Lectures on Aromatic & Heterocyclic Compounds PDF
Document Details
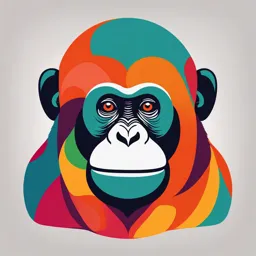
Uploaded by OverjoyedIron9589
Port Said University
Dr. Mohamed Abd El-Moneim
Tags
Summary
This document is a set of lectures on aromatic and heterocyclic compounds. It covers the nomenclature, structures, and reactions of various compounds, including benzene, its derivatives, and different types of heterocyclic rings. The lectures are intended for an undergraduate-level organic chemistry course at Port Said University.
Full Transcript
Port Said University Faculty of Science Chemistry Department Lectures on Aromatic Compounds & Heterocyclic compounds Prepared by: Dr. Mohamed Abd El-Moneim Associated professor - Organic Chemistry...
Port Said University Faculty of Science Chemistry Department Lectures on Aromatic Compounds & Heterocyclic compounds Prepared by: Dr. Mohamed Abd El-Moneim Associated professor - Organic Chemistry 1 Contents Part 1: Aromatic Compounds Introduction …………………………………..……………………………………………………………………...…………… 3 Chapter I: Benzene……………………………………………………………………………………………………………. 4 Chapter II: Alkyl Benzene (Arenes)……………………………………………………………………………………,,,, 35 Chapter III: Halogen Derivatives of Aromatic Hydrocarbons …………………………………………………41 Chapter IV: Aromatic Sulphonic Acids ……………………………………………………………………………….. 48 Chapter V: Aromatic Nitro-Compounds ………………………………………………………………………………56 Chapter VI: Aromatic Amines ……………………………………………………………………………………………. 62 Chapter VII: Aromatic Diazonium Salts ……………………………………………………………………………… 74 Chapter VIII: Phenols and Aromatic Alcohols……………………………………………………………………… 83 Chapter IX: Aromatic Aldehydes and Ketones …………………………………………………………………….. 95 Chapter IV: Aromatic Carboxylic Acids and their Derivatives ……………………………………………… 106 Part 2: Heterocyclic Compounds Chapter I: Nomenclature of heterocyclic compounds……………………………………………………………119 Chapter II: Five-membered heteroaromatic rings ……………………………………………………………… 132 Pyrrole ……………………………………………………………………………………………………………………………132 Furan ………………………………………………………………………………………………………………………………142 Thiophene ………………………………………………………………………………………………………………………147 Chapter III: Six-membered heteroaromatic rings ……………………………………………………………… 150 Pyridine ……………………………………………………………………………………………………………………………150 Chapter IV: Fused bicyclic rings …………………………………………………………………………………………160 Indole ………………………………………………………………………………………………………………………………160 Quinoline …………………………………………………………………………………………………………………………164 Isoquinoline ……………………………………………………………………………………………………………………168 2 Aromatic Compounds Introduction: The word “aromatic” means the pleasant odor of freshly prepared coffee, or of a cinnamon bun. In the history of organic chemistry, pleasantly compounds were isolated from natural oils produced by plants called “aromatic compounds” like: As time passed, chemists found or synthesized many compounds with benzene rings that had no odor, such as benzoic acid and acetylsalicylic acid (aspirin). 3 Structure of benzene Michael Faraday was the first who established the molecular formula of benzene to be C6H6. Comparison of such formula with the fully saturated analogue C6H12 (cyclo Hexane) indicated that benzene is highly unsaturated. Benzene is totally different from unsaturated aliphatic compounds (olefins), benzene does not react with most of the reagents that add to olefins; it undergoes substitution reaction. 1) Action of KMnO4: Addition H2C CH2 H2 C CH2 + Alk. KMnO4 reaction OH OH C6H6 + Alk. KMnO4 No Reaction 2) Action of bromine: Br Br H2C CH2 + Br2 H2C CH2 addition No reaction occure (C6H6Br6 not formed) C6H6 + 3 Br2 reaction Br Substitution + Br2/FeBr3 Reaction bromobenzene Kekulé structure Kekulé was the first who proposed a cyclic structure for benzene with three double bonds. Such formula can explain the following: a- All six hydrogens are equivalent. 4 H H H H H H b- The existence of only one possible monosubstituted benzene derivative. X c- The existence of three possible disubstituted benzene derivatives. X X X X X X o- (ortho) m- (meta) p- (para) Each substituted derivative has an isomer that is different only in the location of double bonds. H H H H H H H H H H H H I II Kekulé modified his theory, he suggested that the double bonds are so rapid shifted, and isomers in such rapid and mobile equilibrium can never be separated. or 5 Bond length: Each bond spends half the time as double and half its time as a single bond, so all the C-C bond lengths in benzene are between single and double bonds 1.39 Ao. C C C C C C C C C 1.54 Ao 1.34 Ao C 1.39 Ao According to the Molecular Orbital Theory: The six carbon atoms of benzene are arranged in a regular planar hexagone. The carbons are sp2 hybridized, the bond angles between them is 120o. Each carbon is attached to a hydrogen atom through a σ bond. H H H H H H The 6p aromatic orbitals are all parallel to each other and perpendicular to the plane of the ring. Overlapping of these atomic orbitals can take place either 1,2 - 3,4 - 5,6 (structure A) or 1,6 – 2,3 – 4,5 (structure B) giving rise to the two kekulé structures (structure A & B). 6 1 2 6 3 5 4 (A) 1 2 6 3 5 4 (B) Each p atomic orbital can also overlap in both directions (structure c). This results in delocalization of the 6p electrons over the whole system. As a result a cloud of negative charge is formed above and below the plane of the ring (structure d). written as (c) (d) Benzene and aromatic compounds react by substitution rather than by addition because addition prevents such delocalization and breaks the aromaticity of the ring. From thermodynamics: Cyclohexene is a six-membered ring containing one double bond, can be hydrogenated easily to cyclohexane and the reaction is exothermic. When the ΔH° (Heat of hydrogenation) for this reaction is measured, it is found to be -120 kJ mol-1, very much like that of any similarly substituted alkene: 7 We would expect that hydrogenation of 1,3-cyclohexadiene would liberate roughly twice as much heat and thus have a ΔH° equal to about -240 kJ mol-1. When this experiment is done, the result is ΔH°-232 kJ mol-1. Benzene is simply 1,3,5-cyclohexatriene, we would predict benzene to liberate approximately 360 kJ mol-1 [3 x (-120)] when it is hydrogenated. When the experiment is actually done, the result is surprisingly different. The