Autonomic Nervous System Fall 2024 PDF
Document Details
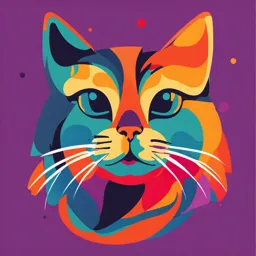
Uploaded by ProblemFreePedalSteelGuitar
Pacific Northwest University of Health Sciences
2024
Tags
Summary
This document is an overview of the autonomic nervous system, including learning objectives, comparisons with somatic systems, and descriptions of the parasympathetic and sympathetic divisions. It is likely part of a larger textbook or study guide on human physiology.
Full Transcript
Nervous System Fall 2024 Autonomic Nervous System FDS October 2nd ‘24 Credits: text originally developed by Dr. Anthony Lyons and edited by Dr. Dan Selski Fundamental Neuroscience (Haines) Chapter 29 – Visceral Motor Pathways Textbook of Medical Physiology (Guyton & Hall) Chapter 61 – T...
Nervous System Fall 2024 Autonomic Nervous System FDS October 2nd ‘24 Credits: text originally developed by Dr. Anthony Lyons and edited by Dr. Dan Selski Fundamental Neuroscience (Haines) Chapter 29 – Visceral Motor Pathways Textbook of Medical Physiology (Guyton & Hall) Chapter 61 – The Autonomic Nervous System Learning Objectives 1. Identify the differences between the somatic and autonomic nervous systems 2. Distinguish between the parasympathetic and sympathetic subsystems of the autonomic nervous system 3. Describe characteristics of preganglionic neurons of the autonomic nervous system 4. Distinguish the differences between sympathetic and parasympathetic ganglia 5. Describe sympathetic and parasympathetic postganglionic neurons and identify the autonomic plexuses 6. Describe features of the sympathetic and parasympathetic division of the autonomic nervous system 7. Describe sympathetic and parasympathetic responses and control of the autonomic nervous system 8. Describe the neurotransmitters and receptors that are involved in autonomic neurotransmission and understand outcomes of pharmacological intervention Comparing the Somatic and Autonomic Nervous Systems The peripheral nervous system includes both a voluntary, somatic branch and an involuntary branch that regulates visceral functions. The peripheral nervous system (PNS) is divided into the somatic nervous system and the autonomic nervous system. The somatic nervous system (SoNS) is the part of the peripheral nervous system associated with the voluntary control of body movements via skeletal muscles. The SoNS consists of efferent nerves responsible for stimulating muscle contraction, including all the non-sensory neurons connected with skeletal muscles and skin. The somatic nervous system controls all voluntary muscular systems within the body, and also mediates involuntary reflex arcs. The autonomic nervous system (ANS) is the part of the peripheral nervous system that acts as a control system, functioning largely below the level of consciousness and controlling visceral functions. The ANS affects heart rate, digestion, respiratory rate, salivation, perspiration, pupillary dilation, micturition (urination), and sexual arousal. Whereas most of its actions are involuntary, some work in tandem with the conscious mind. The enteric nervous system is sometimes considered part of the autonomic nervous system, and sometimes considered an independent system. Divisions of the Autonomic Nervous System The autonomic nervous system (ANS) is classically divided into two subsystems: the parasympathetic nervous system (PSNS) and sympathetic nervous system (SNS). Sympathetic and parasympathetic divisions typically function somewhat in opposition to each other. This opposition is often viewed as complementary in nature rather than antagonistic. For an analogy, one may think of the sympathetic division as the accelerator and the parasympathetic division as the brake. The sympathetic division typically functions in actions requiring quick responses. The parasympathetic division functions with actions that do not require immediate reaction. Many think of sympathetic as fight or flight and parasympathetic as rest and digest or feed and breed. However, many instances of sympathetic and parasympathetic activity cannot be ascribed to fight or rest situations. For example, standing up from a reclining or sitting position would entail an unsustainable drop in blood pressure if not for a compensatory increase in the arterial sympathetic tonus. Another example is the constant, second-to-second modulation of heart rate by sympathetic and parasympathetic influences as a function of the respiratory cycles. More generally, these two systems should be seen as permanently modulating vital functions, in usually antagonistic fashion, to achieve homeostasis. Some functions of the SNS include diverting blood flow away from the gastrointestinal (GI) tract