BI101 Exam #3 Notes Lectures 8, 9, 10 PDF
Document Details
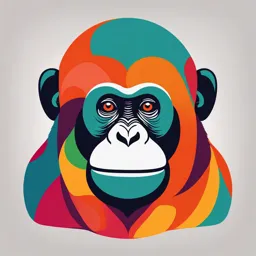
Uploaded by ExcitedAgate1096
Arcadia University
Tags
Summary
These notes cover lectures 8, 9, and 10 for BI101 and detail topics including membrane structure and function, discussing phospholipids, proteins, and transport mechanisms. The material also introduces metabolic pathways, differentiating between catabolic and anabolic processes, with examples. The lectures explore energy transformations and principles of thermodynamics.
Full Transcript
Lecture 8 CH 7 10/3/24 Quiz 4 - Tuesday Oct 8 - membrane Quiz 5 - Oct 10 - metabolism Review - Oct 17 Photosynthesis - Oct 22 No class on Thursday Oct 24 Exam 3 - Tuesday Oct 29 Membrane Structure and Function Life at the Edge - The plasma membrane is the boundar...
Lecture 8 CH 7 10/3/24 Quiz 4 - Tuesday Oct 8 - membrane Quiz 5 - Oct 10 - metabolism Review - Oct 17 Photosynthesis - Oct 22 No class on Thursday Oct 24 Exam 3 - Tuesday Oct 29 Membrane Structure and Function Life at the Edge - The plasma membrane is the boundary that separates the living cell from its surroundings - The plasma membrane exhibits selective permeability, allowing some substances to cross it more easily than others Cellular membranes are fluid mosaics of lipids and proteins - Phospholipids are the most abundant lipid in the plasma membrane - Phospholipids are amphipathic molecules, containing hydrophobic and hydrophilic regions - A phospholipid bilayer can exist as a stable boundary between two aqueous compartments - The fluid mosaic model states that a membrane is a fluid structure with a “mosaic” of various proteins embedded in it - Proteins are not randomly distributed in the membrane \\ Membrane Proteins and Their Functions - A membrane is a collage of different proteins, often grouped together, embedded in the fluid matrix of the lipid bilayer - Proteins determine most of the membrane’s specific functions - Peripheral proteins are bound to the surface of the membrane - Integral proteins penetrate the hydrophobic core - Integral proteins that span the membrane are called transmembrane proteins - Six major functions of membrane proteins - Transport - Enzymatic activity - Signal transduction - Six major functions of membrane proteins - Cell-cell recognition - Intercellular joining - Attachment to the cytoskeleton and extracellular matrix (ECM) - HIV must bind to the immune cell surface protein CD4 and a “co-receptor” CCR5 in order to infect a cell - HIV cannot enter the cells of resistant individuals that lack CCR5 The Role of Membrane Carbohydrates in Cell-Cell Recognition - Cells recognize each other by binding to molecules, often containing carbohydrates, on the extracellular surface of the plasma membrane - Membrane carbohydrates may be covalently bonded to lipids (forming glycolipids) or more commonly to proteins (forming glycoproteins) Membrane structure results in selective permeability - A cell must exchange materials with its surroundings, a process controlled by the plasma membrane - Plasma membranes are selectively permeable, regulation the cell’s molecular traffic The Permeability of the Lipid Bilayer - Hydrophobic (nonpolar) molecules, such as hydrocarbons, can dissolve in the lipid bilayer and pass through the membrane rapidly - Hydrophilic molecules including ions and polar molecules do not cross the membrane easily Transport Proteins - Transport proteins allow passage of hydrophilic substances across the membrane - Some transport proteins, called channel proteins, have a hydrophilic channel that certain molecules or ions can use as a tunnel - Channel proteins called aquaporins facilitate the passage of water - Other transport proteins, called carrier proteins, bind to molecules and change shape to shuttle them across the membrane - A transport protein is specific for the substance it moves Passive transport is diffusion of a substance across a membrane with no energy investment - Diffusion is the tendency for molecules to spread out evenly into the available space - Although each molecule moves randomly, diffusion of a population of molecules may be directional - At dynamic equilibrium, as many molecules cross the membrane in one direction as in the other - Substances diffuse down their concentration gradient, the region along which the density of a chemical substance increases or decreases - No work must be done to move substances down the concentration gradient - The diffusion of a substance across a biological membrane is passive transport because no energy is expended by the cell to make it happen Effects of Osmosis on Water Balance - Osmosis is the diffusion of water across a selectively permeable membrane - Water diffuses across a membrane from the region of lower solute concentration to the region of higher solute concentration until the solute concentration is equal on both sides Water Balance of Cells Without Cell Walls Tonicity is the ability of a surrounding solution to cause a cell to gain or lose water Isotonic solution: solute concentration is the same as that inside the cell: no net water movement across the plasma membrane Hypertonic solution: solute concentration is greater than that inside the cell; cell loses water Hypotonic solution: solute concentration is less than that inside the cell; cell gains water - Hypertonic or hypotonic environments create problems for organisms - Osmoregulation, the control of solute concentrations and water balance, is a necessary adaptation for life in such environments Water Balance of Cells with Cell Walls - Cell walls help maintain water balance - A plant cell in a hypotonic solution swells until the wall opposes uptake; the is now turgid (frim) - If a plant cell and its surroundings are isotonic, there is no net movement of water into the cell; the cell becomes flaccid (limp) - In a hypertonic environment, plant cells lose water - The membrane pulls away from the cell wall causing the plant to wilt, a usually lethal effect called plasmolysis Facilitated Diffusion: Passive Transport Aided by proteins - In facilitated diffusion, transport proteins speed the passive movement of molecules across the plasma membrane - Transport proteins include channel proteins and carrier protein - Channel proteins provide corridors that a specific molecule or ion cross the membrane - Aquaporins facilitate the diffusion of water - Ion channels facilitate the diffusion of ions - Some ion channels, called gated channels, open or close in response to a stimulus - Carrier proteins undergo a subtle change in shape that translocates the solute-binding site across the membrane The Need for Energy in Active Transport - Active transport moves substances against their concentration gradients - Active transport requires energy, usually in the form of ATP - Active transport is performed by specific proteins embedded in the membranes - Active transport allows cells to maintain concentration gradients that differ from their surroundings - The sodium-potassium pump is one type of active transport system \ Active Transport Membrane Transport Cotransport: Coupled Transport by a Membrane Protein - Cotransport occurs when active transport of a solute indirectly drives transport of other substances - Plants commonly use the gradient of hydrogen ions generated by proton pumps to drive active transport of nutrients into the cell Bulk transport across the plasma membrane occurs by exocytosis and endocytosis - Small molecules and water enter or leave the cell through the lipid bilayer or via transport proteins - Large molecules, such as polysaccharides and proteins, cross the membrane in bulk via vesicles - Bulk transport requires energy Exocytosis - In exocytosis, transport vesicles migrate to the membrane, fuse with it, and release their contents outside the cell - Many secretory cells use exocytosis to export their products Endocytosis - In endocytosis, the cell takes in macromolecules by forming vesicles from the plasma membrane - Endocytosis is a reversal of exocytosis, involving different proteins - There are three types of endocytosis - Phagocytosis (“cellular eating”) - Pinocytosis (“cellular drinking”) - receptor -mediated endocytosis”) - receptor -mediated endocytosis - In phagocytosis a cell engulfs a particle in a vacuole - The vacuole fuses with a lysosome to digest the particle - In pinocytosis, molecules dissolved in droplets are taken up when extracellular fluid is “gulped” into tiny vesicles - In receptor-mediated endocytosis, binding of ligands to receptors triggers vesicle formation - A ligand is any molecule that binds specifically to a receptor site of another molecule Lecture 9 CH 8 10/7/24 An Introduction to Metabolism The Energy of Life - The living cell is a miniature chemical factory where thousands of reactions occur - The cell extracts energy stored in sugars and other fuels and applies energy to perform work - Some organisms even convert energy to light, as in bioluminescence An organism’s metabolism transforms matter and energy, subject to the laws of thermodynamics - Metabolism is the totality of an organism’s chemical reactions - Metabolism is an emergent property of life that arises from orderly interaction between molecules Organization of the Chemistry