reaction is exothermic, but only by 208 kJ mol-1. From these results, it becomes clear that benzene is much more stable than the hypothetical 1,3,5-cyclohexatriene by 152 kJ mol-1. This difference can 8 be explained by taking into account the fact that “compounds containing conjugated double bonds are usually somewhat more stable than those that contain Isolated double bonds”. This difference between the amount of heat actually released and that calculated on the basis of the Kekulé structure is now called the resonance energy of the compound. So, benzene undergo substitution reactions, and could not undergo addition reactions. Characteristics of Aromatic Compounds: i) Cyclic structure, contributing atoms arranged in one or more rings. ii) Coplanar structure, with all the contributing atoms in the same plane. iii) Conjugated double bonds, a delocalized conjugated π system, most commonly an arrangement of alternating single and double bond. iv) Obeys Huckel’s rule, the number of π delocalized electrons is equals to 4n + 2, where n is an integer number ( n = 1, 2, 3……. etc ). Examples: Benzene Naphthalene 6 es 10 es 4n+2=6 4 n + 2 = 10 4n=4 4n=8 n=1 n=2 Anthracene 14 es 4 n + 2 = 14 n=3 9 Heterocyclic rings:...... N O S N...... H Pyrrole Furan Thiophene Pyridine 4n + 2 = 6 n =1 The hetero atom in the case of 5-membered rings provides 2é for the aromatic ring, while in the case of the six-membered ring (pyridine) only one p electron is provided from the hetero atom for the aromatic sextet. 6 π es 4 π es 4n+2= 6 4n+2= 4 n=1 n= ½ Aromatic Antiaromatic Non benzenoid Aromatics, these are conjugated cyclic systems (monocyclic or polycyclic) having (4n + 2) electrons and not benzoic compounds. Cycloctatetraene 8 es 4n+2=8 n = 3/2 Cycloctatetraene is non-aromatic compound; it is not obey Huckel’s rule since their atoms are not lying in the same plane. 11 Nomenclature of Benzene Derivatives I) Monosubstituted benzenes: Since all the six hydrogen atoms in benzene are equivalents only one monosubstituted isomer is possible. The monosubstituted compound is usually named as derivative of benzene unless it has a trivial name (common name). The IUPAC name: Monosubstituted benzenes are systematically named in the same manner as other hydrocarbons, with -benzene as the parent name. Thus, C6H5Br is bromobenzene, C6H5NO2 is nitrobenzene, and C6H5CH2CH3 is ethylbenzene. The name phenyl, abbreviated as Ph- is used for the C6H5 unit when the benzene ring is considered as a substituent. In addition, a generalized aromatic substituent is called an aryl group, abbreviated as Ar-, and the name benzyl is used for the C6H5CH2- group. CH3 CH2CH3 Benzene Methylbenzene Ethylbenzene H3C CH3 CH2CH2CH3 CH SO3H NO2 n-Propyl benzene Isopropyl benzene Benzene sulphonic acid Nitrobenzene 11 Common names CH3 CHO COCH3 OH NH2 Toluene Benzaldehyde Acetophenone Phenol Aniline Disubstituted benzenes: Disubstituted benzenes are named using one of the prefixes ortho- (o), meta- (m), or para- (p). An ortho-disubstituted benzene has its two substituents in a 1,2-relationship on the ring; a meta-disubstituted benzene has its two substituents in a 1,3-relationship; and a para-disubstituted benzene has its substituents in a 1,4-relationship. CH3 CH3 CH3 CH3 CH3 CH3 1,2-dimethyl benzene 1,3-dimethyl benzene 1,4-dimethyl benzene o-Xylene m – Xylene p-Xylene Benzenes with more than two substituents Are named by choosing a point of attachment as carbon 1 and numbering the substituents on the ring so that the second substituent has as low a number as possible. The following rules are applied for nomenclature: 1. Number 1 is given to the principal function group which is usually chosen according to the following order. COOH > SO3H > CHO > CN > C = O > OH > NH2 2. The functional group which is given number (1) is named as suffix. 12 3. Other groups are named as prefixes and are arranged in alphabetical order. 4. The ring is oriented with position No. 1 at the top and proceeds numbering in a clockwise manner. COOH OH CH3 CH3 NO2 Br OH I NH2 3-bromo-4-hydroxy-benzoic acid 4-iodo-2-methyl phenol 4-Amino-2-nitrotoluene Aromatic Electrophilic Substitution in Benzene The most common reaction of aromatic compounds is electrophilic aromatic substitution, a process in which an electrophile (E+) reacts with an aromatic ring and substitutes for one of the hydrogens. Many different substituents can be introduced into the aromatic ring by electrophilic substitution. An aromatic ring can be substituted by a halogen 13 (-Cl, -Br, -I), a nitro group (-NO2), a sulfonic acid group (-SO3H), an alkyl group (-R), or an acyl group (-COR). General mechanism: This involves firstly the formation of the so called complex, in which no true bond is formed, but there is only electrostatic attraction between the system of the benzene and the positive end of the electrophile E. The complex is converted slowly to the σ complex which is characterized by the following: 1. There is true bond formation. 2. It has no longer aromatic structure. It is carbocation with 4 p electrons delocalized over 5 carbon atoms, and one C with sp3 hybridization (tetrahedral). 3. The attacking and leaving groups are present in a plane perpendicular to the ring. 4. Formation of σ complex for most electrophilic substitution reactions is the slow step as it requires high energy to break the aromaticity of the ring. 5. Energy is given to attain the transition state 1 which is formed by the approach of electrophile (E+) to benzene, and then the σ complex or the 14 cationic intermediate is formed. This loses rapidly a proton after passing by the transition state 2. H E H E H E + + + E+ + slow E + Transition state 1 (s complex) (p complex) + E E Hd H E - H+ + + H + fast + Final product Transition state 2 1) Nitration : Nitration is the introduction of nitro group (NO2) in benzene. It is usually performed by conc. HNO3 and conc. H2SO4 (nitrating mixture). The actual nitrating agent is the strong electrophile nitronium cation +NO2. The role of conc. H2SO4 is the production of the nitronium cation by its reaction with conc. HNO3 as follows: O O O O.. H+ + -H2O ++ + OH N + H O N+ N N O- O- O H O- Nitronium Ion + H2O + H2SO4 H3O + HSO4- Hydronium ion bisulphate anion 15 H NO2 H NO2 H NO2 + + + NO2 slow + + complex) + NO2 NO2 H H NO2 H+ + - H+ + + fast Nitrobenzene 2) Sulphonation: It is the introduction of sulphonic group (-SO3H) into aromatic rings. The most commonly used sulphonating agent is fuming sulphuric acid (conc. H2SO4 saturated with SO3). The actual sulphonating agent is SO3. The electrophilic center is the sulfur atom which has a partial positive change: The reaction of benzene with fuming sulfuric acid occurs by the usual two- step mechanism. An electrophile first adds to the aromatic ring, and H is then lost. In sulfonation reactions, the electrophile is HSO3. 