Astronomy Final Exam Study Guide PDF
Document Details
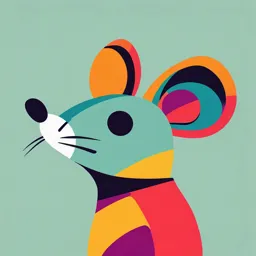
Uploaded by MeaningfulSphinx
Penn State University
Tags
Summary
This document is a study guide for an astronomy final exam, covering fundamental concepts like the scientific method, scientific notation, and measuring distances with light. The guide also touches on topics including the observable universe, components of the universe, daily motion of stars, and more.
Full Transcript
Astronomy Final Exam Study Guide 1. The Scientific Method The scientific method is a systematic process for acquiring knowledge through observation, experimentation, and testing. A scientific theory is a well-substantiated explanation of natural phenomena, based on a body of evidence. Theories are...
Astronomy Final Exam Study Guide 1. The Scientific Method The scientific method is a systematic process for acquiring knowledge through observation, experimentation, and testing. A scientific theory is a well-substantiated explanation of natural phenomena, based on a body of evidence. Theories are tested by making predictions and comparing them to observations. A solid theory is supported by extensive data and repeated tests, while a hypothesis has limited evidence and requires further validation. 2. Scientific Notation Scientific notation is a way to express very large or very small numbers concisely. For example, 3.0 × 10⁸ represents 300,000,000. This notation is useful in astronomy to handle vast distances and sizes efficiently. 3. Measuring Distances with Light Light-years, light-minutes, and light-seconds measure distances based on how far light travels in a year, minute, or second. Light travels at approximately 300,000 kilometers per second. 4. The Observable Universe The observable universe is limited by the speed of light and the age of the universe (~14 billion years). We cannot see beyond this limit because light from more distant regions has not had time to reach us. 5. Components of the Universe The universe consists of ordinary matter (atoms), dark matter, and dark energy. Ordinary matter includes stars, planets, and interstellar gas. Dark matter and dark energy make up most of the universe’s total mass-energy. 6. Daily Motion of Stars Stars appear to move across the sky nightly due to Earth’s rotation. This motion is not caused by the stars themselves but by Earth spinning on its axis. 7. Revolve vs Rotate “Revolve” refers to an object orbiting around another, such as Earth revolving around the Sun. “Rotate” refers to spinning on an axis, like Earth’s daily rotation. 8. Seasons Seasons are caused by Earth’s axial tilt. When one hemisphere tilts toward the Sun, it experiences summer, while the opposite hemisphere experiences winter. Distance from the Sun does not determine seasons. 9. Solstices and Equinoxes Solstices: Occur when the Sun reaches its highest or lowest point in the sky, marking the longest (summer) and shortest (winter) days. Equinoxes: Occur when day and night are of equal length, marking the start of spring and autumn. 10. Phases of the Moon The Moon’s phases result from its orbit around Earth and the changing angles of sunlight hitting its surface. Phases include new moon, crescent, quarter, gibbous, and full moon. 11. Eclipses Solar Eclipse: The Moon blocks the Sun from Earth’s view (Sun-Moon-Earth alignment). Lunar Eclipse: Earth’s shadow falls on the Moon (Sun-Earth-Moon alignment). 12. Trigonometric Parallax Parallax is the apparent shift of a star’s position due to Earth’s orbit. Distance (in parsecs) is calculated using , where is the parallax angle in arcseconds. 13. Tidal Interactions The Moon is tidally locked to Earth, meaning its rotation period matches its orbit, so we always see the same side. Tidal forces also influence Earth’s tides and slow its rotation over time. 14. Kepler’s Three Laws of Planetary Motion 1. Planets orbit the Sun in ellipses, with the Sun at one focus. 2. A line from a planet to the Sun sweeps equal areas in equal times. 3. The square of a planet’s orbital period is proportional to the cube of its average distance from the Sun. 15. Newton’s Three Laws of Motion 1. An object in motion stays in motion unless acted upon by a force. 2. Force equals mass times acceleration (). 3. For every action, there is an equal and opposite reaction. 16. Newton’s Law of Gravity Gravity is the force of attraction between two masses. The force is equal and opposite, but the acceleration depends on the object’s mass (). 