Atomic Configuration and Bonding PDF
Document Details
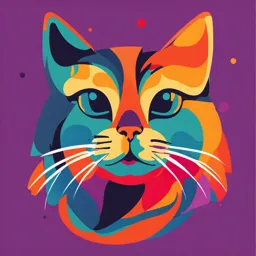
Uploaded by GiftedSagacity1380
Tags
Summary
This document discusses atomic configuration and bonding concepts. It includes diagrams and explanations of various types of bonding, such as ionic, covalent, and metallic. Definitions and examples are provided, making it a great resource for chemistry students at the undergraduate level.
Full Transcript
ATOMIC STRUCTURE and BONDING Atom Nucleus Orbiting Electrons Protons Neutrons Positive Electrically charge neutral Definitions Molecule: Group of atoms bonded together by strong primary bonds (e.g. Diatomic m...
ATOMIC STRUCTURE and BONDING Atom Nucleus Orbiting Electrons Protons Neutrons Positive Electrically charge neutral Definitions Molecule: Group of atoms bonded together by strong primary bonds (e.g. Diatomic molecule, O2, H2,…, Compounds, CH4, H2O ) Atomic number (Z): Number of protons in the nucleus or equivalently number of electrons for a neutral atom. Avagdro’s Number (NA) : Number of atoms or molecules per mole and it is equal to 6.023 x 1023 (atoms/mole) Atomic mass: The sum of masses of protons and neutrons with in the nucleus for an avagdro’s number of atoms. NA (atoms/mole)* [1.67x10-24 (mass of protons) *number of protons + 1.67x10-24 (mass of neutrons ) * number of neutrons] = units is g/mole * As an example one mole of iron contains 6.023 x 1023 atoms and has a mass of 55.847 grams atomic weight (g/mole) BOHR ATOM orbital electrons: n = principal quantum number 1 n=3 2 Adapted from Fig. 2.1, Callister 6e. Nucleus: Z = # protons = 1 for hydrogen to 94 for plutonium N = # neutrons Atomic mass A ≈ Z + N 2 Naturally Occurring Isotopes Some elements have naturally occurring isotopes ( Same atomic number --- same number of protons but different number of Neutrons ----atom mass IS different) C12 C13 C14 Z=6 Z=6 Z=6 N=6 N=7 N=8 Carbon-12 is stable, meaning it never undergoes radioactive decay. Carbon-14 is unstable and undergoes radioactive decay with a half- life of about 5,730 years (meaning that half of the material will be gone after 5,730 years). Such reference decay allows the accurate dating of archaeological artifacts. Electron Configuration Stable Electron Configuration: The outer most shell is completely filled. The s and p states for the outermost shell are filled by a total of 8 electrons SURVEY OF ELEMENTS Most elements: Electron configuration not stable. Electron configuration 1s1 1s2 (stable) 1s22s1 1s22s2 1s22s22p1 Adapted from Table 2.2, 1s22s22p2 Callister 6e.... 1s22s22p6 (stable) 1s22s22p63s1 1s22s22p63s2 1s22s22p63s23p1... 1s22s22p63s23p6 (stable)... 1s22s22p63s23p63d10 4s246 (stable) Why? Valence (outer) shell is usually not filled completely. Valence: Number of electrons in the outer most combined sp level. Deviations from expected electronic structures ex: Fe - atomic # =26 1s2 2s2 2p6 3s2 3p6 3d 6 4s2 valence The unfilled 3d level causes the magnetic behavior of iron electrons Valence electrons – those electrons in the outermost unfilled shells Filled shells more stable Valence electrons are most available for bonding and tend to control the chemical properties – example: C (atomic number = 6) 1s2 2s2 2p2 Example Example Electronegativity: Tendency of an atom to gain an electron and become a negative charged ion. Elements that have almost completely filled outer energy level are strongly electronegative. Electropositivity: Tendency to give up electrons and become positive charged ions. Elements that have nearly empty outer levels readily give up electrons. THE PERIODIC TABLE Columns: Similar Valence Structure Adapted from Fig. 2.6, Callister 6e. Electropositive elements: Electronegative elements: Readily give up electrons Readily acquire electrons to become + ions. to become - ions. 6 ELECTRONEGATIVITY Ranges from 0.7 to 4.0, Large values: tendency to acquire electrons. Smaller electronegativity Larger electronegativity Adapted from Fig. 2.7, Callister 6e. (Fig. 2.7 is adapted from Linus Pauling, The Nature of the Chemical Bond, 3rd edition, Copyright 1939 and 1940, 3rd edition. Copyright 1960 by Cornell University. 