16 - + O H S O SO3- SO3H O- O - H+ + H+ + +S O + O Benzene sulphonic acid 3) Halogenation: By using Halogen and Lewis acid (catalyst) at low temperature and in absence of light, e.g.; Br2/FeBr3, Cl2/FeCl3 or Fe/X2. The electrophile is the chloronium Cl+ or bromonium Br+ cation. Br Absence of light + HBr + FeBr3 + Br2 + FeBr3 Mechanism: Br Br + FeBr3 + + - Br FeBr4 Br H Br slow -H+ + Br+ + + HBr + FeBr3 fast -complex 4) Friedel Crafts Reactions: There are Friedel Crafts alkylation and Friedel Crafts acylation reactions. a) Friedel Crafts alkylation: This is used for the synthesis of alkyl benzene from benzene. The reaction is carried out by treating the aromatic compound with an alkyl halide, RCl or 17 RBr, in the presence of AlCl3 or AlBr3 to generate a carbocation electrophile, R+. Aluminum chloride catalyzes the reaction by helping the alkyl halide to dissociate in much the same way that FeBr3 catalyzes aromatic brominations by helping Br2 dissociate. Loss of H then completes the reaction. CH3 AlCl3/80oC + CH3Br + HBr Benzene Methylbromide Toluene Mechanism: Drawbacks of Friedel Crafts Alkylation i) Polyalkylation: This occurs because the produced alkyl benzene, such as toluene, is more reactive towards electrophilic substitution than the starting material benzene, because of the electron donating effect of the alkyl group (Inductive effect and hyperconjugation). 18 R R AlCl3 RX + RX R AlCl3 The use of small amount of Lewis acid and large amount of the starting material may help to minimize polysubstitution. ii) Isomerisation: For the stability of the carbonium ion (obtained from the alkyl halide) rearrangement to the more stable form and this leads to unexpected isomeric product, example: CH3 AlCl3 + CH3CH2CH2Cl CH CH3 n-Propylbromide Isopropylbenzene (Cumene) Mechanism: AlCl3 + - CH3 CH2 CH2 Cl CH3CH2CH2 Cl.... AlCl3 + H3C + hydride shift CH3 CH CH2 CH H3C H n-propyl carbocation Isopropyl carbocation (primary carbocation) (secondary carbocation) more stable H3C CH3 H3C CH3 CH CH H H3C + slow + -H+ CH + fast H3C s-complex Cumene 19 b) Friedel Crafts acylation: This involves the introduction of an acyl group (RCO-) into an aromatic ring. The reaction is performed by the action of acid chloride, acid anhydride or ester on the aromatic compound in the presence of Lewis acid. The actual acylating agent here is the acyluim cation RCO+ which is generated during the reaction from acid chloride as follows: O + - R - CO - Cl + AlCl3 R-C Cl.... AlCl3 R- C=O + AlCl4 acid chloride acylium ion O H R C H R C O AlCl4 + R C O + AlCl3 + HCl Unlike alkylation, Friedel-Crafts acylation is easily controlled to produce monosubstituted derivatives; because once an acyl group is introduced in a benzene nucleus it is impossible to introduce another acyl group since the acyl group is an electron withdrawing group that deactivates the ring towards further substitution. Introduction of long normal alkyl chain into benzene cannot be obtained by Friedel Crafts alkylation. However, it can be done by Friedel Crafts acylation followed by reduction of the C=O group either by Clemensen's or Wolff-Kishner reduction. O C CH2CH2CH3 (CH2)3CH3 Zn/Hg/HCl Clemensen's + CH3CH2CH2COCl AlCl3 NH2NH2/KOH Phenyl propyl Wolff Kishner n-butylbenzene ketone 21 Substituents Effect in Electrophilic Aromatic substitution Only one product can form when an electrophilic substitution occurs on benzene, but what would happen if we were to carry out an electrophilic substitution on a ring that already has a substituent? A substituent already present on the ring has two effects: Substituents affect the reactivity of an aromatic ring and substituents affect the orientation of a reaction. 1- Substituents affect the reactivity of an aromatic ring: Some substituents activate a ring, making it more reactive than benzene, and some deactivate a ring, making it less reactive than benzene. In aromatic nitration, the presence of an -OH substituent makes the ring 1000 times more reactive than benzene, while an -NO2 substituent makes the ring more than 10 million times less reactive. 2- Substituents affect the orientation of a reaction: When one group is introduced into the benzene ring, only one monosubstituted product is obtained. When a second group is introduced, three isomeric products are possible: ortho-, meta-, and para- are usually not formed in equal amounts. It was found that the position of the incoming group depends on the nature of the group already present (A). A A A A B B B o- 21 m- p- Substituents can be classified into three groups: i) ortho and para-directing activators ii) meta-directing deactivators iii) ortho- and para-directing deactivators The directing effect of a group correlates with its reactivity. All meta directing groups are deactivating, and all ortho- and para-directing groups other than halogen are activating. There are no meta-directing activators. The halogens are unique in being ortho and para-directing but deactivating. Ortho and para-directing Meta-directing Ortho and para- activators deactivators directing deactivators -OH, -OR -NO2 -F, -Cl, -Br, -I -NH2, -NHR, -NR2 -SO3H -C6H5 -COOH, -COOR -CH3, -R (alkyl) -CHO, -COR, -CN Activating and Deactivating Effects in Aromatic Rings Activating groups are donate electrons to the ring, thereby making the ring more electron-rich, stabilizing the carbocation intermediate, and lowering the activation energy for its formation. Deactivating groups are withdraw electrons from the ring, thereby making the ring more electron-poor, destabilizing the carbocation intermediate, and raising the activation energy for its formation. For example: we can predict which would react faster in an electrophilic aromatic substitution reaction, chlorobenzene or ethylbenzene? 