Benzene EASE 2 PDF
Document Details
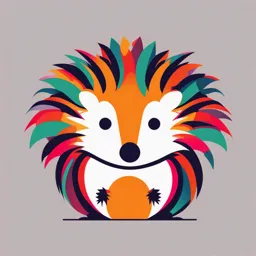
Uploaded by TriumphantWilliamsite9550
Pribadi Depok
Tags
Summary
This document introduces the benzene ring, a core concept in organic chemistry. It discusses structural characteristics, bond lengths, and bonding in benzene.
Full Transcript
25.1 The benzene ring The ‘benzene ring’ is a particularly important functional group found in many organic compounds. A benzene ring is a hexagon made of six carbon atoms bonded together in a particular way. Benzene rings are found in many compounds that are commercially important: for example, as...
25.1 The benzene ring The ‘benzene ring’ is a particularly important functional group found in many organic compounds. A benzene ring is a hexagon made of six carbon atoms bonded together in a particular way. Benzene rings are found in many compounds that are commercially important: for example, as medicines, dyes and plastics. Organic hydrocarbons containing one or more benzene rings are called arenes. In general, compounds of benzene are known as aryl compounds or aromatic compounds; an example is chlorobenzene, which is one of the halogenoarenes. As you read in the section above, in Kekulé’s structure the hexagonal ring contained three double C═C bonds. This is reflected in benzene’s name, which has the same ending as the alkenes. As chemists developed analytical techniques, however, they found out that the benzene molecule was a planar, perfectly symmetrical molecule. Kekulé’s structure would suggest three shorter double C═C bonds and three longer C─C single bonds in the ring. This would produce a distorted hexagonal shape, not the perfect hexagonal arrangement of carbon atoms in benzene’s actual molecules. Figure 25.3 shows how we represent benzene’s skeletal formula. Figure 25.3: The skeletal formula of benzene. It can also be used in displayed formulae of aryl compounds, as in the figure below Figure 25.2, showing the structure of vanillin. We can now measure actual bond lengths. This was impossible in the 19th century when Kekulé worked. Table 25.1 shows that the bond length of the carbon–carbon bonds in benzene lies between the values for C─C single bonds and C═C double bonds. The chemistry of benzene also suggests that the Kekulé structure is incorrect. If there were three C═C bonds in benzene it would undergo addition reactions in the same way as the alkenes (see Section 15.3). However, this is not the case. For example, ethene will decolorise bromine water on mixing at room temperature, but benzene needs much harsher conditions. Bond Bond length / nm C─C 0.154 C═C 0.134 carbon to carbon bond in benzene 0.139 Table 25.1: Comparing bond lengths. The actual structure of benzene can be explained by considering the bonding in the molecule. Each of the six carbon atoms in the hexagonal ring is sp2 hybridised (see Section 4.5), sharing: one pair of electrons with one of its neighbouring carbon atoms one pair of electrons with its other neighbouring carbon atom one pair of electrons with a hydrogen atom. These are three σ (sigma) bonds. These covalent bonds are a pair of electrons found mainly between the nuclei of the atoms bonded to each other. Each carbon atom with sp2 hybrised atomic orbitals forms three σ bonds: so that leaves one electron spare on each of the six carbon atoms in benzene. Each carbon atom contributes its one spare electron to a π (pi) bond (see Section 4.5). However, the π bonds formed by benzene are not the same as the π bonds in an alkene C═C bond (see Section 4.5). In an alkene, a π bond helps to bond one pair of carbon atoms to each other; but in benzene six spare p orbital electrons are spread over all six carbon atoms in the hexagonal ring. The six electrons in the π bonds are said to be delocalised. The π bonding in benzene is formed by the overlap of carbon p atomic orbitals, one from each of the six carbon atoms. To achieve maximum overlap, the benzene molecule must be planar. The lobes of the p orbitals overlap to form a ring of delocalised electrons above and below the plane of the carbon atoms in the benzene molecule. This is shown in Figure 25.4. Figure 25.4: The π bonding in benzene. The three bond angles around each of the sp2 hybridised carbon atoms are 120°. Naming aryl compounds You saw how to name aryl compounds with alkyl side-chains in Section 14.3. Some aryl compounds have functional groups that are substituted directly into the benzene ring in place of a hydrogen atom. You need to know the names of the compounds in Table 25.2. Skeletal formula of aryl compound Name chlorobenzene nitrobenzene phenol 2,4,6-tribromophenol: note the numbering of the carbon atoms in the benzene ring to describe the position of each substituted group (see Section 14.3) phenylamine Table 25.2: The names of some aryl compounds. The phenyl group in a substituted benzene compound can be written as C6H5; e.g. the structural formula of phenylamine is C6H5NH2. Question 1 a Give the number of electrons involved in the π bonding system in a benzene molecule. b State the type of atomic orbital that the electrons in part a come from. c Explain what we mean by the term delocalised electrons in benzene. d Compare the π bonding in benzene with the π bonding in hex-3-ene. e Draw the displayed or skeletal formula of: i 1,3,5-tribromobenzene ii 1,3-dichloro-5-nitrobenzene. f Name the molecules below: i ii Choose a partner and discuss your answers to Question 1 part d together. Then write a mark scheme for this question showing what each mark is given for. Compare your mark scheme with those produced by other pairs of learners. 