and skin via vasoconstriction, enhancing blood flow to skeletal muscles and the lungs, dilating the bronchioles of the lung to allow for greater oxygen exchange, and increasing heart rate. The PSNS typically functions in contrast to the SNS by dilating the blood vessels leading to the GI tract, causing constriction of the pupil and contraction of the ciliary muscle to the lens to enable closer vision, and stimulating salivary gland secretion, in keeping with the rest and digest functions. Preganglionic Neurons In the autonomic nervous system (ANS), nerve fibers that connect the central nervous system to ganglia are known as preganglionic fibers. A ganglia is merely a collection of neuron cell bodies in the peripheral nervous system (i.e.: outside the central nervous system). Sensory ganglia (such as DRG and trigeminal ganglion) have NO synapses within them. Autonomic ganglia are the location of synapses: preganglionic fibers synapse onto the neruons in the ganglia. Thus the neurons located within autonomic ganglia are called “post-ganglionic neurons”. All preganglionic fibers, whether they are in the sympathetic nervous system (SNS) or in the parasympathetic nervous system (PSNS), are cholinergic—that is, these fibers use acetylcholine as their neurotransmitter—and are myelinated. The ANS is unique in that it requires a sequential two-neuron efferent pathway; the preganglionic neuron must first cross a synapse onto a postganglionic neuron before innervating the target organ. The preganglionic, or first neuron, is located in the CNS and the axon projects through the PNS to synapse on the postganglionic, or second neuron’s cell body. The postganglionic neuron will project an axon that synapses on the target organ, which can be a gland or smooth muscle. Sympathetic preganglionic fibers tend to be shorter than parasympathetic preganglionic fibers because sympathetic ganglia are often closer to the spinal cord. Parasympathetic preganglionic fibers tend to project to and synapse with the postganglionic fiber close to the target organ, hence they are longer. Properties of the SNS and PSNS preganglionic neurons also differ with respect to the CNS exit points. The sympathetic division has thoracolumbar outflow, meaning that the neurons begin at the thoracic and lumbar (T1–L2) portions of the spinal cord. The parasympathetic division has craniosacral outflow, meaning that the neurons begin at the cranial nerves (CNIII, CNVII, CNIX, CNX) and sacral (S2–S4) spinal cord. The sympathetic division (thoracolumbar outflow) consists of cell bodies in the lateral horn of the spinal cord (intermediolateral cell columns) from T1 to L2. These cell bodies are GVE (general visceral efferent) neurons and are the preganglionic neurons. There are several locations where preganglionic neurons create synapses with their postganglionic neurons: The paravertebral ganglia of the sympathetic chain (these run on either side of the vertebral bodies), cervical ganglia, thoracic ganglia, lumbar ganglia, and sacral ganglia. The prevertebral ganglia are: celiac ganglion, aorticorenal ganglion, superior mesenteric ganglion, inferior mesenteric ganglion, as well as plexuses along the aorta and down into the pelvis. The chromaffin cells of the adrenal medulla. This is the one exception to the two-neuron pathway rule: preganglionic sympathetic fibers create a synapse directly onto the target cell bodies. The parasympathetic division (craniosacral outflow) consists of cell bodies from one of two locations: the brainstem (cranial nerves III, VII, IX, X) or the sacral spinal cord (S2, S3, S4). These are the preganglionic neurons that synapse with the postganglionic neurons in these locations: Parasympathetic ganglia of the head: 1. Ciliary (CN III). 2. Submandibular (CN VII). 3. Pterygopalatine (CN VII). 4. Otic (CN IX)). In or near the wall of an organ innervated by 5. the vagus (CN X) or 6. pelvic splanchnic nerves (S2, S3, S4). Another major difference between the two ANS systems is divergence, or the number of postsynaptic fibers a single preganglionic fiber creates a synapse with. Whereas in the parasympathetic division there is a divergence factor of roughly 1:4, in the sympathetic division there can be a divergence of up to 1:20. The site of synapse formation and this divergence for both the sympathetic and parasympathetic preganglionic neurons occurs within these ganglia of the peripheral nervous system. Autonomic Ganglia Autonomic ganglia are clusters of neuronal cell bodies and their dendrites. They are essentially a junction between autonomic nerves originating from the central nervous system and autonomic nerves innervating their target organs in the periphery. The two