17. Light and Matter Interactions Light interacts with matter in the following ways: Emission Absorption Transmission Reflection/scattering 18. Light as an Electromagnetic Wave Light has a constant speed () and its wavelength and frequency are related:. 19. Photons Light is also a particle (photon). Photon energy increases with frequency and decreases with wavelength (). 20. Electromagnetic Spectrum The spectrum includes gamma rays, X-rays, ultraviolet, visible light, infrared, microwaves, and radio waves. Only visible light is detectable by the human eye (400–700 nm). 21. Broad Use of the Spectrum Astronomers study all wavelengths to observe phenomena invisible in the visible spectrum, such as radio emissions or X-ray sources. 22. Temperature and the Kelvin Scale Kelvin measures absolute temperature. It starts at absolute zero (no motion of particles), making it ideal for scientific calculations. 23. Measured Spectra of Light Thermal (black-body): Broad spectrum, continuous intensity curve dependent on temperature. Emission spectrum: Bright lines at specific wavelengths, produced by excited atoms/molecules. Absorption spectrum: Dark lines superimposed on a continuous spectrum, caused by light passing through a cooler gas. 24. Temperature and Black-body Spectrum The temperature of an object determines its black-body spectrum. Hotter objects emit more light at shorter wavelengths (blue) while cooler ones emit more at longer wavelengths (red). Every object with a temperature emits thermal radiation. 25. Object Color and Temperature The color of a hot object indicates its temperature: blue is hotter, red is cooler. This is due to the peak wavelength of the black-body spectrum shifting with temperature. 26. Color of Cold Objects Cold objects reflect light from hotter sources like the Sun. Their color is determined by the wavelengths they reflect most efficiently. 27. Spectra as Chemical Fingerprints Emission and absorption lines are unique to each element and molecule, allowing astronomers to determine composition. This is invaluable for studying distant objects. 28. Doppler Effect The Doppler effect shifts light to shorter wavelengths (blueshift) when an object approaches and to longer wavelengths (redshift) when it recedes. This reveals an object’s motion relative to us. 29. Composition and Power of the Sun The Sun is mostly hydrogen and helium. It is powered by nuclear fusion, where hydrogen nuclei fuse to form helium, releasing energy. 30. Solar System Planets In order: Mercury, Venus, Earth, Mars, Jupiter, Saturn, Uranus, Neptune. 31. Rocky Planets vs Gas Giants Rocky planets: Smaller, solid surfaces (Mercury, Venus, Earth, Mars). Gas giants: Larger, composed of hydrogen, helium, and other gases (Jupiter, Saturn, Uranus, Neptune). 32. Solar System Structure Asteroid Belt: Between Mars and Jupiter; rocky objects. Kuiper Belt: Beyond Neptune; icy bodies, source of short-period comets. Oort Cloud: Spherical shell of icy bodies, source of long-period comets. 33. Dwarf Planets Dwarf planets, like Pluto, are round and orbit the Sun but have not cleared their orbital paths of debris. 34. Star Formation Stars form in nebulae from collapsing clouds of gas and dust. Gravity pulls material inward, increasing temperature and pressure until nuclear fusion ignites. 35. Melting and Boiling Points Melting/boiling points depend on temperature and pressure. In space, low pressures allow materials to transition directly from gas to solid (sublimation). 36. Proto-planetary Disks Formed from rotating nebulae, these disks provide material for planets and stars to form. Dust and gas coalesce under gravity and angular momentum. 37. Planet Formation 1. Dust grains stick together (coagulation). 2. Larger bodies form (planetesimals). 3. Planetesimals collide to form protoplanets. Gas giants require rapid growth to capture hydrogen/helium. 38. Frost Line The frost line marks where volatile compounds (e.g., water, methane) freeze in a disk. Beyond this line, more material is available for planet formation. 39. Birth of a Star A star is born when hydrogen fusion begins. The proto-planetary disk is disrupted by stellar winds and radiation. 40. Moons Moons form via: 1. Accretion from debris (e.g., our Moon). 2. Capture (e.g., Neptune’s Triton). 3. Co-formation alongside planets. Clues about moon origins include orbit and composition. 41. Star Sizes and Types Stars differ in color, temperature, size, and mass. Spectral types range from hot, blue O-types to cool, red M-types. 