22 Cl CH2CH3 chlorobenzene ethylbenzene By comparing the relative reactivity of chloro and alkyl groups, the chloro substituent is deactivating, whereas an alkyl group is activating. Thus, ethylbenzene is more reactive than chlorobenzene. An -OH group directs further substitution toward the ortho- and para-positions, while a -CN group directs further substitution primarily toward the meta-position. 2- Orientation and Relative reactivity in Electrophilic Aromatic Substitution Reactions The orientation and reactivity of different groups can be explained in the basis of inductive and resonance effects on the stability of σ-complex intermediates. i) Inductive effect: Inductive effect is the withdrawal or donation of electrons through a bond due to an electronegativity difference between the ring and the attached substituent atom. Alkyl groups are electron-donating and so will increase 23 the electron availability over the benzene ring. The effect in toluene is due to hyperconjugation. H H H H H H C H H C H H C H H C H H C H CH3 The inductive effect of most of other substituents e.g. halogens, -OH, -OCH3, -NH2, -SO3H, will be in the opposite direction, as the atom attached to the ring is more electronegative than the carbon atom to which it is attached. Cl OCH3 ii) Resonance effect: A number of common substituents have unshared electron pairs on the atom attached to the benzene ring, and these can interact with the delocalized π-orbitals of the ring [donation (+R effect). OCH3 OCH3 OCH3 OCH3 OCH3 24 The same effect (+R) applies to –OH, -NH2, -SH, -NHCOCH3, and halogens (-X). The effect in the opposite direction (-R effect) can take place if the atom attached to the benzene ring is bonded to another more electronegative atom by a multiple bond. The interaction with the π-orbitals of the ring takes place as follows: H H H H H C O C O C O C O C O The same consideration applies to –COR, -NO2, -COOH, -SO3H and –CN. Here, the electron availability over the ring is decreased. ii) The overall effect: Electron-donating group lead to more rapid substitution by electrophilic reagents than benzene itself, since the electron density on the ring becomes higher. Such group is considered to be an activating group. On the other hand, electron-withdrawing group lead to less rapid substitution than benzene and is considered to be deactivating group. For example, toluene is more reactive towards nitration than benzene, whereas nitrobenzene is less reactive than benzene towards this electrophilic substitution reaction. For a group like –NO2, both inductive and resonance effects act in the same direction (-I, -R) and the overall is electron-withdrawing. But with groups like (-OH, -NH2, -OCH3 and halogens), the two effects act in opposite directions (-I, +R). -OH, -NH2, -NHR A ( A is activating) -OR, -NHCOCH3 and -R -CHO, -CN, -COOH A (A is deactivating) -COOR, -COR, SO3H, 25 and -halogens iv) Stability of σ-complex intermediates: Electrophile can attack all the available positions of an aromatic ring, but reactions at the more favored sites are more rapid than those at the less active positions. The σ-complex having the lowest energy (more stable) is associated with the more favored position for attack. Ortho- and Para-Directors in Aromatic Rings For example, the carbocation intermediates in nitration of phenol: Because the ortho and para intermediates are more stable than the meta intermediate, they are formed faster. In general, any substituent that has a lone pair of electrons on the atom directly bonded to the aromatic ring allows an electron-donating resonance interaction to occur and thus acts as an ortho and para director. 26 Meta Directors in Aromatic Rings The influence of meta-directing substituents can be explained by using the nitration of benzaldehyde. Of the three possible carbocation intermediates, the meta-intermediate has three favorable resonance forms, while the ortho and para intermediates have only two. In both ortho and para intermediates, the third resonance form is particularly unfavorable because it places the positive charge directly on the carbon that bears the aldehyde group, where it is disfavored 27 by a repulsive interaction with the positively polarized carbon atom of the CO group. Hence, the meta-intermediate is more favored and is formed faster than the ortho- and para-intermediates. In general, any substituent that has a positively polarized atom (δ+) directly attached to the ring makes one of the resonance forms of the ortho- and para-intermediates unfavorable, and thus acts as a meta-director. The behavior of halobenzenes (e.g.chlorobenzene ): Although Cl is a deactivating group (-I > +R effect), it does substitute ortho-para. Cl Cl Cl Cl Cl H H H H Ortho-attack NO2 NO2 NO2 NO2 Most stable Cl Cl Cl Cl Cl Para-attack H NO2 NO2 NO2 H NO2 H H Most stable Cl Cl Cl Cl Meta-attack H H H NO2 NO2 NO2 28 It is clear that the chlorine atom stabilizes the σ-complexes resulting from ortho and para-attack. It does this as –OH and -NH2 groups by donating a pair of nonbonding electrons. These nonbonding electrons give rise to exceptionally stable resonance structures in the hybrids for ortho and para σ-complexes. The following figure summarizes the substituents effect in electrophilic substitution reactions. Orientation in disubstituted benzene derivatives i) If the directing effects of the two groups reinforce each other: It leads to a single product. The entering substituent is directed by both groups into the position indicated by arrows. (o, p-orienting group) CH3 CH3 NO2 + NO2 (m-orienting group) NO2 NO2 4-nitrotoluene 2,4-dinitrotoluene ii) When the directing effects of the two groups opposes each other: Electrophilic substitution occurs according to the more electron donating groups. NH2 > OR > OH > R 29 OH OH CH3 NH2 N is less electronegative than O OH NH2 CH3 Cl CH3 Cl NH2 Br (Br is less electronegative than Cl) CH3 (o, o, p) H3C CH3 (all the three positions are equivalent and are more reactive than toluene) OH OH OH COOH NO2 SO3H NO2 NO2 31 iii) Substitution between meta substituents does not occur because this site is too hindered: Cl Cl Br Br Nitration: 62% 37% iv) If the two groups are deactivating: O2N HNO3 NO2 NO Reaction