hereditary information, and the vast majority of species package it all in a single, circular chromosome called the nucleoid. Replicated chromosomes are distributed actively to the daughter cells in binary fission Replication of the bacterial chromosomes begins at a specific region of DNA sequence called the origin of replication (ori). The ori region of the chromosome is in the middle of the cell, where the enzymes for DNA replication are located. Once the ori has been duplicated, the two new origins migrate toward the two opposite ends of the cell as replication continues for the rest of the chromosome. Chromosomes are the genetic units divided by mitosis In eukaryotes, the hereditary information of the nucleus is distributed among several linear, double stranded DNA molecules. These DNA molecules are combined with proteins that stabilize the DNA, assist in packaging DNA during cell division, and influence the expression of individual genes. Most eukaryotes have diploid (2n) chromosomes, meaning that they have two copies of each type of chromosome in their nuclei (eg. Humans have 23 chromosomes for a diploid number of 46 chromosomes; 2n=46). Other eukaryotes, mostly microorganisms, may have haploid (n) chromosomes, meaning that they have only one copy of each type of chromosome. Ploidy: the number of chromosome sets. Downloaded by isabella denoon ([email protected]) lOMoARcPSD|47304420 Before a cell divides in mitosis, replication of the DNA in a given chromosome produces two identical copies of that chromosome called sister chromatids. Newly formed sister chromatids are held together along their length by proteins called cohesins. Chromosome segregation: the equal distribution of daughter chromosomes into each of the two daughter cells that result from cell division. Interphase extends from the end of one mitosis to the beginning of the next mitosis Interphase consists of three phases of the cell cycle: 1. G1 phase: the cell carries out its function and in some cases, grows. 2. S phase: DNA replication and chromosome duplication occur. 3. G2 phase: a brief gap in the cell during which cell growth continues and the cell prepares for mitosis (M phase) and cytokinesis. G1 is also the stage in which many cell types stop dividing. Cells that are not destined to divide immediately enter the G0 phase. After interphase, mitosis proceeds in five stages 1. Prophase Replicated chromosomes condense Nuclear envelope disintegrates Spindle microtubules being to form 2. Prometaphase Spindle microtubules grow from centrosomes at the opposing spindle poles toward the center of the cell. 3. Metaphase Spindle apparatus is completely formed Spindle microtubules are attached to the centromere regions of the replicated chromosomes Replicated chromosomes line up at the mid-point of the spindle. 4. Anaphase Centromeres of each replicated chromosome separate Single chromosomes are pulled by their centromeres to opposite poles 5. Telophase Single chromosomes arrive at poles and begin to uncoil Nuclear envelope begins to form around each group of chromosomes Cytokinesis begins (cleavage furrow in animal cells, cell plate in plant cells Cytokinesis completes cell division by dividing the cytoplasm between daughter cells Cytokinesis is the division of the cytoplasm and usually follows the nuclear division stage of mitosis and produces two daughter cells. In animals, protists and many fungi, a furrow girdles the cell and gradually deepens until it cuts the cytoplasm into two parts. In plants, a new call wall, called the cell plate, forms between the daughter nuclei and grows until it divides the cytoplasm. Formation and action of the mitotic spindle Downloaded by isabella denoon ([email protected]) lOMoARcPSD|47304420 The mitotic spindle is made up of microtubules and their proteins. Microtubules from a major part of the interphase cytoskeleton of eukaryotic cells. As mitosis approaches, the microtubules disassemble from their interphase arrangement and reorganize into the spindle, which grows until it fills almost the entire cell. Animals and plants form spindles in different ways Centrosome: a site near the nucleus from which microtubules radiate outward in all directions. It is the main microtubule organizing centre (MTOC) of the cell. The centrosome contains a pair of centrioles, usually arranged at right angles to each other. The primary function of the centrioles is to generate the microtubules needed for flagella or cilia. When DNA replicates during the S phase of the cell cycle, the centrioles within the centrosome also duplicate, producing two new pairs. Mitotic spindles can move chromosomes by a combination of two mechanisms When fully formed at metaphase, the spindle may contain many microtubules, depending on the species. These microtubules can be divided into two groups: 1. Kinetochore microtubules: connect the chromosomes to the spindle poles. 