25.2 Reactions of arenes Most reactions of benzene and other arenes maintain the highly stable delocalised ring of π bonding electrons intact. This occurs by substituting an atom, or group of atoms, for one or more hydrogen atoms attached to the benzene ring. Addition reactions into the benzene ring would disrupt the ‘aromatic stabilisation’ achieved by the complete delocalisation in the ring. The initial attack is usually by an electrophile, which is attracted to the high electron density around the benzene ring. Electrophilic substitution with chlorine or bromine Benzene will react with bromine in the presence of an anhydrous aluminium bromide catalyst. The substitution reaction is: At first sight, the electrophile that starts the attack on benzene is not obvious. The electrophile is created when an aluminium bromide molecule polarises a bromine molecule. The Br2 molecule forms a dative (co- ordinate) bond with aluminium bromide by donating a lone pair of electrons from one of its bromine atoms into an empty 3p orbital in the aluminium. This draws electrons from the other bromine atoms in the Br2 molecule, making it partially positive, and creating the electrophile. We can think of the electrophile as a Br+ cation: The Br+ cation and the ‘electron-rich’ benzene ring are attracted to each other, as the mechanism of electrophilic substitution below shows. Remember that the curly arrows show the movement of a pair of electrons: A similar reaction happens when chlorine gas is bubbled through benzene at room temperature in the presence of a catalyst, such as aluminium chloride. The products of this electrophilic substitution are chlorobenzene and hydrogen chloride. The catalysts in these reactions, AlBr3 and AlCl3, are known as halogen carriers. When we halogenate methylbenzene or other alkylarenes, the halogen atom substitutes into the benzene ring at positions 2 or 4. These positions are ‘activated’ by any electron-donating groups bonded directly to the benzene ring (see Table 25.4 at the end of the chapter). Other examples of benzene compounds that are activated in these positions are phenol (C6H5OH) and phenylamine (C6H5NH2). So when we react methylbenzene with chlorine gas, using an anhydrous aluminium chloride catalyst, two products can be made: If excess chlorine gas is bubbled through, we can form 2,4-dichloromethylbenzene, 2,6- dichloromethylbenzene and 2,4,6-trichloromethylbenzene. (Remember that the 2 and 6 positions in substituted arenes are equivalent.) The carbon–halogen bond in halogenoarenes is stronger than the equivalent bond in a halogenoalkane, making the halogenoarenes much less reactive. This is because one of the lone pairs on the halogen atom overlaps slightly with the π bonding system in the benzene ring. This gives the carbon–halogen bond in a halogenoarene a partial double bond character. Notice that the methyl side-chain off the benzene ring is not affected under the conditions used in the reaction above. However, we learned in Section 15.2 that chlorine will react with alkanes in the presence of ultraviolet (UV) light or strong sunlight. This reaction of the alkanes is a free-radical substitution reaction. So, if the chlorine gas is passed into boiling methylbenzene in the presence of UV light, the following reaction takes place: Note that there is no substitution into the benzene ring under these conditions. In excess chlorine, eventually all three of the hydrogen atoms on the methyl side-chain will be replaced by chlorine atoms. Question 2 a Write the equation for the reaction of chlorine with benzene in the presence of an aluminium chloride catalyst. b Name this type of mechanism for the reaction in part a. c Draw the mechanism of the reaction in part a, with Cl+ as the attacking species and using curly arrows to show the movement of electron pairs. d Draw the displayed formula of the ‘tri-substituted’ halogenoarene produced if methylbenzene is added to excess bromine at room temperature in the presence of aluminium bromide. e Suggest how the reaction in part d would differ if the methylbenzene and bromine were boiled in the presence of UV light. f Name the mechanism of the reaction in part e. Nitration of benzene The nitration of benzene is another example of an electrophilic substitution. Nitration refers to the introduction of the ─NO2 group into a molecule. In this reaction the electrophile is the NO2+ ion, known as the nitronium ion (or nitryl cation). This is made from a mixture of concentrated nitric acid and concentrated sulfuric acid: HNO3 + 2H2SO4 → NO2+ + 2HSO4a− + H3O+ This ‘nitrating mixture’ is refluxed with benzene at between 25 °C and 60 °C to make nitrobenzene: The mechanism of the electrophilic substitution in this nitration is: In stage 1 in the mechanism, the electrophile, NO2+, is attracted to the high electron density of the π bonding system in benzene. A pair of electrons from the benzene ring is donated to the nitrogen atom in NO2+, and forms a new covalent bond. At this point, benzene’s delocalised ring of electrons is disrupted. There are now four π bonding electrons and a positive charge spread over five carbon atoms. However, the full delocalised ring is restored in stage 2 when the C─H bond breaks heterolytically (see Section 14.7). Both electrons in the C─H covalent bond go into nitrobenzene’s π bonding system, and hydrogen leaves as an H+ ion. There are now six electrons spread over the six carbon atoms, so the chemical stability of the benzene ring is retained in this substitution reaction. In