H2SO4 m-dinitrobenzene But in case of presence of an activating group like -CH3 or -OH, the third nitration is possible. v) If there are 2 benzene rings (biphenyl): The second substituent will enter in the unsubstituted benzene ring. It will favor the p-position due to the steric hindrance in the o-position, independently of the group found on the substituted ring. R 31 Preparation of Benzene A- Industrial Methods: 1- From Distillation of Coal tar: When heated at 1000-1300 oC in coke oven in absence of air, it gives gases such as H2, CH4, CO, ammonia, light tar and coke. The light oil (80-170oC) can be fractionated into benzene (60%), toluene (14%), mixed xylene (7%) and other liquid products. Light oil Medium Oil Heavy Oil Anthracene Oil b.p. (80-170oC) b.p. (170-230oC) b.p. (230-270oC) b.p. (270-360oC) Benzene, Naphthaline, Alkyl benzene, Anthracene, Toluene, Phenols, Pyridine. Cresol, Phenanthrene, Xylene Quinoline, Florine, Naphthaline Isolated Isoquinoline, Carbazole, isolated by according to Indole Acridine freezing, and their boiling pyridine by using points H2SO4 2-From Petroleum: Petroleum contains few aromatic compounds and consists of largely alkanes. Arenes can be obtained from certain petroleum fractions (C6-C8) by Dehydrogenated cyclization. The n-parafines are probably first dehydrogenated to olefins and then cyclized to benzene and its homologues. CH3 CH2 H2C CH3 HC CH2 Cr2O3 / Al2O3 - H2 H2C CH2 - 3 H2 HC CH C C H2 H CH3 CH3 CH2 CH CH3 H2C CH3 HC CH2 Cr2O3 / Al2O3 - H2 H2C CH2 - 3 H2 HC CH C C H2 H 32 b- Laboratory Methods: 1- From acetylene: by heating of acetylene in hot tube at 500 oC. CH HC CH 500oC HC CH CH 2- From Benzene sulphonic acid: by distillation with superheated steam SO3H H2O distillation + 3- From phenol: by passing its vapour over zinc dust. OH Zn distillation + ZnO + 4- From sodium benzoat: by heating with sodalime (decarboxylation). COONa Sodalime, + Na2CO3 (CaO+NaOH) 5- From benzenediazonium chloride: by heating of benzenediazonium chloride with hypophosphoric acid in the presence of cuprous salt. N2+ -Cl H3PO2 Cu(1+) 33 Physical properties of benzene Benzene is colorless liquid with a characteristic odor its boiling point is 80.01 oC. It burns with a smoky flame. Benzene is miscible in all proportions with most organic solvents. Water is soluble in benzene to the extent of (1%). Chemical Reactions of benzene A- Addition reactions: 1- Catalytic hydrogenation of benzene give cyclohexane: 3H2 / Pt 2- Addition of halogens: in presence of sun light and in absence of catalyst it gives benzenehexahalides. Cl hv Cl Cl + 3Cl2 Cl Cl Cl 3- Ozonolysis of benzene: It gives triozonide which can be hydrolyzed to glyoxal. O O H2O HC O + 3 O3 3 HC O O glyoxal The following diagram summarizes some important preparations and reactions of benzene: 34 N2Cl CH3 CH2Cl NO2 H3PO2 CH2O /HCl Cu+1 ZnCl2 CHO CH3Cl AlCl3 conc. HNO3/ CO / HCl H2SO4 OH AlCl3-CuCl COOH Zn dust Sodalime [CaO/NaOH] 400-500oC CH3CH2Br AlCl3 3 CH CH SO3 CH2CH3 CH3COCl H2SO4 AlCl3 Br2 /Fe SO3H COCH3 Br 35 Alkyl Benzene (Arenes) These compounds having one or more alkyl group attached to the aromatic nucleus. The simplest of the alkylbenzenes is Toluene. Compounds containing longer side-chains are named by prefixing the name of the alkyl group to the word- benzene. e.g. Ethylbenzene, n-propylbenzene and isobutylbenzene. Isobutylbenzene n-propylbenzene Ethylbenzene Toluene CH3 CH2CH2CH3 CH2CH3 CH3 CH2CHCH3 Industrial Importance of Arenes: Some arenes e.g. benzene, ethylbenzene, xylene, isopropylbenzene, etc. are used for manufacturing petrochemicals. 1- Benzene: Benzene may be regarded as the parent compound of nearly all aromatics. In petroleum refining processes, there is more Toluene produced which is converted to benzene by hydroalkynation. Toluene is mixed with hydrogen and passed over a platinum catalyst on Al2O3 at 600 oC under pressure. CH3 Al2O3 / Pt + H2 600 oC, pressure 2- Isopropylbenzene (cumene): This compound is used for the manufacture of phenol and acetone. Phenol is used to make a variety of resins and plastics. 36 CH3 H3PO4 Isopropylbenzene + CH3CH CH2 CH catalyst CH3 CH3 H H3C C CH3 H3C C OOH OH O O2 / 100oC aq. H2SO4 + CH3 C CH3 6 atm. 100oC 1-(2-hydroperoxypropan-2-yl)benzene phenol Acetone 3- Poly (phenylethene): This is known as polystyrene. The monomer of this polymer, styrene, is prepared from benzene as follow: H2C CH3 HC CH2 AlCl3 / HCl ZnO + H2C CH2 o 85 C 600oC Ethylbenzene Styrene Methods of preparation of Alkyl Benzene 1- From Wurtz- Wittig synthesis: by warming an ethereal solution of alkyl and aryl halides with sodium metal. Br CH3 2 Na + CH3Br + 2NaBr ether Toluene Br CH2(CH2)2CH3 2 Na/ + CH3(CH2)2CH2Br + 2NaBr ether 37 2- From Friedel-Craft’s alkylation of benzene: CH3 AlCl3 + H3C Cl + HCl 3- From Grignard’s reaction: by the action of an alkyl halide on phenyl magnesium bromide. Br MgBr CH3 Mg / ether CH3Br + MgBr2 4- Fia Clemensen reduction: COCH3 CH2CH3 Zn / Hg / HCl + H2O Acetophenone Chemical Reactions of alkylbenzenes The reactions of alkyl benzenes can be divided into two categories: a) Those involving the benzene ring b) Those involving the aliphatic side-chain a) Electrophilic substitution in the aromatic ring Like benzene, alkylbenzenes undergo electrophilic substitution reactions. Because of its electron-donating effect, an alkyl group activates the benzene ring to which it is attached and directs substitution to the ortho and para positions. 