2. Nonkinetochore microtubules: extend between the spindle poles without connecting to chromosomes. At one time, it was thought that microtubules pulled chromosomes toward the poles of the spindle. New information suggests that chromosomes, themselves, walk along kinetochore microtubules using motor proteins on the kinetochore. The tubulin subunits of the kinetochore microtubules are then disassembled behind the moving kinetochore. Thus, kinetochore microtubules become shorter as the chromosomes are moved towards the two poles of the spindle. Downloaded by isabella denoon ([email protected]) lOMoARcPSD|47304420 GENETIC RECOMBINATION SUMMARY Genetic recombination occurs in eukaryotes during meiosis Sexual reproduction depends on meiosis, a specialized process of cell division that recombines DNA sequences and produces cells with half the number of chromosomes present in the somatic (body cells) of a species. At fertilization, the nuclei of an egg and a sperm fuse, producing a cell called the zygote, which Is diploid. Both meiosis and fertilization also mix genetic information into new combinations, so none of the offspring are genetically identical to either parents. Meiosis changes both chromosome number and DNA sequence Meiosis is different to mitosis because meiosis has halved chromosome numbers and new combinations of alleles rising from recombined DNA sequences. Homologous chromosomes: pairs of chromosomes that have the same genes, are similar in size, and are arranged in the same order. Paternal chromosomes are derived from the male parent of the organism and maternal chromosomes are derived from the female parent. Meiosis produces four genetically different daughter cells This can occur during the process of synapsis, where homologous chromosomes pair up to form tetrads. During this pairing, recombination occurs, and chromosomal segments are exchanged. Genetic exchange occurs between homologous chromosomes during a process called crossing over. During the second meiotic division, these sister chromatids are separated into different cells, further reducing the amount of DNA in each product of meiosis. A total of four cells, each with the haploid number of chromosomes and a novel collection of alleles, is the final result of the two meiotic divisions. Random segregation of chromosomes and random fertilization also results in genetically different daughter cells. Stages of meiosis Meiosis I: halves the number of chromosomes (diploid -> haploid) 1. Prophase I Downloaded by isabella denoon ([email protected]) lOMoARcPSD|47304420 Replicated chromosomes condense, nuclear envelope disintegrates Spindle microtubules begin to form Homologous chromosomes pair up by synapsis to form tetrads Genetic exchange occurs between homologous chromosomes during a process called crossing over. 2. Metaphase I Spindle microtubules attach to the centromeres of each replicated chromosome Tetrads line up along the midregion of the cell 3. Anaphase I The homologous chromosomes separate from each other and are moved to opposite poles of the cell by the spindle apparatus 4. Telophase I Replicated chromosomes arrive at poles and begin to uncoil Spindle apparatus disappears and nuclear envelope starts to re-form Cytokinesis begins At the end of telophase I, each daughter cell contains the haploid number of replicated chromosomes (n = 2) Meiosis II: separates replicated chromosomes into single chromosomes. 5. Prophase II Replicated chromosomes condense again and nuclear envelope disintegrates again New spindle apparatus forms 6. Metaphase II Spindle microtubules attach to the centromeres of each replicated chromosome Replicated chromosomes line up individually along the metaphase plate of each of the daughter cells 7. Anaphase II Centromeres of each replicated chromosome separate and the resulting single chromosomes are moved to opposite poles by the spindle apparatus 8. Telophase II Single chromosomes arrive at opposite poles and start to uncoil Spindle apparatus disappears and nuclear envelope will re-form Nucleoli will become visible Cytokinesis begins At the end of telophase II, there will be four daughter cells, each containing the haploid number of single chromosomes (n = 2) Summary of differences Event or feature Meiosis Mitosis Cell divisions Two; occurs only in diploid cells One; occurs in both haploid and diploid cells Synapsis of homologous Yes No chromosomes Crossing over Yes No Number of daughter cells 4; genetically different 2; genetically identical Role in life cycle Halving chromosome number in Cell division for growth, asexual Downloaded by isabella denoon ([email protected]) lOMoARcPSD|47304420 animal cells that produce gametes, reproduction in some eukaryotes, in plant cells that produce spores, replacement of old or damaged and in fungi and algae to produce tissues spores DEFINING LIFE AND ITS ORIGINS SUMMARY Seven characteristics shared by all lifeforms: Display order: all forms of life are arranged in a highly organized manner, with the cell being the fundamental unit that exhibits all properties of life. Harness and utilize energy: all forms of life acquire energy from the environment and use it to maintain their highly ordered state. Reproduce: all organisms have the ability to make more of their own kind. Respond to stimuli: organisms can make adjustments to their structure, function, and behaviour in response to changes to the external environment. Exhibit homeostasis: organisms are able to regulate their internal environment such that conditions remain relatively constant (Eg. Sweating). Grow and develop: all organisms increase their size by increasing the size and/or number of cells. Many organisms also change over time. Evolve: populations of living organisms change over the course of generations to become better adapted to their environment. The characteristics of life are emergent The seven properties of life are a result of emergent properties because they come about, or emerge, from many simpler interactions that, in themselves, do not have the properties found at the higher levels. In other words, an emergent property is new and cannot be broken down into or analyzed solely in terms of its component parts (Eg. The liver). Hypotheses of the origins of life 1. Reducing atmosphere hypothesis The primordial atmosphere of earth probably contained an abundance of water vapour from the evaporation of water at the surface, as well as hydrogen, carbon