42. Star Lifespan Larger stars burn fuel faster, resulting in shorter lifespans compared to smaller stars, which can last billions of years. 43. Radioactive Decay Unstable isotopes decay over time, releasing particles. Half-life is the time for half of the isotope to decay, used to date objects. 44. Age of Earth and Solar System Approximately 4.6 billion years old, determined through radiometric dating of meteorites. 45. Density and Planet Composition Density helps infer a planet’s composition: high density suggests metals, while low density suggests gases or ices. 46. Planetary Layers Terrestrial planets have core, mantle, and crust. Differentiation occurs as denser materials sink while lighter materials rise. 47. Seismic Waves Earthquakes produce waves that reveal Earth’s interior structure, such as the liquid outer core and solid inner core. 48. Planetary Cooling Larger planets cool more slowly due to insulating layers. Earth and Venus retain liquid interiors; Mercury and Mars are solidified. 49. Heat Sources Present-day heating comes from radioactive decay within planets. 50. Volcanism Volcanism reshapes surfaces and releases gases. Active volcanism indicates internal heat. Earth, Venus, and Io exhibit active volcanism today. 51. Atmosphere Formation Volcanism and outgassing create atmospheres. Cooling interiors reduce volcanic activity, thinning atmospheres. Earth and Venus retain thick atmospheres due to volcanic activity and magnetic fields. 52. Impact Craters Central peaks in craters form from rebound after impacts. 53. Atmosphere Differences Terrestrial planets have thinner atmospheres from volcanic gases. Gas giants have thicker, hydrogen-rich atmospheres. 54. Greenhouse Effect Atmospheres trap heat. Venus’s thick CO2 atmosphere causes extreme heating, surpassing Mercury’s surface temperature. 55. Past Atmospheres Mars likely had a thicker atmosphere. Losses occur from solar wind, low gravity, and lack of magnetic fields. Earth and Venus retain theirs through magnetic protection. 56. CO2 on Earth Earth’s CO2 is mostly stored in rocks and oceans, unlike Venus and Mars, where it dominates the atmosphere. 57. Erosion Erosion reveals planetary activity, including wind, water, and tectonics, indicating internal heating, atmospheric conditions, and surface dynamics. For example, river valleys show past water flows, while dunes show wind activity. 58. Magnetic Fields and Atmosphere Retention A planet needs a liquid, conducting core and rapid rotation to generate a magnetic field through the dynamo effect. Magnetic fields shield atmospheres from solar wind, protecting against atmospheric erosion. Without it, like Mars, the atmosphere can thin over time. 59. Features of Gas Giants Gas giants are primarily composed of hydrogen and helium, lack solid surfaces, and have strong magnetic fields. Some parts, like their cores, may be solid or liquid metal under immense pressure. 60. Jupiter’s Magnetic Field Despite its small rocky core, Jupiter generates a powerful magnetic field due to metallic hydrogen in its interior, where extreme pressure makes hydrogen conductive. 61. Gas Giant Colors Gas giant colors come from different gases and cloud layers. For example, Jupiter’s and Saturn’s browns and yellows result from ammonia and water clouds, while Uranus and Neptune’s blue 62) Why are Jupiter’s, Uranus’s, and Neptune’s rings less visible than Saturn’s? Ring Visibility: Saturn’s rings are composed of a higher proportion of icy particles that reflect sunlight well, making them much more visible. Jupiter, Uranus, and Neptune have rings primarily composed of darker material such as dust and smaller particles, which are less reflective and thus harder to see. Density and Size: Saturn’s rings are also significantly denser and more extensive, contributing to their striking appearance compared to the faint and narrow rings of the other gas giants. 63) Are gas giant moons more like gas giants or terrestrial planets? What is the main difference in their compositions? Similarity to Terrestrial Planets: Gas giant moons are more like terrestrial planets because they are solid and rocky, unlike the gaseous nature of gas giants. They typically have a core, mantle, and crust structure. Key Difference: Gas giant moons often contain significant amounts of ice in addition to rock, reflecting their formation in colder regions of the Solar System. Terrestrial planets, by contrast, are almost entirely rocky with little to no ice. 64) What additional internal heating source is available to gas giant moons but not terrestrial planets? Tidal Heating: This is the additional heating source. Tidal heating occurs when gravitational forces from a planet (and sometimes other moons) cause the moon’s shape to deform, generating heat through friction within the moon’s interior. Examples of Evidence: The Galilean moons of Jupiter, such as Io (volcanically active), Europa (subsurface ocean), and Ganymede, show clear evidence of tidal heating. 