main categories are: sympathetic ganglia and parasympathetic ganglia. An example of parasympathetic ganglion is the ciliary ganglion, involved in pupil constriction and accommodation. Dorsal root ganglia are sensory, and older textbooks do not classify them with the autonomic nervous system. However sensation from organs, glands and smooth muscle follow sympathetic and parasympathetic routes, so more modern desriptions include these in descriptions of the autonomic nervous system. Interestingly, dorsal root ganglia and autonomic ganglia (and the adrenal medulla) develop from neural crest, which separates from the neural tube as it closes to form the future spinal cord. Therefore, the dorsal root and autonomic ganglia can be regarded as extensions of the gray matter of the spinal cord that became translocated to the periphery, as parts of the peripheral nervous system: analogous to the lower motor neurons of the somatic nervous system are extensions of the grey matter of the ventral horn of the spinal cord. A dorsal root ganglion (or spinal ganglion) is a swelling or nodule on a dorsal root that contains the cell bodies of nerve cells (neurons) that carry signals from the sensory organs towards the appropriate integration center. In the PNS, nerves that carry signals towards the brain are known as afferent nerves. The axons of dorsal root ganglion neurons are known as afferents. In the peripheral nervous system, afferents refer to the axons that relay sensory information into the central nervous system (i.e., the brain and the spinal cord). These neurons are of the pseudo-unipolar type, meaning they have an axon with two branches that act as a single axon, often referred to as a distal process and a proximal process. Unlike the majority of neurons found in the central nervous system, an action potential in a dorsal root ganglion neuron may initiate in the distal process in the periphery, bypass the cell body, and continue to propagate along the proximal process until reaching the synaptic terminal in the dorsal horn of the spinal cord. The distal section of the axon may either be a bare nerve ending or encapsulated by a structure that helps relay specific information to a nerve. The nerve endings of dorsal root ganglion neurons have a variety of sensory receptors that are activated by mechanical, thermal, chemical, and noxious stimuli. In these sensory neurons, a group of ion channels thought to be responsible for somatosensory transduction have been identified. For example, a Meissner’s corpuscle or Pacinian corpuscle may encapsulate the nerve ending, rendering the distal process sensitive to mechanical stimulation, such as stroking or vibration, respectively. Sympathetic Ganglia Sympathetic ganglia are the ganglia of the sympathetic nervous system. They deliver information to the body about stress and impending danger, and are responsible for the familiar fight-or-flight response. This response is also known as the sympathetico-adrenal response because the pre-ganglionic sympathetic fibers that end in the adrenal medulla—like all sympathetic fibers—secrete acetylcholine. This secretion activates the secretion of adrenaline (epinephrine) and to a lesser extent noradrenaline (norepinephrine) from the adrenal medulla. Therefore, this response is mediated directly via impulses transmitted through the sympathetic nervous system, and indirectly via catecholamines secreted from the adrenal medulla, and acts primarily on the cardiovascular system. They contain approximately 20,000–30,000 nerve cell bodies and are located close to and on either side of the spinal cord in long chains. Sympathetic ganglia are the tissue from which neuroblastoma tumors arise. The bilaterally symmetric sympathetic chain ganglia —also called the paravertebral ganglia —are located just ventral and lateral to the spinal cord. The chain extends from the upper neck down to the coccyx. Preganglionic nerves from the spinal cord create a synapse at one of the chain ganglia, and the postganglionic fiber extends to an effector, typically a visceral organ in the thoracic cavity. There are usually 21 or 23 pairs of these ganglia: three in the cervical region, 12 in the thoracic region, four in the lumbar region, four in the sacral region and a single, unpaired ganglion lying in front of the coccyx called the ganglion impar. Neurons of the collateral ganglia, also called the prevertebral ganglia, receive input from the splanchnic nerves and innervate organs of the abdominal and pelvic region. These include the celiac ganglia, superior mesenteric ganglia, and inferior mesenteric ganglia. Parasympathetic Ganglia Parasympathetic ganglia are the autonomic ganglia of the parasympathetic nervous system. Most are small terminal ganglia or intramural ganglia, so named because they lie near or within (respectively) the organs they innervate. The exceptions are the four paired parasympathetic ganglia of the head. Each has three roots entering the ganglion (motor, sympathetic, and sensory roots) and a variable number of exiting branches. 