of Life into Metabolic Pathways - A metabolic pathway begins with a specific molecule and ends with a product - Each step is catalyzed by a specific enzyme - Major metabolic pathways - Catabolic pathway - Anabolic pathway - Catabolic pathways release energy by breaking down complex molecules into simpler compounds - Cellular respiration, the breakdown of glucose in the presence of oxygen, is an example of a pathway of catabolism - Anabolic pathways consume energy to build complex molecules from simpler ones - The synthesis of protein from amino acids is an example of anabolism - Bioenergetics is the study of how energy flows through living organisms Forms of Energy - Energy is the capacity to cause change - Potential energy is the energy that matter possesses because of its location or structure - Chemical energy is potential energy available for release in a chemical reaction - Kinetic energy is the energy associated with motion - Heat (thermal energy) is kinetic energy associated with random movement of atoms or molecules - Energy can be converted from one form to another Energy Concepts The Laws of Energy Transformation - Thermodynamics is the study of energy transformations - An isolated system, such as that liquid in a thermos, is unable to exchange energy or matter with its surroundings - In an open system, energy and matter can be transferred between the system and its surroundings - Organisms are open systems The First Law of Thermodynamics - According to the first law of thermodynamics, the energy of the universe is constant - Energy can be transferred and transformed, but it cannot be created or destroyed - The first law is also called the principle of conservation of energy The Second Law of Thermodynamics - During every energy transfer or transformation, some energy is unusable, and is often lost as heat - According to the second law of thermodynamics - Every energy transfer or transformation increases the entropy (disorder) of the universe - Spontaneous processes occur without energy input; they can happen quickly or slowly The free-energy change of a reaction tells us whether or not the reaction occurs spontaneously - Biologists want to know which reaction occur spontaneously and which require input of energy - To do so, they need to determine energy changes that occur in chemical reactions Free-energy Change, ΔG - A living system’s free energy is energy that can do work when temperature and pressure are uniform as in a living cell - The change in free energy (ΔG) during a process is related to the change in enthalpy, or change in total energy (ΔH), change in entropy (ΔS), and temperature in Kelvin units (T) ΔG = ΔH - TΔS Free Energy, Stability, and Equilibrium - Free energy us a measure of a system’s instability, its tendency to change to a more stable state ΔG (Gibbs Free Energy) Free Energy and Metabolism - The concept of free energy can be applied to the chemistry of life’s processes Exergonic and Endergonic Reactions in Metabolism - An exergonic reaction proceeds with a net release of free energy and is spontaneous - An endergonic reaction absorbs free energy from its surroundings and is nonspontaneous Equilibrium and Metabolism - Reactions in a closed system eventually reach equilibrium and then do no work - Cells are not in equilibrium; they are open systems experiencing a constant flow of materials - A defining feature of life is that metabolism is never at equilibrium - A catabolic pathway in a cell releases free energy in a series of reactions ATP powers cellular work by coupling exergonic reactions to endergonic reactions - A cell does three main kinds of work - Chemical - Transport - - Mechanical - To do work, cells manage energy resources by energy coupling, the use of an exergonic process to drive an endergonic one - Most energy coupling in cells is mediated by ATP The Structure and Hydrolysis of ATP - ATP (adenosine triphosphate) is the cell’s energy shuttle - ATP is composed of ribose (a sugar), adenine (a nitrogenous base), and three phosphate groups - The bonds between the phosphate groups of ATP’s tail can be broken by hydrolysis - Energy is released from ATP when the terminal phosphate bond is broken How the Hydrolysis of ATP Performs Work - The three types of cellular work (mechanical, transport, and chemical) are powered by the hydrolysis of ATP - In the cell, the energy from the exergonic reaction of ATP hydrolysis can be used to drive an endergonic reaction - Transport and mechanical work in the cell are c\also powered by ATP hydrolysis - ATP hydrolysis leads to a change in protein shape and binding ability The Regeneration of ATP - ATP is a renewable resource that is regenerated by addition of a phosphate group to adenosine diphosphate (ADP) - The energy to phosphorylate ADP comes from catabolic reactions in the cell - The ATP cycle is a revolving door through which energy passes during its transfer from catabolic to anabolic pathways Enzymes speed up metabolic reactions by lowering energy barriers - A catalyst is a chemical agent that speeds up a reaction without being consumed by the reaction - An enzyme is a catalytic protein - Hydrolysis of sucrose by the enzyme sucrase is an example of an enzyme-catalyzed reaction The Activation Energy Barrier - Every chemical reaction between molecules involves bond breaking and bond forming - The initial energy needed to start a chemical reaction is called the free energy of activation, or activation energy (EA). How Enzymes Work How Enzymes Speed Up Reactions - Enzymes catalyze reactions by lowering the EA barrier - Enzymes do not affect the change in free energy (ΔG); instead, they speed up reactions that would occur eventually Substrate Specificity of Enzymes - The reactant that an enzyme acts on is called the enzyme’s substrate - The enzyme binds to its substrate, forming an enzyme-substrate complex - The reaction catalyzed by each enzyme is very specific - The active site is the region on the enzyme where the substrate binds Effects of Local Condition on Enzyme Activity - An enzyme's activity can be affected by - General environmental factors, such as temperature and pH - Chemicals that specifically influence the enzyme Effects of Temperature and pH - Each enzyme has an optimal temperature in which it can function - Each enzyme has an optimal pH in which it can function - Optimal conditions favor the most active shape for the enzyme molecule Cofactors - Cofactors are nonprotein enzyme helpers - Cofactors may be inorganic (such as a metal in ionic form) or organic - An organic cofactor is called a coenzyme - Coenzymes include vitamins Enzyme Inhibitors - Competitive inhibitors bind to the active site of an enzyme, competing with the substrate - Noncompetitive inhibitors bind to another part of an enzyme, causing the enzyme to change shape and making the active site less effecting - Examples of inhibitors include toxins, poisons, pesticides, and antibiotics The Evolution of Enzymes - Enzymes are proteins encoded by genes - Changes (mutations) in genes lead to changes in amino acid composition of an enzyme - Altered amino acids in enzymes may result in novel enzyme activity or altered substrate specificity - Under new environmental conditions a novel form of an ez]enzyme of an enzyme might be favored - For example, six amino acid changes improved substrate binding and breakdown in E. coli Regulation of enzyme activity helios control metabolism - Chemical chaos would result if a cell’s metabolic pathways were not tightly regulated - A cell does this by switching on or off the genes that encode specific enzymes or by regulating the activity enzymes Feedback Inhibition - In feedback inhibition, the end product of a metabolic pathway shuts down the pathways - Feedback inhibition prevents a cell from wasting chemical resources by synthesizing more product than is needed Localization of Enzymes Within the Cell - Some enzymes act as structural components of membranes - In eukaryotic cells, some enzymes reside in specific organelles; for example, enzymes for cellular respiration are located in mitochondria Lecture 10 CH 9 10/10/24 Cellular Respiration and Fermentation Life Is Work - Living cells require energy from outside sources - Some animals, such as the giraffe, obtain energy by eating plants, and some animals feed on other organisms that eat plants - Energy flows into an ecosystem as sunlight and leaves as heat - Photosynthesis generates O2 and organic molecules, which are used in cellular respiration - Cells use chemical energy stored in organic molecules to generate ATP, which powers work The Carbon Cycle Catabolic pathways yield energy by oxidizing organic fuels - Catabolic pathways release stored energy by breaking down complex molecules - Electron transfer plays a major role in these pathways - These processes are central to cellular respiration Catabolic Pathways and Production of ATP - The breakdown of organic molecules is exergonic - Fermentation is a partial degradation of sugars that occurs without O2 - Aerobic respiration consumes organic molecules and O2 and yields ATP - Cellular respiration includes both aerobic and anaerobic respiration but is often used to refer to aerobic respiration - Although carbohydrates, fats, and proteins are all