65) Apart from Earth, what are the best places in the Solar System to search for liquid water? Europa (moon of Jupiter): Believed to have a subsurface ocean beneath its icy crust. Enceladus (moon of Saturn): Known for plumes of water vapor, indicating a subsurface ocean. Titan (moon of Saturn): May have liquid water beneath its surface. Ganymede (moon of Jupiter): Potential subsurface ocean. 66) Why were astronomers and planetary scientists excited to observe plumes of water on Europa and Enceladus? Significance of Plumes: Plumes provide direct evidence of liquid water reservoirs beneath the surface. This increases the possibility of finding microbial or other life forms. Accessibility: These plumes allow scientists to sample subsurface materials indirectly without needing to drill through thick ice. 67) What is noteworthy about Titan (moon of Saturn)? Why does it have a methane cycle instead of a water cycle? Noteworthy Features: Titan is the only moon in the Solar System with a thick atmosphere. Its atmosphere is primarily nitrogen, with significant methane and trace hydrocarbons. Methane Cycle: Titan’s surface temperatures are so low (-179°C) that water is frozen solid, and methane exists in liquid and gaseous forms, driving a cycle analogous to Earth’s water cycle. Methane evaporates, forms clouds, rains down, and collects in lakes and rivers. 68) What is an exoplanet? Do we expect them to be common and why? Why are they difficult to observe? Definition: An exoplanet is a planet that orbits a star outside our Solar System. Commonality: Exoplanets are expected to be common based on the observation that most stars host planetary systems. Challenges: Exoplanets are small, faint, and close to their bright host stars, making direct observation difficult. Techniques like detecting their effects on their stars are often used instead. 69) Approximately how many exoplanets have been discovered to date? Number: Almost 6,000 exoplanets have been discovered. 70) Describe the radial velocity and transit methods for detecting exoplanets. Can we determine a planet’s mass or radius from either method? What detection biases are associated with these methods? Radial Velocity Method: Measures the wobble in a star’s motion caused by gravitational interactions with orbiting planets. It provides the planet’s minimum mass. Transit Method: Detects the dimming of a star’s light when a planet passes in front of it. This method provides the planet’s radius. Biases: ○ Radial velocity is better at detecting large planets close to their stars. ○ Transit method is biased toward planets with orbits aligned such that they pass directly in front of their stars. 71) Why does the radial velocity technique work? How can we use the Doppler shift to measure a star’s radial velocity? Mechanism: As a planet orbits, it exerts a gravitational pull on its star, causing the star to move in a small orbit. This motion creates a Doppler shift in the star’s light. Doppler Shift: When the star moves toward us, its light shifts to blue; when it moves away, the light shifts to red. Measuring these shifts allows scientists to calculate the star’s radial velocity. 72) What was surprising about the discovery of 51 Pegasi b? How does planetary migration explain it? Surprise: 51 Pegasi b is a hot Jupiter, a massive gas giant orbiting extremely close to its star, which challenges early theories of planet formation. Planetary Migration: Explains that gas giants can form farther out in cooler regions and then migrate inward due to interactions with the protoplanetary disk or other planets. 73) Are there any exoplanets that have been directly observed? Answer: Yes, a small number of exoplanets have been directly imaged, typically large planets far from their host stars. 74) How can we detect an atmosphere around an exoplanet? Can we learn about its composition? Detection: Observing how starlight interacts with the planet’s atmosphere during a transit (transmission spectroscopy) or by analyzing reflected or emitted light. Composition: Specific wavelengths of light absorbed or emitted by the atmosphere reveal the presence of gases such as water vapor, methane, or oxygen. 