1. The motor root carries presynaptic parasympathetic nerve fibers (general visceral efferent fibers) that terminate in the ganglion by creating a synapse for the postsynaptic fibers traveling to target organs. 2. The sympathetic root carries postsynaptic sympathetic fibers (general visceral efferent fibers) that traverse the ganglion without creating a synapse. 3. The sensory root carries general sensory fibers (general somatic afferent fibers) that also do not create a synapse in the ganglion. Some cranial ganglia also carry special sensory fibers (special visceral afferent) for taste sensation. Efferent parasympathetic nerve signals are carried from the central nervous system to their targets by a system of two neurons. The first neuron in this pathway is referred to as the preganglionic or presynaptic neuron. Its cell body sits in the central nervous system and its axon usually extends to a ganglion somewhere else in the body, where it synapses with the dendrites of the second neuron in the chain. This second neuron is referred to as the postganglionic or postsynaptic neuron. The axons of presynaptic parasympathetic neurons are usually long. They extend from the CNS into a ganglion that is either very close to or embedded in their target organ. As a result, the postganglionic parasympathetic nerve fibers are usually very short. In the autonomic nervous system, fibers from the ganglion to the effector organ are called postganglionic fibers. The post-ganglionic neurons are directly responsible for changes in the activity of the target organ via biochemical modulation and neurotransmitter release. The neurotransmitters used by postganglionic fibers differ. In the parasympathetic division, they are cholinergic and use acetylcholine as their neurotransmitter. In the sympathetic division, most are adrenergic, meaning they use norepinephrine as their neurotransmitter. The Sympathetic Fibers At the synapses within the ganglia, the preganglionic neurons release acetylcholine, a neurotransmitter that activates nicotinic acetylcholine receptors on postganglionic neurons. In response to this stimulus, postganglionic neurons—with two important exceptions—release norepinephrine, which activates adrenergic receptors on the peripheral target tissues. The activation of target tissue receptors causes the effects associated with the sympathetic system. The two exceptions mentioned above are the postganglionic neurons of sweat glands and the chromaffin cells of the adrenal medulla. The postganglionic neurons of sweat glands release acetylcholine for the activation of muscarinic receptors. The chromaffin cells of the adrenal medulla are analogous to post-ganglionic neurons—the adrenal medulla develops in tandem with the sympathetic nervous system and acts as a modified sympathetic ganglion. Within this endocrine gland, the pre- ganglionic neurons create synapses with chromaffin cells and stimulate the chromaffin cells to release norepinephrine and epinephrine directly into the blood. Presynaptic nerves’ axons terminate in either the paravertebral ganglia or prevertebral ganglia. In all cases, the axon enters the paravertebral ganglion at the level of its originating spinal nerve. After this, it can then either create a synapse in this ganglion, ascend to a more superior ganglion, or descend to a more inferior paravertebral ganglion and make a synapse there, or it can descend to a prevertebral ganglion and create a synapse there with the postsynaptic cell. The postsynaptic cell then goes on to innervate the targeted end effector (i.e., gland, smooth muscle, etc.). Because paravertebral and prevertebral ganglia are relatively close to the spinal cord, presynaptic neurons are generally much shorter than their postsynaptic counterparts, which must extend throughout the body to reach their destinations. The Parasympathetic Fibers The axons of presynaptic parasympathetic neurons are usually long. They extend from the CNS into a ganglion that is either very close to or embedded in their target organ. As a result, the postsynaptic parasympathetic nerve fibers are very short. In the cranium, preganglionic fibers (cranial nerves III, VII, and IX) usually arise from specific nuclei in the central nervous system (CNS) and create a synapse at one of four parasympathetic ganglia: ciliary, pterygopalatine, otic, or