consumed as fuel, it is helpful to trace cellular respiration with the sugar glucose Redox Reactions: Oxidation and Reduction - The transfer of electrons during chemical reactions releases energy stored in organic molecules - This released energy is ultimately used to synthesize ATP The Principle of Redox - Chemical reactions that transfer electrons between reactants are called oxidation-reduction reactions, or redox reactions The Principle of Redox - In oxidation, a substance loses electrons, or is oxidized - In reduction, a substance gains electrons, or is reduced - The electron donor is called the reducing agent - The electron acceptor is called the oxidizing agent - An example is the reaction between methane and O2 Oxidation of Organic Fuel Molecules During Cellular Respiration - During cellular respiration, the fuel (such as glucose) is oxidized, and O2 is reduced Stepwise Energy Harvest via NAD+ and the Electron Transport Chain - In cellular respiration, glucose and other organic molecules are broken down in a series of steps - Electrons from organic compounds are usually first transferred to NAD+, a coenzyme - As an electron acceptor, NAD+ functions as an oxidizing agent during cellular respiration - Each NADH (the reduced form of NAD+) represents stored energy that is tapped to synthesize ATP - NADH passes the electrons to the electron transport chain - Unlike an uncr=ontrolled reaction, the electron transport chain passes electrons in a series of steps instead of one explosive reaction - O2 pulls electrons down the chain in an energy-yielding tumble - The energy yielded is used to regenerate ATP The Stages of Cellular Respiration: A Preview - Harvesting of energy from glucose has three stages - Glycolysis (breaks down glucose into two molecules of pyruvate) - The citric acid cycle (completes the breakdown of glucose) - Oxidative phosphorylation (accounts for most of the ATP synthesis) - The process that generates most of the ATP is called oxidative phosphorylation because it is powered by redox reactions - Oxidative phosphorylation accounts for almost 90% if the ATP generated by cellular respiration - A smaller amount of ATP is formed in glycolysis and the citric acid cycle by substrate-level phosphorylation - For each molecule of glucose degraded to CO2 and water by respiration, the cell makes up to 32 molecules of ATP Glycolysis harvests chemical energy by oxidizing glucose to pyruvate - Glycolysis (“sugar splitting”) breaks down glucose into two molecules of pyruvate - Glycolysis occurs in the cytoplasm and has two major phases - Energy investment phase - Energy payoff phase - Glycolysis occurs whether or not O2 is present After pyruvate is oxidized, the citric acid cycle completes the energy-yielding oxidation of organic molecules - In the presence of O2, pyruvate enters the mitochondrion (in eukaryotic cells) where the oxidation of glucose is completed Oxidation of Pyruvate to Acetyl CoA - Before the citric acid cycle can begin, pyruvate must be converted to acetyl Coenzyme A (acetyl CoA), which links glycolysis to the citric acid cycle - This step is carried out by a multienzyme complex that catalyzes three reactions The Citric Acid Cycle - The citric acid cycle, also called the Krebs cycle, completes the breakdown of pyruvate to CO2 - The cycle oxidizes organic fuel derived from pyruvate, generating 1 ATP, 3 NADH, and 1 FADH2 per turn - The citric acid cycle has eight steps, each catalyzed by a specific enzyme - The acetyl group of acetyl CoA joins the cycle by combining with oxaloacetate, forming citrate - The next seven steps decompose the citrate back to oxaloacetate, making the process a cycle During oxidative phosphorylation, chemiosmosis couples electron transport to ATP synthesis - Following glycolysis and the citric acid cycle, NDAH and FADH2 account for most of the energy extracted from food - These two electron carriers donate electrons to the electron transport chain, which powers ATP synthesis via oxidative phosphorylation The Pathway of Electron Transport - The electron transport chain is in the inner membrane (cristae) of the mitochondrion - Most of the chain’s components are proteins, which exist in multiprotein complexes - Electrons drop in free energy as they go down the chain and are finally passed to O2, forming H2O - Electrons are transferred from NADH or FADH2 to the electron transport chain - Electrons are passed through a number of proteins including cytochromes (each with an iron atom) to O2 - The electron transport chain generates no ATP directly - It breaks the large free-energy drop from food to O2 into smaller steps that