38 1- Nitration: By the action of conc. HNO3 /H2SO4 give a mixture from o-nitro alkylbenzene and p-nitro alkylbenzene. CH3 CH3 CH3 NO2 Conc. HNO3 + H2SO4 NO2 2- Sulphonation: By the action of conc. H2SO4 / SO3 give a mixture from o- and p- alkylbenzene sulphonic acids. CH3 CH3 CH3 SO3H SO3 + H2SO4 SO3H 3- Halogenation: When halogenation is carried out in absence of sunlight in the presence of catalyst, halogenation takes place in benzene ring giving a mixture from o- and p-haloalkylbenzene (i.e. electrophilic substitution reaction). CH3 CH3 CH3 X2 X + AlCl3 X=Cl, Br X 4- Alkylation: CH3X CH3 CH3 + H3C CH3 AlX3 X=Cl, Br CH3 39 p-Xylene o-Xylene b) Reactions of the side-chain: 1- Halogenation: If the halogenation is carried out in the presence of sunlight and in absence of catalyst, the halogenations takes place at the side chain (i.e. free radical substitution reaction). CH3 CH2Cl CHCl2 CCl3 Cl2 Cl2 Cl2 hv hv hv Toluene benzyl chloride benzal chloride benzo trichloride NaOH NaOH NaOH CH2OH CHO COOH benzyl alcohol benzaldehyde benzoic acid 2- Oxidation: Oxidation to benzoic acid can be carried out by heating toluene with dilute nitric acid in a sealed tube, by the action of chromic acid or by the action of alkaline potassium permanganate. CH3 COOH KMnO4 / OH-, heat However, oxidation with MnO2 (conc. H2SO4) gives benzaldehyde. CH3 CHO + 2MnO2 + 2H2SO4 + 3H2O + 2MnSO4 41 Catalytic air oxidation of ethylbenzene gives acetophenone. H2C CH3 COCH3 Mn(OAc)2 + O2 130 oC, 50 psi 41 Aromatic halogenated compounds There are two types of halogen derivatives; Aryl halides and Aralkyl halides. Aryl halides: They are compounds in which the halogen atoms are attached directly to an aromatic nucleus. They have the general formula [Ar-X]. Bromobenzene p-Chlorotoluene p-Bromostyrene Br CH3 HC CH2 Cl Br Methods of Preparation 1- Direct Halogenation: (Cl2, Br2) react with benzene or alkyl benzene in presence of a catalyst such as FeCl3 or AlCl3 and in absence of sun light to give substitution products in benzene ring. Br Cl Br2 Cl2 HBr + + HCl FeBr3 AlCl3 2- Substitution of hydroxyl group in phenol with Halogen atom: OH Br + PBr5 + POBr3 + HBr Phosphorous penta bromide 42 3- Sandmeyer reaction: Replacement of diazonium group by –Cl or –Br is carried out by mixing a solution of freshly prepared diazonium salt with cuprous chloride or cuprous bromide. Ar-N2X + CuX Ar-X + N2 Gattermann reaction: Modification of Sandmeyer reaction was achieved by Gattermann, in which the copper powder and hydrogen halide are used instead of cuprous halide. Replacement of diazonium group with iodide is carried by mixing the diazonium salt directly with potassium iodide. CH3 BF4 Cu F CH3 CH3 CH3 Cu/HCl NaNO2 HCl Cl NH2 CH3 N2Cl Cu/HBr Br CH3 KI I 43 4- Addition Of Halogens: Addition of halogens (Cl2, Br2) to benzene was carried out in presence of sun light and absence of the catalyst. Cl Cl Cl 3 Cl2 hv Cl Cl Cl gamexan (hexachloro benzene) Reactions of Aryl halides 1- Formation of Grignard reagents: Aryl halides react with Mg in presence of water free ether as a solvent to give aryl magnesium halides where the reactivity of halogen in this reaction is in the following order: I2 > Br2 > Cl2 dry ether I + Mg MgI 2- Ullmann reaction: When aryl halides are heated with activated cupper powder, resulted in coupling of the two aromatic nucleuses. 2 Cu Heat 2 CuI 2 I + + Biphenyl 3- Electrophilic aromatic substitution: The aromatic ring can undergo the typical electrophilic substitutions. e.g. nitration: 44 NO2 conc. HNO3 Cl Cl + O2N Cl conc. H2SO4 o-Nitrochlorobenzene p-Nitrochlorobenzene 4- Reaction with nucleophiles (Nucleophilic Aromatic Substitution): Aryl halides are characterized by very low reactivity toward the nucleophilic reagents like OH-, OR-, NH2 and CN-. This low of reactivity is due to delocalization of the unshared electrons on the halogen atom by resonances. Cl Cl Cl Cl Cl This means that the C-Cl bond has certain double bond character (i.e. this bond become difficult to be broken and difficult to replace by nucleophiles compared with the C-Cl bond in alkyl halides). Cl + Nu No reaction Aryl halides can undergo nucleophilic aromatic substitution reactions by activation [heat (or/and) in presence of electron-withdrawing groups like (- NO2, -NO, -SO3H, -CHO, -COR or –CN) in the aromatic ring]. The following examples illustrate the effect of such groups on the reactivity: 45 a- Replacement of hydroxyl group: NaOH (aq.) Cl OH 300oC, pressure Chlorobenzene Phenol aq. NaOH O2N Cl O2N OH 160oC p-Nitrochlorobenzene p-Nitrophenol Cl OH aq. Na2CO3 NO2 NO2 130oC O 2N O2 N 2,4-Dinitrochlorobenzene 2,4-Dinitrophenol O 2N O2N H2 O Cl NO2 HO NO2 warm O2 N O2 N 2,4,6-Trinitrochlorobenzene 2,4,6-Trinitrophenol b- Reaction with cyanide: NaCN + CuCN Hydrolysis Cl CN COOH 200oC, pressure H+, H2O C- Reaction with ammonia: 2NH3 , CuO 2 Cl 2 NH2 + CuCl2 + H2O 200oC, pressure 46 4- Formation of DDT: Heating of chlorobenzene with chloral, in the presence of concentrated sulphuric acid resulted in formation DDT. DDT possesses a strong toxicity towards various insects and widely used as pest-controller in agriculture. Cl conc. H2SO4 2 Cl + CCl3CHO CCl3CH - H2O Cl Chlorobenzene Chloral DDT b- Aralkyl Halides These are compounds in which the halogen atom is not directly attached to the ring but to a side chain, e.g. benzyl chloride, C6H5CH2Cl. Methods of preparation 1- Halogenation: Chlorination and bromination favour the high temperature and light. Chlorination of toluene is carried out stepwise to give mono, di, and trichloro derivatives. CH3 CH2Cl CHCl2 CCl3 Cl2 Cl2 Cl2 hv hv hv Toluene benzyl chloride benzal chloride benzo trichloride 2- Chloromethylation of benzene: Benzene reacts with formaldehyde and HCl in the presence of ZnCl2 as Lewis acid catalyst in methylene chloride as solvent. 47 ZnCl2 + CH2O / HCl CH2Cl Mechanism: H2C O H H2 C O H H2C OH H2C OH HCl Ar H Ar CH2 OH Ar CH2 Cl + H2O ZnCl2 Chemical Reactions The halogen in the side-chain undergoes the same reactions as those of aliphatic alkyl halides. For example, benzyl chloride on heating with dilute alkali yields benzyl alcohol, with potassium cyanide, benzyl cyanide, and with ammonia, benzylamine. aq. NaOH CH2OH CH2Cl Benzylalcohol KCN H2O CH2CN CH2COOH Benzylcyanide Phenylacetic acid alc. NH3 CH2NH2 Benzylamine 48 Aromatic Sulphonic Acids The direct displacement of one or more hydrogen atoms by a sulpho group -SO3H, by means of conc. Sulphuric acid or oleum, is particularly characteristic of aromatic hydrocarbons and their derivatives. Physical Properties: Aromatic sulphonic acids are colorless, crystalline, highly dissolve in water as completely dissociated in water. Because of the difficulty of isolating anhydrous sulphonic acids, they are usually salted out as sodium salt by the addition of a saturated salt solution. SO3H SO3Na + NaCl + HCl Benzenesulfonic acid Sod.benzenesulfonate SO3H SO3H SO3H CH3 SO3H benzenesulfonic acid benzene-1,3-disulfonic acid o-toluenesulfonic acid SO3H SO3H SO3H Cl CH3 NH2 p-toluenesulfonic acid 2-chlorobenzenesulfonic acid 4-aminobenzenesulfonic acid CH3 CH3 SO2Cl SO2NH2 p-toluenesulfonyl chloride p-toluenesulfonamide 49 Methods of preparation 1- Direct sulphonation: SO3H H2SO4 SO3 + + H2O 30-50oC CH3 CH3 H2SO4 SO3 + + H2O o 110-120 C SO3H 2- Effect of Chloro sulphonic acid: SO3H + Cl SO3H CCl4 + HCl 3- Effect of sulphoryl chloride: SO2Cl SO3H pyridine H2O + SO2Cl2 + HCl - HCl Reactions of Sulphonic acids The sulphonic group is a good leaving group so it can be replaced by another atom or group in nucleophilic displacement reactions. 