dioxide, ammonia, and methane. There was almost a complete absence of oxygen. Aleksandr Oparin and John Haldane proposed that organic molecules could have formed in the atmosphere of primordial Earth. These molecules of hydrogen, methane and ammonia contain an abundance of electrons and hydrogen, and they would have entered into reactions with one another and would have yielded larger and more complex organic molecules. Downloaded by isabella denoon ([email protected]) lOMoARcPSD|47304420 In comparison to today’s atmosphere, the prescience of high levels of oxygen prevents complex, electron-rich molecules from being formed because oxygen is a strong oxidizing molecule and would itself accept the electrons from organic molecules and be reduced to water. This hypothesis was tested by the Miller-Urey experiment: using an apparatus, Stanley Miller demonstrated that organic molecules can be synthesized and under conditions stimulating primordial Earth. 2. Deep-sea vents hypothesis Gunter Wachtershauser proposed that the complex organic molecules necessary for life could have originated on the ocean floor at the side of deep sea (hydrothermal) vents. He argues that the early chemistry necessary for the formation of organic molecules of life did not occur in bulk solutions in the surface oceans, but on mineral surfaces within recently discovered vents. There are two kinds of hydrothermal vents that have been identified: black smokers and white smoker. 3. Extraterrestrial origins hypothesis It is possible that the key organic molecules required for life to begin came from space. Some scientists have hypothesized that the Earth was salted with an abundance of these organic molecules from carbonaceous chondrite meteorites during the origin of the primordial earth. Analysis of the Murchison meteorite showed that it contains an assortment of biologically important molecules, including a range of amino acids such as glycine, glutamic acid, and alanine, as well as purines and pyrimidines. Life requires the synthesis of polymers (clay hypothesis) It is likely that solid surfaces, especially clays, could have provided the type of environment necessary for polymerization to occur. Clays consist of very thin minerals separated by layers of water only a few nanometers thick. The layered structure of clay is also charged, allowing for molecular adhesion forces to bring monomers together in precise orientations that could more readily lead to polymer formation. Clay can also store the potential energy that may have been used for energy-requiring polymerization reactions. This clay hypothesis demonstrates that the formation of short nucleic acid chains and polypeptides can be synthesized on a clay surface. Lipid spheres may have led to the development of cells Protobiont: term given to a group of abiotically produced organic molecules that are surrounded by a membrane or membranelike structure. Protobionts could have formed spontaneously, given the conditions on primordial Earth. An early type of protobiont could have been similar to a liposome, which is a lipid vesicle in which the lipid molecules form a bilayer very similar to a cell membrane. Liposomes can easily be made in the laboratory and are selectively permeable, allowing only some molecules to move in and out. Downloaded by isabella denoon ([email protected]) lOMoARcPSD|47304420 The presence of clay not only catalyzes the polymerization of nucleic acids but also accelerates the formation of lipid vesicles. As well, clay particles often become encapsulated in these vesicles, which would provide catalytically active surfaces within membrane vesicles upon which key reactions could take place. RNA can carry information and catalyze reactions DNA is the molecule that provides every cell with the genetic instructions necessary to function. Central dogma: the flow of information from DNA to RNA to protein. Each step of the information flow requires the involvement of a group of proteins called enzymes, which catalyze the transcription of DNA into RNA and the translation of the RNA into protein. Ribozymes: a group of RNA catalysts that can catalyze reactions on the precursor RNA molecules that lead to their own synthesis, as well as on unrelated DNA molecules. Ribozyme’s have catalytic properties because these single stranded molecules can fold into very specific shapes based on intramolecular hydrogen bonding or base pairing. The discovery of ribozymes revolutionized thinking about the origin of life. Early life may have existed in an “RNA world,” where a single type of molecule, RNA, could serve as a carrier or information and a structural/functional molecule similar to a protein. RNA is replaced by DNA for information storage and proteins for catalysis RNA was replaced by DNA because DNA does a better job of information storage and catalysis. At some point, the DNA nucleotides paired with the RNA informational molecules and were assembled into complementary copies of the RNA sequences. Once the DNA copies were made, selection may have favoured DNA, as it is a much better way to store information than RNA, for three main reasons: 1. Each strand of DNA is chemically more stable than RNA. 2. The base uracil found in RNA is not found in DNA. 3. DNA is double stranded, so in the case of a mutation to one of the strands, the information contained on the complementary strand can be used to correctly repair the damaged strand. The first cells were probably anaerobic heterotrophs The earliest forms of life were most likely heterotrophs, organisms that obtain carbon from organic molecules. The earliest forms of life were most certainly anaerobic because there is no requirement for