submandibular. From these four ganglia the postsynaptic fibers complete their journey to target tissues via cranial nerve V (the trigeminal ganglion with its ophthalmic, maxillary, and mandibular branches). The vagus nerve does not participate in these cranial ganglia, as most of its fibers are destined for a broad array of ganglia on or near the thoracic viscera (esophagus, trachea, heart, lungs) and the abdominal viscera (stomach, pancreas, liver, kidneys). It travels all the way down to the midgut/hindgut junction, which occurs just before the splenic flexure of the transverse colon. The pelvic splanchnic efferent preganglionic nerve cell bodies reside in the lateral gray horn of the spinal cord at the S2–S4 spinal levels. Their axons continue away from the CNS to synapse at an autonomic ganglion close to the organ of innervation. This differs from the sympathetic nervous system, where synapses between pre- and post-ganglionic efferent nerves in general occur at ganglia that are farther away from the target organ. The parasympathetic nervous system uses acetylcholine (ACh) as its chief neurotransmitter, although peptides (such as cholecystokinin) may act on the PSNS as a neurotransmitter. The ACh acts on two types of receptors, the muscarinic and nicotinic cholinergic receptors. Most transmissions occur in two stages: When stimulated, the preganglionic nerve releases ACh at the ganglion, which acts on the nicotinic receptors of the postganglionic neurons. The postganglionic nerve then releases ACh to stimulate the muscarinic receptors of the target organ. Autonomic Plexuses Autonomic plexuses are formed from sympathetic and parasympathetic fibers that innervate and regulate the overall activity of visceral organs. The autonomic plexuses include: the cardiac plexus, the pulmonary plexus, the esophageal plexus, the abdonimal aortic plexus, and the superior and inferior hypogastric plexuses. Autonomic plexuses are formed from sympathetic postganglionic axons, parasympathetic preganglionic axons, and some visceral sensory axons. Plexuses provide a complex innervation pattern to the target organs, since most organs are innervated by both divisions of the autonomic nervous system. Autonomic Reflexes Autonomic reflexes are unconscious motor reflexes relayed from the organs and glands to the CNS through visceral afferent signaling. While the unconscious reflex arcs are normally undetectable, in certain instances they may trigger pain, typically masked as referred pain. The sympathetic nervous system is a quick-response, mobilizing system while the parasympathetic system is a more slowly activated, dampening system—but there are exceptions, such as in sexual arousal and orgasm where both systems play a role. Within the brain, the ANS is located in the medulla oblongata in the lower brainstem. The medulla’s major ANS functions include respiration, cardiac regulation, vasomotor activity, and certain reflex actions (such as coughing, sneezing, vomiting, and swallowing). Sympathetic Responses The sympathetic division of the autonomic nervous system maintains internal organ homeostasis and initiates the stress response. Alongside the other two components of the autonomic nervous system, the sympathetic nervous system aids in the control of most of the body’s internal organs. Stress—as in the hyperarousal of the flight-or-fight response— is thought to counteract the parasympathetic system, which generally works to promote maintenance of the body at rest. The sympathetic nervous system is responsible for regulating many homeostatic mechanisms in living organisms. Fibers from the SNS innervate tissues in almost every organ system and provide physiological regulation over diverse body processes including pupil diameter, gut motility (movement), and urinary output. The SNS is perhaps best known for mediating the neuronal and hormonal stress response commonly known as the fight-or-flight response, also known as sympatho-adrenal response of the body. This occurs as the preganglionic sympathetic fibers that end in the adrenal medulla secrete acetylcholine, which activates the secretion of adrenaline (epinephrine), and to a lesser extent noradrenaline (norepinephrine). Therefore, this response is mediated directly via impulses transmitted through the sympathetic nervous system, and also indirectly via catecholamines that are secreted from the adrenal medulla, and acts primarily on the cardiovascular system. Messages travel through the SNS in a bidirectional flow. Efferent messages can trigger simultaneous changes in different parts of the body. For example, the sympathetic nervous system can accelerate heart rate, widen bronchial passages, decrease motility of the large