7 PRIMARY ATOMIC BONDING IONIC BONDING: Metallic elements give their valence electrons to non metallic elements, doing so the atoms acquire stable configuration Occurs between + and - ions. Requires electron transfer. Large difference in electronegativity required. Example: NaCl EXAMPLES: IONIC BONDING Predominant bonding in Ceramics (metallic and non metallic) NaCl MgO H He 2.1 CaF2 - Li Be O F Ne 1.0 1.5 CsCl 3.5 4.0 - Na Mg Cl Ar 0.9 1.2 3.0 - K Ca Ti Cr Fe Ni Zn As Br Kr 0.8 1.0 1.5 1.6 1.8 1.8 1.8 2.0 2.8 - Rb Sr I Xe 0.8 1.0 2.5 - Cs Ba At Rn 0.7 0.9 2.2 - Fr Ra 0.7 0.9 Give up electrons Acquire electrons Bonding energy is large, thus known to have high melting temperatures (e.g. MgO Tm=2800 C), ionic materials are known to be hard and brittle, electrically and thermally insulative. Ionic bond – metal + nonmetal donates accepts electrons electrons Dissimilar electronegativities ex: MgO Mg 1s2 2s2 2p6 3s2 O 1s2 2s2 2p4 3s2 Mg2+ 1s2 2s2 2p6 O2- 1s2 2s2 2p6 Ionic bonding is nondirectional ; that is the magnitude of the 16 bond is equal in all directions around the ion. Ionic Bonding Energy – minimum energy most stable – Energy balance of attractive and repulsive terms A B EN = EA + ER = - + n r r Repulsive energy ER Interatomic separation r Net energy EN Adapted from Fig. 2.10(b), Callister & Rethwisch 9e. Attractive energy EA 17 COVALENT BONDING Stable electron configuration is achieved by sharing of electrons. Requires shared electrons Example: CH4 C: has 4 valence e, needs 4 more H: has 1 valence e, needs 1 more Electronegativities are comparable. * Covalently bonded material are strong but posses poor ductility and poor electrical and thermal conductivity. 10 EXAMPLES: COVALENT BONDING H2O column IVA H2 F2 C(diamond) H He 2.1 SiC - Cl2 Li Be C O F Ne 1.0 1.5 2.5 2.0 4.0 - Na Mg Si Cl Ar 0.9 1.2 1.8 3.0 - K Ca Ti Cr Fe Ni Zn Ga Ge As Br Kr 0.8 1.0 1.5 1.6 1.8 1.8 1.8 1.6 1.8 2.0 2.8 - Rb Sr Sn I Xe 0.8 1.0 1.8 2.5 - Cs Ba Pb At Rn 0.7 0.9 1.8 2.2 - Fr Ra 0.7 0.9 Adapted from Fig. 2.7, Callister 6e. (Fig. 2.7 is GaAs adapted from Linus Pauling, The Nature of the Chemical Bond, 3rd edition, Copyright 1939 and 1940, 3rd edition. Copyright 1960 by Cornell University. Molecules with nonmetals Molecules with metals and nonmetals Elemental solids ( diamond (carbon); Silicon, Germanium)(RHS of Periodic Table) Compound solids (about column IVA) It is possible to have interatomic bonds that are partially ionic and partially covalent, and, in fact, very few compounds exhibit pure ionic or covalent bonding. For a compound, the degree of either bond type depends on the relative positions of the constituent atoms in the periodic table or the difference in their electronegativities. The greater the difference in electronegativity, the more ionic the bond. Conversely, the smaller the difference in electronegativity, the greater the degree of covalency. Ionic-Covalent Mixed Bonding % ionic character = x (100%) where XA & XB are Pauling electronegativities Ex: MgO XMg = 1.3 XO = 3.5 The covalent bond is directional. Diamond Two allotropes of carbon are very familiar to us: diamond and graphite. The allotropes of carbon all have the same composition—they are pure carbon— and yet they display dramatically different materials properties. The predominantly covalent bonding in diamond profoundly influences its macroscopic properties. Diamond is one of the highest melting-point materials known with a melting temperature of 3550°C. This is due to the strong covalent bonding between atoms. Diamond also has one of the highest known thermal conductivities (2000 W/(m-K)). For comparison, aluminum (which is an excellent thermal conductor) has a thermal conductivity of only 238 W/(m-K). The high thermal conductivity of diamond is due to the rigidity of its covalently bonded structure. As a material is heated, the atoms vibrate with more energy. When the bonds are stiff, the vibrations are transferred efficiently between atoms, thereby conducting heat. Diamond is the stiffest material with an elastic modulus of 1100 GPa. Diamond is about ten times stiffer than titanium and more than fifteen times stiffer than aluminum On the other hand, diamond is an electrical insulator. All of the valence electrons of each carbon atom are shared with the neighboring atoms, leaving no free electrons to conduct electricity Diamond is one of the hardest substances known, which is why it is often used in cutting tools in industrial applications Graphite, like pure diamond, contains only carbon atoms, but we know from the experience of writing with graphite pencils that the properties of graphite are significantly different from that of diamond. In graphite, the carbon atoms are arranged in layers. In each layer, the carbon atoms