75) What is SETI? What is significant about the Voyager space probes? What is the Drake Equation? SETI: The Search for Extraterrestrial Intelligence focuses on detecting signals or evidence of intelligent life beyond Earth. Voyager Space Probes: Carry messages from Earth (golden records) intended for any extraterrestrial life that may encounter them. Drake Equation: An equation estimating the number of active, communicative extraterrestrial civilizations in our galaxy. It considers factors like the rate of star formation, the fraction of stars with planets, and the likelihood of life arising. In-depth Summary of the Topics: 12. Trigonometric Parallax Trigonometric parallax is a method used to measure the distance to nearby stars by observing their apparent shift in position as Earth moves along its orbit. This shift is known as parallax. The distance to a star is inversely proportional to its parallax angle, which is measured in arcseconds. The formula for distance is: d(in parsecs)=1p(in arcseconds)d (\text{in parsecs}) = \frac{1}{p (\text{in arcseconds})}d(in parsecs)=p(in arcseconds)1 Where ppp is the parallax angle. A smaller parallax angle indicates a greater distance. This technique is effective for measuring the distance to stars within a few thousand light-years. 14. Kepler’s Three Laws of Planetary Motion Kepler's laws describe the motion of planets in our solar system: 1. First Law (Ellipses): Planets move in elliptical orbits with the Sun at one focus. 2. Second Law (Equal Areas): A line drawn from a planet to the Sun sweeps equal areas in equal time intervals, meaning planets move faster when closer to the Sun and slower when farther away. 3. Third Law (Orbital Periods): The square of a planet’s orbital period is proportional to the cube of its average distance from the Sun. This law helps calculate the distance of planets based on their orbital period. These laws revolutionized our understanding of planetary motion, replacing the geocentric model with a heliocentric one. 17. Light and Matter Interactions Light interacts with matter in several fundamental ways: 1. Emission: Matter emits light when atoms or molecules transition from a high-energy state to a lower one, releasing photons. 2. Absorption: Matter absorbs light, which increases its energy. The absorbed light can excite electrons to higher energy levels. 3. Transmission: Light passes through matter, which may alter its intensity or direction but not necessarily its composition. 4. Reflection/Scattering: Light bounces off surfaces or scatters in various directions, depending on the surface properties and the light’s wavelength. These interactions are key to understanding phenomena like spectra, temperature, and composition of astronomical objects. 23. Measured Spectra of Light Spectra of light provide valuable information about matter: 1. Thermal (Black-body) Spectrum: A broad spectrum of light emitted by objects based on their temperature. The intensity of this radiation follows a continuous curve, and the peak wavelength depends on temperature (Wien’s Law). 2. Emission Spectrum: Consists of bright lines at specific wavelengths. These lines correspond to specific energies emitted by excited atoms or molecules. 3. Absorption Spectrum: Features dark lines superimposed on a continuous spectrum, where light is absorbed by cooler gases, leaving characteristic gaps in the spectrum. These spectra reveal the chemical composition, temperature, and motion of astronomical objects. 36. Proto-planetary Disks Proto-planetary disks are rotating disks of gas and dust surrounding young stars. These disks are the birthplaces of planets and stars. The material within the disk coalesces under gravity and angular momentum, forming solid bodies (planetesimals) and eventually larger objects like protoplanets. These disks play a crucial role in planet formation. 37. Planet Formation Planet formation begins with small dust grains sticking together (coagulation). These grains then form larger bodies (planetesimals), which collide to form protoplanets. Gas giants require rapid growth to capture large amounts of hydrogen and helium from the surrounding nebula before the gas dissipates. The process is influenced by the solar nebula's temperature, density, and chemical composition. 