order to predict the products of further substitution into the benzene ring of the nitrobenzene formed, we must consider the ‘directing effect’ of the nitro group. Further nitration of the nitrobenzene produces 1,3- dinitrobenzene and 1,3,5-trinitrobenzene. Unlike the electron-donating methyl group in methylbenzene (which activates the 2 and 4 positions in the benzene ring), the ─NO2 group is electron-withdrawing. This type of group (which includes ─COOH) deactivates the 2 and 4 positions in the benzene ring. Therefore, when there is a nitro group bonded to the benzene ring, further substitutions are directed to the 3 and 5 positions (see Table 25.4 at the end of the chapter). Question 3 a Copy and complete the two equations below, which can both be used to show the nitration of methylbenzene: i C6H5CH3 + NO2+ → ____+____ ii C6H5CH3 + HNO3 ____+____ iii Name the possible mono-substituted products in parts i and ii. iv 2,4-dinitromethylbenzene and 2,4,6-trinitromethylbenzene are formed on further nitration of methylbenzene. Draw the displayed formula of each compound. b Benzene also undergoes electrophilic substitution when refluxed with fuming sulfuric acid for several hours. This is called sulfonation. The electrophile is the SO3 molecule and the product formed is benzenesulfonic acid, C6H5SO3H. i Suggest which atom in the SO3 molecule accepts an electron pair in the mechanism of sulfonation. ii Write an equation in the style of part a i for the sulfonation of benzene to form benzenesulfonic acid. Discuss and sketch with a partner the mechanism of sulfonation in part b above. If you get stuck, work with another group before displaying your suggested mechanism. Alkylation (or acylation) of benzene (Friedel–Crafts reactions) Friedel–Crafts reactions, named after the chemists who first discovered them, are a third example of electrophilic substitution into the benzene ring. Sometimes chemists need to change the structure of an arene in order to make a new product. Examples include the manufacture of detergents or the reactants needed to make plastics, such as poly(phenylethene), commonly known as polystyrene. They can use a Friedel–Crafts reaction to substitute a hydrogen in the benzene ring for an alkyl group, such as a methyl (─CH3) or an ethyl (─C2H5) group: The same type of reaction can also be used to introduce an acyl group into a benzene ring. An acyl group contains an alkyl group and a carbonyl (C═O) group: IMPORTANT Friedel–Crafts reactions result in the introduction of a side-chain into a benzene ring. Friedel–Crafts reactions are also called alkylation or acylation reactions. For example: The mechanisms for Friedel–Crafts reactions involve attack on the benzene ring by an electrophile. In these reactions, the electrophile carries a positive charge on a carbon atom, so there is attack by a carbocation. The electrophile is formed by adding an aluminium chloride catalyst to: a halogenoalkane, to make an alkylbenzene, or an acyl chloride, to make an acylbenzene. This creates the carbocation electrophile to attack the benzene in the first step of the two mechanisms below. In alkylation The alkylation of benzene, substituting an alkyl chain into the benzene ring, can be described by this three- step mechanism: Step 1: The arrow between Cl and AlCl3 represents a coordinate (dative covalent) bond. The carbocation electrophile made then attacks the benzene ring: Step 2: The aluminium chloride catalyst is regenerated in the final step: Step 3: Further alkylation of the benzene ring can take place as the reaction proceeds. In acylation The acylation of benzene, substituting an acyl group into the benzene ring, can be described by this three- step mechanism: Step 1: Again, the electrophile made then attacks the benzene ring: Step 2: Then the aluminium chloride catalyst is regenerated in the final step: Step 3: Oxidation of the side-chain in arenes The presence of the benzene ring in an alkylarene, such as methylbenzene, can affect the characteristic reactions of its alkyl side-chain. For example, alkanes are not usually oxidised by a chemical oxidising agent such as potassium manganate(VII). However, in alkylarenes, the alkane side-chain is oxidised to form a carboxylic acid. For example, methylbenzene produces benzoic acid when refluxed with alkaline potassium manganate(VII), and then acidified with dilute sulfuric acid: Hydrogenation Unusually, the hydrogenation reaction is similar to an alkene’s reaction with hydrogen. The unsaturated alkene becomes saturated as an alkane is formed. The reaction is carried out by heating the alkene with hydrogen gas and a nickel or platinum catalyst. The same reaction takes place with benzene. The benzene is hydrogenated and is converted to cyclohexane. With methylbenzene, the product is methylcyclohexane: Question 4 a Copy and complete the two equations, which can be used to show the alkylation and acylation of benzene to produce the mono-substituted products. i C6H6 + CH3CH2CH2Cl → _____ + _____ ii C6H6 + CH3CH2CH2CH2 COCl → _____ + _____ b i Name the mono-substituted organic product in part a i. ii Name the class of compound formed in part a ii. c Hexylbenzene is refluxed with alkaline potassium manganate(VII) and then acidified with dilute sulfuric acid. The same experiment is carried out but using hexane and the oxidising agent. Compare what would happen in these experiments. d i Give the reagents and conditions needed to convert benzene into cyclohexane. ii Which type of reaction classifies the reaction in part d i? A addition B condensation C elimination