are arranged in a hexagonal pattern. There is a bond bond between the layers, but this is a much weaker van der Waals bond METALLIC BONDING Metals have one or two or at the most three valence electrons. These valence electrons are given away forming a sea of electrons between positive charged ions. Arises from a sea of donated valence electrons (1, 2, or 3 from each atom). Adapted from Fig. 2.11, Callister 6e. Primary bond for metals and their alloys 12 SECONDARY BONDING (Van der waal Bonding) Weak electrostatic attraction arising from polarized molecules. Polarized molecule: A molecule whose structure cause a portion of the molecule to have a negative charge while other portion have a positive charge. Molecule induced dipole SECONDARY BONDING (Van der waal Bonding) Arises from interaction between dipoles Fluctuating dipoles Short lived distortions of the electrical symmetry due to atoms constant vibrational motion Adapted from Fig. 2.13, Callister 6e. Hydrogen bonding: Permanent dipoles-molecule induced A special type of -general case: secondary bonding, exist when hydrogen -ex: liquid HCl is one of the dipole. It is the strongest secondary bonding -ex: polymer type Permanent dipoles-molecule induced Binding Energy and Interatomic spacing Interatomic Spacing The equilibrium distance between atoms is caused by a balance between repulsive and attractive forces. In the metallic bond, for example, the attraction between the electrons and the ion cores is balanced by the repulsion between ion cores. Equilibrium separation occurs when the total interatomic energy (IAE) of the pair of atoms is at a minimum, or when no net force is acting to either attract or repel the atoms The minimum energy is the binding energy, or the energy required to create or break the bond. Consequently, materials having a high binding energy also have a high strength and a high melting temperature. Ionically bonded materials have a particularly large binding energy because of the large difference in electronegativities between the ions. Metals have lower binding energies because the electronegativities of the atoms are similar. Young’s modulus Other properties can be related to the force-distance and energy-distance expressions in Figure 2-19. For example, the modulus of elasticity of a material (the slope (E) of the stress-strain curve in the elastic region, also known as Young’s modulus) is related to the slope of the force-distance curve (Figure 2-19). A steep slope, which correlates with a higher binding energy and a higher melting point, means that a greater force is required to stretch the bond; thus, the material has a high modulus of elasticity. Coefficient of thermal expansion (CTE) Another property that can be linked to the binding energy or interatomic force distance curves is the coefficient of thermal expansion (CTE). The CTE, often denoted as , is the fractional change in linear dimension of a material per degree of temperature. It can be written (dL/ L)/(dT), where L is length and T is temperature. The CTE is related to the strength of the atomic bonds. It describes how much the material expand or contract when its temperature is changed. In the case of thin films or coatings on substrates, we are not only concerned about the actual values of thermal expansion coefficients but also the difference between thermal expansion coefficients between the substrate and the film or coating. Too much difference between these causes development of stresses that can lead to delamination or warping of the film or coating. In order for the atoms to move from their equilibrium separation, energy must be supplied to the material. If a very deep interatomic energy (IAE) trough caused by strong atomic bonding is characteristic of the material (Figure 2-20), the atoms separate to a lesser degree and have a low, linear coefficient of thermal expansion. Materials with a low coefficient of thermal expansion maintain their dimensions more closely when the temperature changes Properties From Bonding: Tm Bond length, r Melting Temperature, Tm Energy r Bond energy, Eo ro r Energy smaller Tm unstretched length ro larger Tm r Eo = Tm is larger if Eo is larger. “bond energy” 36 Properties From Bonding: α Coefficient of thermal expansion, α length, L o coeff. thermal expansion unheated, T1 ΔL ΔL = α (T2 -T1) heated, T 2 Lo α ~ symmetric at ro Energy unstretched length ro r α is larger if Eo is smaller. E larger α o E smaller α 37 o