intestine, constrict blood vessels, increase peristalsis in the esophagus, cause pupillary dilation, piloerection (goose bumps) and perspiration (sweating), and raise blood pressure. Afferent messages carry sensations such as heat, cold, or pain. Some evolutionary theorists suggest that the sympathetic nervous system operated in early organisms to maintain survival since the sympathetic nervous system is responsible for priming the body for action. One example of this priming is in the moments before waking, in which sympathetic outflow spontaneously increases in preparation for activity. The Fight-or-Flight Response Catecholamine hormones, such as adrenaline or noradrenaline, facilitate the immediate physical reactions associated with a preparation for violent muscular action. These include the following: Acceleration of heart and lung action. Paling or flushing, or alternating between both. Inhibition of stomach and upper-intestinal action to the point where digestion slows down or stops. General effect on the sphincters of the body. Constriction of blood vessels in many parts of the body. Liberation of nutrients (particularly fat and glucose) for muscular action. Dilation of blood vessels for muscles. Inhibition of the lacrimal gland (responsible for tear production) and salivation. Dilation of pupil (mydriasis). Relaxation of bladder. Inhibition of erection. Auditory exclusion (loss of hearing). Tunnel vision (loss of peripheral vision). Disinhibition of spinal reflexes; and shaking. In prehistoric times, the human fight-or-flight response manifested fight as aggressive, combative behavior and flight as fleeing potentially threatening situations, such as being confronted by a predator. In current times, these responses persist, but fight-and-flight responses have assumed a wider range of behaviors. For example, the fight response may be manifested in angry, argumentative behavior, and the flight response may be manifested through social withdrawal, substance abuse, and even television viewing. Parasympathetic Responses The parasympathetic nervous system regulates organ and gland functions during rest and is considered a slowly activated, dampening system. A useful acronym to summarize the functions of the parasympathetic nervous system is SLUDD (salivation, lacrimation, urination, digestion, and defecation). The parasympathetic nervous system may also be known as the parasympathetic division. The parasympathetic nervous system uses chiefly acetylcholine (ACh) as its neurotransmitter, although peptides (such as cholecystokinin) may act on the PSNS as neurotransmitters. The ACh acts on two types of receptors, the muscarinic and nicotinic cholinergic receptors. Most transmission occurs in two stages. When stimulated, the preganglionic nerve releases ACh at the ganglion, which acts on nicotinic receptors of the postganglionic neurons. The postganglionic nerve then releases ACh to stimulate the muscarinic receptors of the target organ. Control of Autonomic Nervous System Function The medulla oblongata, in the lower half of the brainstem, is the control center of the autonomic nervous system. Within the brain, the ANS is located in the medulla oblongata in the lower brainstem. The medulla’s main functions are to control the cardiac, respiratory, and vasomotor centers, to mediate autonomic, involuntary functions, such as breathing, heart rate, and blood pressure, and to regulate reflex actions such as coughing, sneezing, vomiting, and swallowing. The hypothalamus acts to integrate autonomic functions and receives autonomic regulatory feedback from the limbic system to do so. The ANS is classically divided into two subdivisions, the sympathetic division and the parasympathetic division. The sympathetic division of the ANS is often referred to as the sympathetic nervous system (SNS). The SNS provides noradrenergic drive to the ANS. It is often referred to as a quick response mobilizing system that initiates the body’s fight-or-flight response. PSNS input to the ANS is responsible for the stimulation of feed-and-breed and rest-and-digest responses, as opposed to the fight-or-flight response initiated by the SNS. The parasympathetic division of the ANS (PSNS) acts to complement and modulate the drive provided by SNS neurotransmission within the ANS. As a rule, the SNS functions in actions requiring quick responses while the PSNS is initiated in actions that don’t require immediate response. Neurotransmitters All somatic motor neurons release acetylcholine (ACh) at their synapses with skeletal muscle fibers. The effect is always excitatory, and if stimulation reaches threshold, the muscle fibers contract. Neurotransmitters released onto visceral