48. Planetary Cooling Larger planets cool more slowly because their large sizes allow them to retain heat longer, with insulating layers preventing the escape of internal heat. Smaller planets cool more quickly. For example, Earth and Venus maintain liquid interiors, while Mercury and Mars have solidified interiors due to their smaller sizes. 49. Heat Sources The main heat source for most planets today is radioactive decay. This decay of isotopes within a planet’s interior releases energy, which contributes to planetary heating and helps maintain internal processes like volcanic activity and tectonics. 58. Magnetic Fields and Atmosphere Retention To generate a magnetic field, a planet must have a liquid, conducting core and rapid rotation. This process, known as the dynamo effect, creates a magnetic field that shields the atmosphere from the solar wind. Without a magnetic field, as seen with Mars, a planet’s atmosphere can be stripped away over time, causing atmospheric erosion and reducing surface pressure. 64. Tidal Heating in Gas Giant Moons Tidal heating occurs when gravitational forces from a planet or other moons cause a moon’s shape to deform, generating heat due to friction within the moon’s interior. This source of heat is particularly important for moons of gas giants, such as Io (which is volcanically active), Europa (which may have a subsurface ocean), and Ganymede. 65. Best Places to Search for Liquid Water in the Solar System Apart from Earth, the best places to search for liquid water are: Europa (moon of Jupiter): A subsurface ocean beneath its icy crust. Enceladus (moon of Saturn): Plumes of water vapor suggest a subsurface ocean. Titan (moon of Saturn): Possible liquid water beneath the surface. Ganymede (moon of Jupiter): Potential subsurface ocean. These moons are considered the most likely places to find conditions suitable for life outside Earth. 66. Significance of Plumes on Europa and Enceladus The discovery of plumes of water on Europa and Enceladus is significant because they provide direct evidence of liquid water beneath the icy surface, increasing the possibility of microbial life. These plumes also allow for indirect sampling of subsurface materials, facilitating exploration without the need to drill through thick ice. 67. Titan’s Methane Cycle Titan, Saturn’s largest moon, is unique for having a thick nitrogen-rich atmosphere and a methane cycle instead of a water cycle. Due to extremely cold surface temperatures (-179°C), water is frozen, and methane exists in liquid and gaseous forms. Titan’s methane cycle resembles Earth’s water cycle, with methane evaporating, forming clouds, raining down, and collecting in lakes and rivers. 68. Exoplanets An exoplanet is a planet that orbits a star outside our Solar System. Exoplanets are expected to be common because most stars have planetary systems. However, exoplanets are difficult to observe directly because they are small, faint, and close to their bright host stars. Astronomers detect exoplanets by studying the effects they have on their parent stars, such as slight wobbles or dimming during transits. 69. Number of Discovered Exoplanets Nearly 6,000 exoplanets have been discovered to date, with many more expected as observational techniques improve. 70. Radial Velocity and Transit Methods for Detecting Exoplanets Radial Velocity Method: Measures the wobble of a star caused by the gravitational pull of an orbiting planet. It provides the minimum mass of the planet. Transit Method: Detects the dimming of a star’s light when a planet passes in front of it. This method helps determine the planet’s radius. Biases: The radial velocity method is more sensitive to large planets that are close to their stars. The transit method is biased toward detecting planets with orbits aligned to pass directly in front of their stars. 71. Radial Velocity Technique and Doppler Shift The radial velocity technique works by detecting the slight wobble of a star due to the gravitational pull of an orbiting planet. This motion causes a Doppler shift in the star’s light: blue when the star moves toward us, and red when it moves away. These shifts allow astronomers to measure the star’s radial velocity and infer the presence of a planet. 72. 51 Pegasi b and Planetary Migration The discovery of 51 Pegasi b, a hot Jupiter, was surprising because it challenged early theories of planet formation, which suggested gas giants should form far from their stars. Planetary migration explains this by suggesting that gas giants can form at greater distances and then move inward through interactions with the protoplanetary disk or other planets. 4o mini