51 1- Replacement of the sulphonic group by Hydrogen (Desulphonation): This can be carried out by heating benzene sulphonic acid with dilute HCl or H2SO4, It gives benzene. SO3H H2SO4 H2SO4 + H2O + 150-200oC 2- Salt formation: They form salts (benzene sulphonate) when they are reacted with NaOH solution. SO3H SO3 Na + NaOH + H2O The strong acidic character of sulphonic acids is due to formation of the stable sulphonate ion which stabilized by resonance. O O S O H S O + H O O The salt is very important which can be used in the preparation of some organic compounds, for example: a- Conversion into amines: SO3Na NH2 + NaNH2 fussion + Na2SO3 Sodamide Aniline 51 b- Conversion into carboxylic acids: SO3Na COOH + HCOONa + Na2SO3 Sodium formate c- Conversion into Phenols: by fusion with NaOH. SO3Na ONa OH fusion H2SO4 + NaOH - Na2SO3 d- Conversion into Cyanides: By fusion with sodium cyanide, then with the acidic hydrolysis gives the corresponding acid SO3Na C N COOH fusion H2O + NaC N - Na2SO3 HCl e- Conversion into sulphonyl chlorides: SO2 OH SO2Cl + PCl5 + POCl3 + HCl phosphorous benzene sulphonyl chloride penta chloride f- Conversion into esters: SO2 OH SO2 OC2H5 NaOH H2O + HO C2H5 + 52 Ethyl benzenesulphonate Preparation of m-toluene sulphonic acid: CH3 CH3 CH3 NaNO2 2H HCl SO3H 0oC SO3H SO3H NH2 N2Cl 2-amino-5-methylbenzenesulfonic acid Preparation of sulphanilic acid: NH2 NH3 HSO4 NH SO3H NH2 conc. - H2 O rearrange H2SO4 Aniline SO3H sulphanilic acid Preparation of Saccharin: CH3 CH3 CH3 SO3H SO2Cl NH3 SO2NH2 PCl5 o-Toluenesulphonic acid COOH CO SO2NH2 (O) heat NH KMnO4 - H2 O SO2 Saccharin Sulphonamides Ammonia, primary and secondary amines react with aryl sulphonyl chlorides to form sulphonamides. 53 Ar SO2Cl + 2 NH3 Ar SO2NH2 + NH4Cl H H Ar SO2Cl + H N R Ar SO2 N R R R Ar SO2Cl + H N R Ar SO2 N R Sulphonamides have medical value as antibacterial agents, e.g. 4-aminobenzenesulphonamide (sulphanilamide) H2N SO2NH2 Sulpha drugs (Sulphanilamide) The general structure of the sulpha drugs has the formula: H2N SO2NH R Where R is often a heterocyclic group Variation of the chemical structure related to sulphanilamide was made, among the successful variations were the compounds shown below: Sulphapyridine Sulphadiazine N N H2N SO2NH H2N SO2NH N Sulphathiazole Sulphacetamide N H2N SO2NH H2N SO2NHCOCH3 S 54 Preparation of sulphanilamide: NH2 NHCOCH3 NHCOCH3 NHCOCH3 NH2 (CH3CO)2O ClSO2OH NH3 H2O -H2O H+ SO2Cl SO2NH2 SO2NH2 Aniline Acetanilide Sulphanilamide General method for the preparation of Sulpha drugs: NH2 NHCOCH3 NHCOCH3 NHCOCH3 NH2 (CH3CO)2O ClSO2OH R NH2 H2O -H2O H+ SO2Cl SO2NHR SO2NHR 55 Aromatic Nitro-Compounds Aromatic nitro-compounds have big importance, particularly as raw materials in the dyestuff and explosive industry, as well for the synthesis of pharmaceuticals. This partly is due to the ease with which aromatic hydrocarbons undergo nitration. These compounds contain one or more nitro group, which represented as follow: O O N N O O Nomenclature: NO2 NO2 NO2 O2N NO2 NO2 Nitrobenzene 1,3-Dinitrobenzene 1,3,5-Trinitrobenzene CH3 CH3 CH3 NO2 O2 N NO2 NO2 NO2 2-Nitrotoluene 4-Nitrotoluene 2,4,6-Trinitrotoluene Preparations: 1- From direct nitration: Nitration was carried out by gentle heating of the aromatic hydrocarbon like benzene with a mixture of conc. nitric acid and conc. sulphuric acid. Other nitrating agents are: fuming nitric acid, nitronuim borofluoride 56 (NO2+BF4-), and acetyl nitrate (CH3COONO2). Mono nitro benzene can be prepared by heating the nitrating mixture (conc. HNO3/H2SO4) at 60oC, and to introduce second nitro group we must use fuming HNO3 and rising the temperature to 90 oC. NO2 NO2 conc. HNO3 fuming HNO3 conc. H2SO4 conc. H2SO4 60oC 90oC NO2 Nitrobenzene 1,3-dinitrobenzene The direct introduction of a third nitro group into the benzene ring is extremely difficult, because the two NO2 groups are deactivating groups which decrease the electron density at the benzene ring. The presence of an activating group facilate the third substitution. For example; Preparation of 1,3,5-trinitrobenzene CH3 CH3 CH3 CH3 NO2 NO2 conc. HNO3 Nitration + conc. H2SO4 NO2 NO2 2,4-Dinitrotoluene CH3 O2 N NO2 Nitration NO2 2,4,6-Trinitrotoluene (TNT) 57 Trinitrobenzene (TNB): It can be prepared by oxidation then decarboxylation of 2,4,6- trinitrotoluene (TNT): CH3 COOH O2N NO2 O2 N NO2 O2 N NO2 Na2Cr2O7 NaOH/CaO H2SO4 heat NO2 NO2 NO2 2,4,6-Trinitrotoluene 2,4,6-Trinitrobenzoic acid 1,3,5-Trinitrobenzene (TNT) (TNB) 2- From diazonium salts: N2Cl NO2 CuNO2 + NaNO2 CH3 CH3 m-Nitrotoluene: It can be prepared from p-nitrotoluene by indirect method: CH3 CH3 CH3 CH3 Sn / HCl (CH3CO)2O HNO3 Reduction NO2 NO2 NH2 NHCOCH3 NHCOCH3 p-Nitrotoluene CH3 CH3 CH3 C2H5OH HNO2 HCl H2O heat 0oC NO2 NO2 NO2 N2Cl NH2 m-Nitrotoluene 58 Chemical Reactions 1- Reduction Reactions: Aromatic nitro-compounds are reduced to primary amines in mineral acid solutions. The reduction of nitrobenzene to aniline proceeds through a number of stages: NO2 N O NHOH NH2 Nitrosobenzene N-hydroxybenzenamine Aniline The reduction reaction is dependent on the pH of the solution. a) Reduction to aniline (in strong acidic solution): By the reduction of nitrobenzene with iron and hydrochloric acid 4 Ph NO2 + 8 Fe + 4 H2O (HCl) 4 PhNH2 + 4 Fe2O3 b) Reduction to N-phenylhydroxylamine (in neutral or weakly acidic solution): N-phenylhydroxylamine, represents the lowest reduction stage of nitrobenzene which has been isolated. Ph NO2 + 2 Zn + 4 NH4Cl (aq.) Ph NHOH + 2 ZnCl2 + H2O Although nitrosobenzene was regarded as the primary reduction product of nitrobenzene, it cannot be isolated as it immediately undergoes further reduction. It can be prepared from the oxidation of N- phenylhydroxylamine; Na2Cr2O7 3 Ph NHOH 3 Ph N O + Na2SO4 + Cr2(SO4)3 + 7 H2O 4 H2SO4 59 c- Reduction to azoxybenzene (in alkaline solution): By heating of nitrobenzene with methanolic potassium hydroxide 4 Ph NO2 + 3 CH3OK 2 Ph N N Ph + 3 H2O + 3 HCOOk O nitrobenzene azoxybenzene Preparation of Azobenzene: 1- From Nitrobenzene: Reduction of nitrobenzene with sodium amalgam or lithium aluminium hydride: LiAlH4 2 Ph NO2 + 8H Ph N N Ph + 4 H2O Azobenzene 2- From azoxybenzene: Reduction of azoxybenzene by heating with iron filings gives azobenzene. 