membrane bound complexes, which is necessary for electron transport chains, and there is no requirement for O2, which was found in only trace amounts in the early atmosphere. Compared to heterotrophs, autotrophs obtain carbon from the environment in an inorganic form, most often carbon dioxide. Plants and other photosynthetic organisms are the dominant autotrophs today and are classified as photoautotrophs because they use light as an energy source. Downloaded by isabella denoon ([email protected]) lOMoARcPSD|47304420 Oxygenic photosynthesis led to a rise in oxygen in the atmosphere Starting about 2.5 billion years ago, oxygen levels in the atmosphere began increasing. Evidence for this comes from dating a type of sedimentary rock called banded iron. Geologists believe that these distinctive striped rocks were formed in the sediments of lakes and oceans when dissolved oxygen reacted with the iron in the water, forming a red-coloured precipitate, iron oxide (rust), which ended up being incorporated into the resulting sedimentary rock formations. The oxygen had a biological source, it was thought to have been derived from a group of photosynthetic prokaryotic cells called cyanobacteria. Cyanobacteria had the ability to extract electrons from a molecule that was much more abundant: water. Oxygenic photosynthesis: photosynthesis that relies on the oxidation of water as the source of electrons. The theory of endosymbiosis suggests that mitochondria and chloroplasts evolved from ingested prokaryotic cells Evidence indicates that mitochondria and chloroplasts are descended from free-living prokaryotic cells. Mitochondria are descended from aerobic bacteria, and chloroplasts are descended from cyanobacteria. The model of endosymbiosis states that the prokaryotic ancestors of modern mitochondria and chloroplasts were engulfed by larger prokaryotic cells, forming a mutually advantageous relationship called a symbiosis. Slowly, over time, the host cell and the endosymbionts became inseparable parts of the same single-celled organism. 6 lines of evidence suggest that both chloroplasts and mitochondria have distinctly prokaryotic characteristics that are not found in other eukaryotic organelles: 1. Morphology: the shape and size of both mitochondria and chloroplasts are similar to those of prokaryotic cells. 2. Reproduction: a cell cannot synthesize a mitochondrion or a chloroplast. Just like free- living prokaryotic cells, where daughter cells arise by cell division, new mitochondria and chloroplasts are derived from the division of pre-existing organelles. Both chloroplast and mitochondria divide by binary fission, which is how prokaryotic cells divide. 3. Genetic information: both mitochondria and chloroplasts contain their own DNA, which contain both protein-coding and non-coding genes that are essential for organelle function. As with prokaryotic cells, the DNA molecule in most mitochondria and chloroplasts is circular, while the DNA molecules in the nucleus are linear. 4. Transcription and translation: both chloroplasts and mitochondria contain a complete transcription and translational machinery. They contain ribosomes and tRNA’s that are necessary to translate organelle mRNAs into proteins. 5. Electron transport: similar to free-living prokaryotic cells, both mitochondria and chloroplasts have electron transport chains and the enzyme ATP synthase, which together are used to generate chemical energy. Downloaded by isabella denoon ([email protected]) lOMoARcPSD|47304420 6. Sequence analysis: the sequence of chloroplast rRNA most closely matches that of cyanobacteria, and the sequence of mitochondrial rRNA is most similar to that of heterotrophic bacteria. It is suggested that endosymbiosis occurred in stages, with mitochondria occurring first and then chloroplasts. The endomembrane system may be derived from the plasma membrane The hypothesis is that the endomembrane system is derived from the infolding of the plasma membrane. In cell lines leading from prokaryotic cells to eukaryotes, pockets of the plasma membrane may have extended inward and surrounded the nuclear region. Some of these membranes fused around the DNA, forming the nuclear envelope, which defines the nucleus. The remaining membranes formed vesicles in the cytoplasm and gave rise to the ER and the golgi complex. Evolution of multicellular eukaryotes The increase in energy supplied to eukaryotic cells by the presence of numerous mitochondria per call has permitted an: 1. Overall increase in body size through multicellularity and increased levels of activity. 2. Increase in size and completely of individual cells 3. Increased size of the genome. More genes = more diversity (the average eukaryotic cell has 25 times more DNA than a prokaryotic cell. 4. The development of tissues and organs that increased the efficiency of managing body functions in eukaryotes. Multi-celled organisms could have evolved if the dividing cell of once unicellular organisms began to remain together rather than separating after mitosis. Could also happen if individual cells of the same species aggregated together to form a multicellular organism. First multi celled eukaryotes were likely colonial, with all the cells being structurally and functionally similar. Since all cells in a colony are similar, often, one cell released from a colony could survive on its own and divide by mitosis to produce a new colony. Over time, cells within the organism made up of more than one cell began to specialize their functions such that individual cells could no longer live on their own. These organisms would be truly multicellular. Downloaded by isabella denoon ([email protected])