effector organs by postganglionic autonomic fibers include norepinephrine (NE) secreted by most sympathetic fibers, and ACh released by parasympathetic fibers. Depending on the type of receptors present on the target organ, the organ’s response may be either excitation or inhibition. Cholinergic Receptors The two types of receptors that bind ACh are named for drugs that bind to them and mimic acetylcholine’s effects. The first of these receptors identified were the nicotinic receptors, which respond to nicotine. A mushroom poison, muscarine, activates a different set of ACh receptors, named muscarinic receptors. All ACh receptors are either nicotinic or muscarinic. Nicotinic receptors are found on (1) the sarcolemma of skeletal muscle cells at neuromuscular junctions (which, as you will recall, are somatic and not autonomic targets), (2) all ganglionic neurons, both sympathetic and parasympathetic, and (3) the hormone-producing cells of the adrenal medulla. The effect of ACh binding to nicotinic receptors anywhere is always stimulatory. Just as at the sarcolemma of skeletal muscle, ACh binding to any nicotinic receptor directly opens ion channels, depolarizing the postsynaptic cell. Muscarinic receptors occur on all effector cells stimulated by postganglionic cholinergic fibers—that is, all parasympathetic target organs and a few sympathetic targets, such as eccrine sweat glands and some blood vessels of skeletal muscles. The effect of ACh binding to muscarinic receptors can be either inhibitory or stimulatory, depending on the subclass of muscarinic receptor found on the target organ. For example, binding of ACh to cardiac muscle receptors slows heart activity, whereas ACh binding to receptors on smooth muscle of the gastrointestinal tract increases its motility. Adrenergic Receptors There are also two major classes of adrenergic (NE-binding) receptors: alpha (α) and beta (β). These receptors are further divided into subclasses (α1 and α2; β1, β2, and β3). Organs that respond to NE (or to epinephrine) have one or more of these receptor subtypes. NE or epinephrine can have either excitatory or inhibitory effects on target organs depending on which subclass of receptor predominates in that organ. For example, binding of NE to the β1 receptors of cardiac muscle prods the heart into more vigorous activity, whereas epinephrine binding to β2 receptors in bronchiole smooth muscle causes it to relax, dilating the bronchiole. The Effects of Drugs Drugs that modify autonomic function can act at the level of neurotransmitter synthesis, storage, or release, as agonists or antagonists at receptors, or by modifying action potential propagation or signal termination. At standard doses, some drugs produce highly specific effects (e.g. by acting at a specific subtypes of cholinergic or adrenergic receptors); others produce more general effects by acting on processes common to all autonomic neurons (e.g. those that inhibit action potential propagation). For example, cholinergic drugs can exert their actions at neuromuscular junctions, at autonomic ganglia, and/or at target tissues of postganglionic parasympathetic neurons and some postganglionic sympathetic neurons. Knowing the locations of the cholinergic and adrenergic receptor subtypes allows specific drugs to be prescribed to obtain the desired inhibitory or stimulatory effects on selected target organs. For example, atropine is an anticholinergic drug that blocks muscarinic ACh receptors. It is routinely administered before surgery to prevent salivation and to dry up respiratory system secretions. Ophthalmologists also use it to dilate the pupils for eye examination. The anticholinesterase drug neostigmine inhibits the enzyme acetylcholinesterase, preventing enzymatic breakdown of ACh and allowing it to accumulate in synapses. This drug is used to treat myasthenia gravis, a condition in which skeletal muscle activity is impaired for lack of ACh stimulation. NE is one of our “feeling good” neurotransmitters, and drugs that prolong the activity of NE on the postsynaptic membrane help to relieve depression. Hundreds of over-the-counter drugs used to treat colds, coughs, allergies, and nasal congestion contain sympathomimetics (phenylephrine and others), sympathetic-mimicking drugs that stimulate α- adrenergic receptors. Much pharmaceutical research is directed toward finding drugs that affect only one subclass of receptor without upsetting the whole adrenergic or cholinergic system. An important breakthrough was finding drugs that mainly activate β2 receptors. People with asthma use such β2 activators to dilate their lung bronchioles without activating β1 receptors, which would increase their heart rate.