3 Ph N N Ph + 2 Fe Ph N N Ph + Fe2O3 O 3- From hydrazobenzene (Ph-NHNHPh): Via oxidation with air or NaOBr H H Ph N N Ph + NaOBr Ph N N Ph + NaBr + H2O 61 Preparation of Hydrazobenzene It is the end product of alkaline reduction of nitrobenzene, and it can be formed by the reduction with zinc dust and aqueous sodium hydroxide: H H 2 Ph NO2 + 5 Zn + 10 NaOH Ph N N Ph + 5 Na2ZnO2 + 4 H2O Hydrazobenzene On heating hydrazobenzene, converted to azobenzene and aniline: H H 2 Ph N N Ph Ph N N Ph + 2 Ph NH2 61 Aromatic Amines Aromatic amines contain an amino group (-NH2) or substituted amino group linked directly to the benzene nucleus. As in aliphatic series, aromatic amines are divided into primary, secondary and tertiary amines and subdivided into mixed aliphatic/aromatic and purely aromatic amines: Amine General formula Example Primary Amines Ar-NH2 Ph-NH2 (Aniline) Secondary Amines Ar-NHR Ph-NH-CH3 (N-Methylaniline) (Ar)2NH Ph-NH-Ph (Diphenylamine) Tertiary Amines Ar-NR2 Ph-N(CH3)2 (N,N-Dimethyl aniline) Ar3N (Ph)3N (Triphenylaniline) There are three isomeric amines derived from toluene: o-Toluidine m-Toluidine p-Toluidine CH3 CH3 CH3 NH2 NH2 NH2 62 Preparation of Primary Amines 1- From nitro-compounds: via reduction of nitro-compounds NO2 NH2 Sn/HCl + 2H2O 6H CH3 CH3 NO2 NH2 Sn/HCl + 2H2O 6H 2- From Aryl halides: via amination of aryl halides Cl NH2 Cu2O + NH3 o + HCl 200 C, presssure In presence of nitro group in the orth-o and para- positions, the amination becomes much more easier in the ordinary conditions. Cl NH2 O2N NO2 O2 N NO2 + NH3 + HCl O2N O2 N 3- From Action of ammonia on Phenols: OH NH2 ZnCl2 + NH3 + H2O 300oC, pressure 63 4- From sulphonic acid: Fusion Ph SO3Na + NaNH2 Ph NH2 + Na2SO3 sod. benzene sulphonate sodamide Aniline 5- From amides via Hofmann degradation: Amides react with solutions of bromine or chlorine in sodium hydroxide to yield amines. CONH2 NH2 Br2 NaOH Benzamide Mechanism: O H O Br OH Ar C N H2O + Br + Ar C N Br Br H H OH O H2O Na2CO3 + H2N Ar O C N Ar Ar C N Br OH 6- From acyl azides via Curtius rearrangement: Aroyl azides, may be obtained from the reaction of sodium azide with acid chlorides, which decompose on heating to the aryl isocyanates. The isocyanates on hydrolysis give aromatic amines. Acid chlorides are prepared from carboxylic acids by the action of thionyl chloride. 64 O Ar COCl + NaN3 Ar C N N N Acid chloride sod. azide Aroyl azides Ar N C O HOH Ar NH2 + CO2 Ar N N N C - N2 Aryl isocyanates O SOCl2 SO2 HCl Ar COOH Ar COCl + + Preparation of Secondary and Tertiary Amines 1) N-Alkylated anilines: a- Via alkylation of aniline by alkyl halides: NH2 HN C2H5 NaOH + C2H5Br + HBr Aniline Ethyl bromide N-ethylaniline 1o amine 2o amine HN C2H5 C2H5 N C2H5 C2H5Br NaOH + + HBr N,N-Diethylaniline 3o amine b- Reduction of Anilides: NH COCH3 NH CH2CH3 LiAlH4 65 Acetanilide N-ethylaniline H3C N COCH3 H3C N CH2CH3 LiAlH4 N-methylacetanilide N-ethyl-N-methylaniline 2) N-Arylated anilines: Diphenylamine is obtained by heating aniline with aniline hydrochloride to 200 oC (Phenylation). 200 oC H Ph NH2 + Ph NH3Cl Ph N Ph + NH4Cl Aniline aniline hydrochloride Diphenyl amine Triphenylamine is obtained by Ullmann reaction in which diphenylamine and iodobenzene are heated together with potassium carbonate and a little amount of cupper. K2CO3 (Ph)2NH + Ph I (Ph)3N + KI + KHCO3 Cu Diphenyl amine Iodobenzene Triphenylamine Chemical Reactions The reactivity of amines arises from the ability of the nitrogen atom to share an electron pair. A- Amine acting as a base: N + H N H 66 B- Amine acting as a nucleophile in alkylation reactions: N + R CH2 Br N CH2R + Br C- Amine acting as a nucleophile in acylation reactions: N + R C Cl N C R + HCl O O 1- Basicity: Comparing the basicity of amines could be measured by the extent to which they accept hydrogen proton from water, the equilibrium constant is called the basicity constant (Kb). The smaller the numerical value of pKb , the stronger the base to which refers. Aromatic amines are very weak bases compared with ammonia or aliphatic amines. RNH2 + H2O RNH3 + OH [RNH3+] [OH-] Kb = [RNH2] pKb = - log Kb Base PhNH2 NH3 CH3NH2 pKb 9.38 4.75 3.46 This was explained on the basis of resonance stabilization afforded by the interaction of the unshared electron pair on the aniline nitrogen atom with the delocalized π–orbitals of the nucleus. 67 NH2 NH2 NH2 NH2 NH2 Salt formation: Due to the weak basicity of aniline, it forms salts with only strong acids. PhNH2 + HCl [PhNH3]+Cl- (Aniline hydrochloride) PhNH2 + H2SO4 [PhNH3]+HSO4- (Aniline sulphate) 2- Alkylation: Aromatic amines react with alkyl halides, to give secondary and tert. aromatic amines. CH3 NH2 HN CH3 H3C N CH3 H3C N CH3 I CH3I CH3I CH3I - HI HI (N-Methylaniline) N,N-Dimethylaniline) (Trimethylanilinium iodide) (sec. amine) (t. amine) (quaternary salt) 3- Acylation: Aromatic amines can be acylated by acid chlorides and anhydrides, to give the anilides. HN COC6H5 NH2 HN COCH3 C6H5COCl CH3COCl (Benzamide) (Acetanilide) 68 Mechanism: O H O R C + H2N Ar R C N Ar Cl Cl H Cl O O H H -H R C N Ar R C N Ar H The reaction of amines with sulphonyl chlorides leads to the formation of the corresponding sulphoanilides. C6H5SO2Cl NaOH NH2 + NHSO2C6H5 - HCl (Benzenesulphoanilide) 4- Reaction with isocyanates: H O O H H PhNH2 + Ph N C O Ph N C N Ph Ph N C N Ph (phenyl isocyanate) H (Diphenylurea) 5- Reaction with nitrous acid: a- With Primary amine: Primary aromatic amines reacted with nitrous acid at 0-5oC to yield diazonium salt. NaNO2/HCl Ar N N Cl Ar NH2 + NaCl + 2H2O 0-5 oC 69 Mechanism: H - H2O Ar N N O Ar NH2 + HO N O (N-Nitrosoaniline) fast +HCl fast Ar N N Cl Ar N N OH Ar N N OH -H2O (Diazonium chloride) (Diazonium hydroxide) b- With Secondary amines: Secondary amines react with nitrous acid to yield N-nitrosoamine. H NO NaNO2/HCl N