release energy in manageable amounts Chemiosmosis: The Energy-Coupling Mechanism - Electron transfer in the electron transport chain causes proteins to pump H+ from the mitochondrial matrix to the intermembrane space - H+ then moves back across the membrane, passing through the protein complex, ATP synthase - ATP synthase uses the exergonic flow of H+ to drive phosphorylation of ATP - This is an example of chemiosmosis, the use of energy in a H+ gradient to drive cellular work An Accounting of ATP Production by Cellular Respiration - During cellular respiration, most energy flows in this sequence: Glucose –-> NADH —> electron transport chain —> proton-motive force —> ATP - About 34% of the energy in a glucose molecule is transferred to ATP during cellular respiration, making aout 32 ATP Fermentation and anaerobic respiration enable cells to produce ATP without the use of oxygen - Most cellular respiration requires O2 to produce ATP - Without O2, the electron transport chain will cease to operate - In that case, glycolysis couples with anaerobic respiration or fermentation to produce ATP Types of Fermentation - Fermentation consists of glycolysis plus reactions that regenerate NAD+, which can be reused by glycolysis - Two common types are alcohol fermentation and lactic acid fermentation - In alcohol fermentation, pyruvate is converted to ethanol in two steps - The first step releases CO2 - The second step produces ethanol - Alcohol fermentation by yeast is used in brewing, winemaking, and baking - In lactic acid fermentation, pyruvate is reduced by NADH, forming lactate as an end product, with no release of CO2 - Lactic acid fermentation by some fungi and bacteria is used to make cheese and yogurt - Human muscle cells use lactic acid fermentation to generate ATP when O2 is scarce Comparing Fermentation with Anaerobic and Aerobic Respiration - All use glycolysis (net ATP = 2) to oxidize glucose and harvest chemical energy of food - In all, Nad+ is the oxidizing agent that accepts electrons during glycolysis - The processes have different mechanisms for oxidizing NADH: - In fermentation, an organic molecule (such as pyruvate or acetaldehyde) acts as a final electron acceptor - In cellular respiration electrons are transferred to the electron transp[ort chain - Cellular respiration produces 32 ATP per glucose molecule; fermentation produces 2 ATP per glucose molecule - Obligate anaerobes carry out fermentation or anaerobic respiration and cannot survive in the presence of O2 - Yeast and many bacteria are facultative anaerobes, meaning that they can survive using either fermentation or cellular respiration - In a facultative anaerobe, pyruvate is a fork in the metabolic road that leads to two alternative catabolic routes The Evolutionary Significance of Glycolysis - Ancient prokaryotes are thought to have used glycolysis long before there was oxygen in the atmosphere - Very little O2 was available in the atmosphere until about 2.7 billion years ago, so early prokaryotes likely used only glycolysis to generate ATP - Glycolysis is a very ancient process Glycolysis and the citric acid cycle connect to many other metabolic pathways - Glycolysis and the citric acid cycle are major intersections to various catabolic and anabolic pathways The Versatility of Catabolism - Catabolic pathways funnel electrons from many kinds of organic molecules into cellular respiration - Glycolysis accepts a wide range of carbohydrates - Proteins must be digested to amino acids; amino groups can feed glycolysis or the citric acid cycle - Fats are digested to glycerol (used in glycolysis) and fatty acids (used in generating acetyl CoA) - Fatty acids are broken down by beta oxidation and yield acetyl CoA - An oxidized gram of fat produces more than twice as much ATP as an oxidized gram of carbohydrate Biosynthesis (Anabolic Pathways) - The body uses small molecules to build other substances - These small molecules may come directly from food, from glycolysis, or from the citric acid cycle Regulation of Cellular Respiration via Feedback Mechanisms - Feedback inhibition is the most common mechanism for metabolic control - If ATP concentration begins to drop, respiration speeds up; when there is plenty of AATP, respiration slows down - Control of catabolism is based mainly on regulating the activity of enzymes at strategic points in the catabolic pathway Know what delta g and all of them mean Know exergonic and endergonic Oxidation and reduction and relate it to everything Two different types of electron carrier NAD and FAD Types of fermentation Exam bulk is on the second two lectures, not as much membrane